Biology:Electric organ
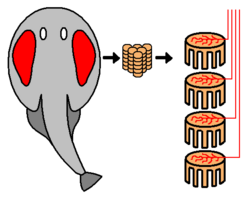
In biology, the electric organ is an organ common to all electric fish used for the purposes of creating an electric field. The electric organ is derived from modified nerve or muscle tissue.[1] The electric discharge from this organ is used for navigation, communication, mating, defense and also sometimes for the incapacitation of prey.[2][3]
Research history
In the 1770s the electric organs of the torpedo and electric eel were the subject of Royal Society papers by Hunter, Walsh and Williamson. They appear to have influenced the thinking of Luigi Galvani and Alessandro Volta - the founders of electrophysiology and electrochemistry.[4]
In the 19th century, Charles Darwin discussed the electric organ in his Origin of Species as a likely example of convergent evolution: "But if the electric organs had been inherited from one ancient progenitor thus provided, we might have expected that all electric fishes would have been specially related to each other…I am inclined to believe that in nearly the same way as two men have sometimes independently hit on the very same invention, so natural selection, working for the good of each being and taking advantage of analogous variations, has sometimes modified in very nearly the same manner two parts in two organic beings".[5]
Since the 20th Century, electric organs have received extensive study, for example Hans Lissmann's pioneering 1951 paper[6] and his review of their function and evolution in 1958.[7] More recently, Torpedo californica electrocytes were used in the first sequencing of the acetylcholine receptor by Noda and colleagues in 1982, while Electrophorus electrocytes served in the first sequencing of the voltage-gated sodium channel by Noda and colleagues in 1984.[8]
Evolution
Electric organs have evolved at least six times in various teleost and elasmobranch fish.[9][10][11][12] Notably, they have convergently evolved in the African Mormyridae and South American Gymnotidae groups of electric fish. The two groups are distantly related, as they shared a common ancestor before the supercontinent Gondwana split into the American and African continents, leading to the divergence of the two groups. A whole-genome duplication event in the teleost lineage allowed for the neofunctionalization of the voltage-gated sodium channel gene Scn4aa which produces electric discharges.[13][14]
Although previous research pointed to the convergence of the exact genetic development of the same genes and developmental and cellular pathways to make an electric organ in the different lineages, more recent genomic research has proven more nuanced. [15] Comparative transcriptomics of the Mormyroidea, Siluriformes, and Gymnotiformes lineages conducted by Liu (2019) concluded that although there is no parallel evolution of the entire transcriptomes of electric organs among different lineages, there are a significant number of genes that exhibit parallel gene expression changes at the level of pathways and biological functions. Even though electric organs from these diverse lineages may have resulted from different genetic alterations, the genes that changed expression during the evolution from skeletal muscle to discharge organs were likely genes with similar functions within their respective organism. These results solidify the hypothesis that it is not different genes but conserved biological functions that play a crucial role in the convergence of this particular complex phenotype. [16] Despite different genes being involved in the development process of the electric organ, the ultimate result was obtained via similar wholescale pathways and biological functions.
The electrocytes are derived from skeletal muscle in all clades except Apteronotus (Latin America), where the cells are derived from neural tissue.[8]
The original function of the electric organ has not been fully established, although there has been promising research regarding the African freshwater catfish genus Synodontis. [17] This research illustrates that the simple myogenic electric organs of Synodontis were derived from muscles that previously performed a sound-generation function.
Electrocytes
Electrocytes, electroplaques or electroplaxes are cells used by electric eels, rays, and other fish for electrogenesis.[8] In some species they are cigar-shaped; in others, they are flat disk-like cells.[8] Electric eels have several thousand of these cells stacked, each producing 0.15 V. The cells function by pumping positive sodium and potassium ions out of the cell via transport proteins powered by adenosine triphosphate (ATP). Postsynaptically, electrocytes work much like muscle cells. They have nicotinic acetylcholine receptors. Despite the shared origin of skeletal muscle cells and electrocytes in myogenic electric organs, electric organs and skeletal muscle remain distinct in both morphology and physiology. Some key ways in which these cells differ include size (electrocytes are much larger) and the lack of any contractible machinery on the part of electrocytes.
The stack of electrocytes has long been compared to a voltaic pile, and may even have inspired the invention of the battery, since the analogy was already noted by Alessandro Volta.[4] While the electric organ is structurally similar to a battery, its cycle of operation is more like a Marx generator, in that the individual elements are slowly charged in parallel, then suddenly and nearly simultaneously discharged in series to produce a high voltage pulse.
