Earth:Marine ice sheet instability
![]() | It has been suggested that this page be merged into Ice-sheet dynamics. (Discuss) Proposed since January 2024. |
File:West Antarctic Collapse.ogv Marine ice sheet instability (MISI) describes the potential for ice sheets grounded below sea level to destabilize in a runaway fashion. The mechanism was first proposed in the 1970s[1][2] by Johannes Weertman and was quickly identified as a means by which even gradual anthropogenic warming could lead to relatively rapid sea level rise.[3][4] In Antarctica, the West Antarctic Ice Sheet, the Aurora Subglacial Basin, and the Wilkes Basin are each grounded below sea level and are inherently subject to MISI.
General
The term marine ice sheet describes an ice sheet whose base rests on ground below sea level, and marine ice sheet instability describes the inherent precarious nature of marine ice sheets due to Archimedes' principle. Because seawater is denser than ice, marine ice sheets can only remain stable where the ice is thick enough for its mass to exceed the mass of the seawater displaced by the ice. In other words, wherever ice exists below sea level, it is held in place only by the weight of overlying ice. As a marine ice sheet melts, the weight of the overlying ice decreases. If melt causes thinning beyond a critical threshold, the overlying ice may no longer be heavy enough to prevent the submarine ice below it from lifting off the ground, allowing water to penetrate underneath.
The location of the grounding line, the boundary between the ice sheet and the floating ice shelves, is unstable in this case. The amount of ice flowing over the grounding line initially matches the production of ice from snow upstream. When the grounding line is pushed backwards, due to for instance melt by warm water, the ice sheet is thicker at the new location of the grounding line and the total amount of ice flowing through may increase. (This depends on the slope of the subaerial surface.) As this causes the ice sheet to lose mass, the grounding line is pushed back even further and this self-reinforcing mechanism is the cause of the instability. Ice sheets of this type have accelerated ice sheet retreat.[5][6]
Strictly speaking the MISI theory is only valid if the ice shelves are free floating and not constrained in an embayment.[7]
The initial perturbation or push-back of the grounding line might be caused by high water temperatures at the base of ice shelves so that melt increases (basal melt). The thinned ice shelves, which earlier stabilized the ice sheet, exert less of an buttressing effect (back stress).[5]
Marine Ice Cliff Instability
A related process known as Marine Ice Cliff Instability (MICI) posits that due to the physical characteristics of ice, subaerial ice cliffs exceeding ~90 meters in height are likely to collapse under their own weight, and could lead to runaway ice sheet retreat in a fashion similar to MISI.[5] For an ice sheet grounded below sea level with an inland-sloping bed, ice cliff failure removes peripheral ice, which then exposes taller, more unstable ice cliffs, further perpetuating the cycle of ice front failure and retreat. Surface melt can further enhance MICI through ponding and hydrofracture.[7][8]
Ocean warming
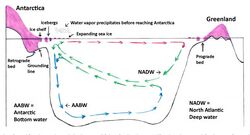
According to a 2016 published study, cold meltwater provides cooling of the ocean's surface layer, acting like a lid, and also affecting deeper waters by increasing subsurface ocean warming and thus facilitating ice melt.
Our "pure freshwater" experiments show that the low-density lid causes deep-ocean warming, especially at depths of ice shelf grounding lines that provide most of the restraining force limiting ice sheet discharge.[9]
Another theory discussed in 2007 for increasing warm bottom water is that changes in air circulation patterns have led to increased upwelling of warm, deep ocean water along the coast of Antarctica and that this warm water has increased melting of floating ice shelves.[10] An ocean model has shown how changes in winds can help channel the water along deep troughs on the sea floor, toward the ice shelves of outlet glaciers.[11]
Observations
In West Antarctica, the Thwaites and Pine Island glaciers have been identified to be potentially prone to MISI, and both glaciers have been rapidly thinning and accelerating in recent decades.[12][13][14][15] In East Antarctica, Totten Glacier is the largest glacier known to be subject to MISI,[16] and its potential contribution to sea level rise is comparable to that of the entire West Antarctic Ice Sheet. Totten Glacier has been losing mass nearly monotonically in recent decades,[17] suggesting rapid retreat is possible in the near future, although the dynamic behavior of Totten Ice Shelf is known to vary on seasonal to interannual timescales.[18][19][20] The Wilkes Basin is the only major submarine basin in Antarctica that is not thought to be sensitive to warming.[14]
In October 2023, a study published in Nature Climate Change projected that ocean warming at about triple the historical rate is likely unavoidable in the 21st century, with no significant difference between mid-range emissions scenarios versus achieving the most ambitious targets of the Paris Agreement—suggesting that greenhouse gas mitigation has limited ability to prevent collapse of the West Antarctic Ice Sheet.[21]
See also
- Ice sheet dynamics
- Seabed gouging by ice
References
- ↑ Weertman, J. (1974). "Stability of the Junction of an Ice Sheet and an Ice Shelf" (in en). Journal of Glaciology 13 (67): 3–11. doi:10.3189/S0022143000023327. ISSN 0022-1430.
- ↑ Thomas, Robert H.; Bentley, Charles R. (1978). "A Model for Holocene Retreat of the West Antarctic Ice Sheet" (in en). Quaternary Research 10 (2): 150–170. doi:10.1016/0033-5894(78)90098-4. ISSN 0033-5894. Bibcode: 1978QuRes..10..150T.