Firing
To discharge the electrocytes at the correct time, the electric eel uses its pacemaker nucleus, a nucleus of pacemaker neurons. When an electric eel spots its prey, the pacemaker neurons fire and acetylcholine is subsequently released from electromotor neurons to the electrocytes. The electrocytes fire an action potential using the voltage-gated sodium channels on one or both sides of the electrocyte, depending on the complexity of the electric organ in that species. If the electrocyte has sodium channels on both sides, the depolarization caused by firing action potentials on one side of the electrocyte can cause the sodium channels on the other side of the electrocyte to fire as well.[18]
Location
In most fishes, electric organs are oriented to fire along the length of the body, usually lying along the length of the tail and within the fish's musculature, with smaller accessory electric organs in the head. However, there are some exceptions; in stargazers and in rays the electric organs are oriented along the dorso-ventral (up-down) axis. In the electric torpedo ray, the organ is near the pectoral muscles and the gills (see the image). The stargazer's electric organs lie between the mouth and the eye. In the electric catfish, the organs are located just below the skin and encase most of the body like a sheath.
Electric organ discharge
Electric organ discharge is the electric field generated by the organs of animals including electric fish. In some cases the electric discharge is strong and is used for protection from predators; in other cases it is weak and it is used for navigation and communication.[19] Weakly electric fish' electric organ discharges can be broadly categorized as either wave-type or pulse-type discharges. Wave-type discharges are periodic quasi-sinusoidal, while pulse-type discharges are highly variable in their duration with longer pause intervals.[20] Communicating through electric organ discharges occurs when a fish uses its own electroreceptors to sense the electric signals of a nearby fish.[21] Electric fish navigate by detecting distortions in their electrical field by using their cutaneous electroreceptors.[22][23][24] Electric organ discharges influence mate choice in weakly electric fish, as females have been shown to be attracted to electric discharge characteristics of conspecific males.[3]
See also
- Biology in fiction#Physiology
References
- ↑ Kramer, Bernd (1996). "Electroreception and communication in fishes". Progress in Zoology 42. http://epub.uni-regensburg.de/2108/1/ubr00728.pdf.
- ↑ Castello, M. E., A. Rodriguez-Cattaneo, P. A. Aguilera, L. Iribarne, A. C. Pereira, and A. A. Caputi (2009). "Waveform generation in the weakly electric fish Gymnotus coropinae (Hoedeman): the electric organ and the electric organ discharge". Journal of Experimental Biology 212 (9): 1351–1364. doi:10.1242/jeb.022566. PMID 19376956.
- ↑ 3.0 3.1 Feulner, P. G., M. Plath, J. Engelmann, F. Kirschbaum, R. Tiedemann (2009). "Electrifying love: electric fish use species-specific discharge for mate recognition". Biology Letters 5 (2): 225–228. doi:10.1098/rsbl.2008.0566. PMID 19033131.
- ↑ 4.0 4.1 Mauro Alexander (1969). "The role of the voltaic pile in the Galvani-Volta controversy concerning animal vs. metallic electricity". Journal of the History of Medicine and Allied Sciences XXIV (2): 140–150. doi:10.1093/jhmas/xxiv.2.140. PMID 4895861.
- ↑ Darwin, Charles (1859). On the origin of species by means of natural selection, or the preservation of favoured races in the struggle for life.. London: John Murray. ISBN 978-1-4353-9386-8.
- ↑ Lissmann, Hans W. (1951). "Continuous Electrical Signals from the Tail of a Fish, Gymnarchus niloticus Cuv". Nature 167 (4240): 201–202. doi:10.1038/167201a0. PMID 14806425. Bibcode: 1951Natur.167..201L.
- ↑ Lissmann, Hans W. (1958). "ON THE FUNCTION AND EVOLUTION OF ELECTRIC ORGANS IN FISH". Journal of Experimental Biology 35: 156ff. doi:10.1242/jeb.35.1.156.
- ↑ 8.0 8.1 8.2 8.3 Markham, M. R. (2013). "Electrocyte physiology: 50 years later". Journal of Experimental Biology 216 (13): 2451–2458. doi:10.1242/jeb.082628. ISSN 0022-0949. PMID 23761470.
- ↑ Zakon, H. H., D. J. Zwickl, Y. Lu, and D. M. Hillis (2008). "Molecular evolution of communication signals in electric fish". Journal of Experimental Biology 211 (11): 1814–1818. doi:10.1242/jeb.015982. PMID 18490397.
- ↑ Lavoue, S., R. Bigorne, G. Lecointre, and J. F. Agnese (2000). "Phylogenetic relationships of mormyrid electric fishes (Mormyridae; Teleostei) inferred from cytochrome b sequences". Molecular Phylogenetics and Evolution 14 (1): 1–10. doi:10.1006/mpev.1999.0687. PMID 10631038.
- ↑ Lavoué S., Miya M., Arnegard M. E., Sullivan J. P., Hopkins C. D., Nishida M. (2012). "Comparable ages for the independent origins of electrogenesis in African and South American weakly electric fishes". PLOS ONE 7 (5): e36287. doi:10.1371/journal.pone.0036287. PMID 22606250. Bibcode: 2012PLoSO...736287L.
- ↑ Kawasaki, M. (2009). "Evolution of Time-Coding Systems in Weakly Electric Fishes". Zoological Science 26 (9): 587–599. doi:10.2108/zsj.26.587. PMID 19799509.
- ↑ Gallant, J. R., L. L. Traeger, J. D. Volkening, H. Moffett, P. H. Chen, C. D. Novina, G. N. Phillips (2014). "Genomic basis for the convergent evolution of electric organs". Science 344 (6191): 1522–1525. doi:10.1126/science.1254432. PMID 24970089. Bibcode: 2014Sci...344.1522G.
- ↑ Arnegard, M. E., D. J. Zwickl, Y. Lu, H. H. Zakon (2010). "Old gene duplication facilitates origin and diversification of an innovative communication system-twice". Proceedings of the National Academy of Sciences 107 (51): 22172–22177. doi:10.1073/pnas.1011803107. PMID 21127261.
- ↑ Liu, A., F. He, J. Zhou, Y. Zou, Z. Su, and X. Gu. (2019). "Comparative Transcriptome Analyses Reveal the Role of Conserved Function in Electric Organ Convergence Across Electric Fishes". Frontiers in Genetics 10: 664. doi:10.3389/fgene.2019.00664. PMID 31379927.
- ↑ Zhou, X., I. Seim, and V. N. Gladyshev. (2015). "Convergent evolution of marine mammals is associated with distinct substitutions in common genes". Scientific Reports 5: 16550. doi:10.1038/srep16550. PMID 26549748. Bibcode: 2015NatSR...516550Z.
- ↑ Boyle, K. S., O. Colleye, and E. Parmentier. (2014). "Sound production to electric discharge: sonic muscle evolution in progress in Synodontis spp. catfishes (Mochokidae)". Proceedings of the Royal Society B: Biological Sciences 281 (1791): 20141197. doi:10.1098/rspb.2014.1197. PMID 25080341.
- ↑ Salazar, V. L., R. Krahe, J. E. Lewis (2013). "The energetics of electric organ discharge generation in gymnotiform weakly electric fish". Journal of Experimental Biology 216 (13): 2459–2468. doi:10.1242/jeb.082735. PMID 23761471.
- ↑ Caputi, A. A., B. A. Carlson, and O. Macadar. 2005. Electric organs and their control. Pages 410-451 in T. H. Bullock, C. D. Hopkins, A. N. Popper, and R. R. Fay, eds. Electroreception. New York.
- ↑ Krahe, Rüdiger (2019), Carlson, Bruce A.; Sisneros, Joseph A.; Popper, Arthur N. et al., eds., "Evolutionary Drivers of Electric Signal Diversity" (in en), Electroreception: Fundamental Insights from Comparative Approaches, Springer Handbook of Auditory Research (Cham: Springer International Publishing) 70: pp. 191–226, doi:10.1007/978-3-030-29105-1_7, ISBN 978-3-030-29105-1, https://doi.org/10.1007/978-3-030-29105-1_7, retrieved 2020-11-15
- ↑ Crampton W. G. R., Davis J. K., Lovejoy N. R., Pensky M. (2008). "Multivariate classification of animal communication signals: A simulation-based comparison of alternative signal processing procedures using electric fishes". Journal of Physiology-Paris 102 (4–6): 304–321. doi:10.1016/j.jphysparis.2008.10.001. PMID 18984042.
- ↑ Bastian J. 1986. Electrolocation: behavior, anatomy, and physiology. Pages 577-612 in T. H. Bullock and W. Heiligenberg, eds. Electroreception. New York.
- ↑ Aguilera P. A., Caputi A. A. (2003). "Electroreception in G. carapo: detection of changes in waveform of the electrosensory signals". Journal of Experimental Biology 206 (6): 989–998. doi:10.1242/jeb.00198. PMID 12582141.
- ↑ Pereira, A. C.; A. A. Caputi (2010). "Imaging in Electrosensory Systems". Interdisciplinary Sciences-Computational Life Sciences 2 (4): 291–307. doi:10.1007/s12539-010-0049-2. PMID 21153776.