- ↑ Mercer, J. H. (1978). "West Antarctic ice sheet and CO2 greenhouse effect: a threat of disaster" (in En). Nature 271 (5643): 321–325. doi:10.1038/271321a0. ISSN 0028-0836. Bibcode: 1978Natur.271..321M.
- ↑ Vaughan, David G. (2008-08-20). "West Antarctic Ice Sheet collapse – the fall and rise of a paradigm" (in en). Climatic Change 91 (1–2): 65–79. doi:10.1007/s10584-008-9448-3. ISSN 0165-0009. Bibcode: 2008ClCh...91...65V. http://nora.nerc.ac.uk/id/eprint/769/1/The_return_of_a_paradigm_16_-_nora.pdf.
- ↑ 5.0 5.1 5.2 David Pollard; Robert M. DeConto; Richard B. Alley (2015). "Potential Antarctic Ice Sheet retreat driven by hydrofracturing and ice cliff failure". Nature 412: 112–121. doi:10.1016/j.epsl.2014.12.035. Bibcode: 2015E&PSL.412..112P.
- ↑ David Docquier (2016). "Marine Ice Sheet Instability "For Dummies"". EGU. https://blogs.egu.eu/divisions/cr/2016/06/22/marine-ice-sheet-instability-for-dummies-2/.
- ↑ 7.0 7.1 Pattyn, Frank (2018). "The paradigm shift in Antarctic ice sheet modelling" (in En). Nature Communications 9 (1): 2728. doi:10.1038/s41467-018-05003-z. ISSN 2041-1723. PMID 30013142. Bibcode: 2018NatCo...9.2728P.
- ↑ Dow, Christine F.; Lee, Won Sang; Greenbaum, Jamin S.; Greene, Chad A.; Blankenship, Donald D.; Poinar, Kristin; Forrest, Alexander L.; Young, Duncan A. et al. (2018-06-01). "Basal channels drive active surface hydrology and transverse ice shelf fracture" (in en). Science Advances 4 (6): eaao7212. doi:10.1126/sciadv.aao7212. ISSN 2375-2548. PMID 29928691. Bibcode: 2018SciA....4.7212D.
- ↑ J. Hansen; M. Sato; P. Hearty; R. Ruedy; M. Kelley; V. Masson-Delmotte; G. Russell; G. Tselioudis et al. (2016). "Ice melt, sea level rise and superstorms: evidence from paleoclimate data, climate modeling, and modern observations that 2 °C global warming could be dangerous". Atmospheric Chemistry and Physics 16 (6): 3761–3812. doi:10.5194/acp-16-3761-2016. Bibcode: 2016ACP....16.3761H.
- ↑ "Statement: Thinning of West Antarctic Ice Sheet Demands Improved Monitoring to Reduce Uncertainty over Potential Sea-Level Rise". http://www.jsg.utexas.edu/news/2007/05/statement-thinning-of-west-antarctic-ice-sheet-demands-improved-monitoring-to-reduce-uncertainty-over-potential-sea-level-rise/.
- ↑ Thoma, M.; Jenkins, A.; Holland, D.; Jacobs, S. (2008). "Modelling Circumpolar Deep Water intrusions on the Amundsen Sea continental shelf, Antarctica". Geophysical Research Letters 35 (18): L18602. doi:10.1029/2008GL034939. Bibcode: 2008GeoRL..3518602T. http://epic.awi.de/25479/1/2008_Modelling_Circumpolar_Deep_Water_intrusions_on_the_Amundsen_Sea_continental_shelf_Antarctica.pdf.
- ↑ "After Decades of Losing Ice, Antarctica Is Now Hemorrhaging It". The Atlantic. 2018. https://www.theatlantic.com/science/archive/2018/06/after-decades-of-ice-loss-antarctica-is-now-hemorrhaging-mass/562748/.
- ↑ "Marine ice sheet instability". AntarcticGlaciers.org. 2014. http://www.antarcticglaciers.org/glaciers-and-climate/ice-ocean-interactions/marine-ice-sheets/.
- ↑ 14.0 14.1 Gardner, A. S.; Moholdt, G.; Scambos, T.; Fahnstock, M.; Ligtenberg, S.; van den Broeke, M.; Nilsson, J. (2018-02-13). "Increased West Antarctic and unchanged East Antarctic ice discharge over the last 7 years". The Cryosphere 12 (2): 521–547. doi:10.5194/tc-12-521-2018. ISSN 1994-0424. Bibcode: 2018TCry...12..521G.
- ↑ IMBIE team (2018). "Mass balance of the Antarctic Ice Sheet from 1992 to 2017" (in En). Nature 558 (7709): 219–222. doi:10.1038/s41586-018-0179-y. ISSN 0028-0836. PMID 29899482. Bibcode: 2018Natur.558..219I. https://orbi.uliege.be/handle/2268/225208.
- ↑ Young, Duncan A.; Wright, Andrew P.; Roberts, Jason L.; Warner, Roland C.; Young, Neal W.; Greenbaum, Jamin S.; Schroeder, Dustin M.; Holt, John W. et al. (2011-06-02). "A dynamic early East Antarctic Ice Sheet suggested by ice-covered fjord landscapes" (in En). Nature 474 (7349): 72–75. doi:10.1038/nature10114. ISSN 0028-0836. PMID 21637255. Bibcode: 2011Natur.474...72Y.
- ↑ Mohajerani, Yara (2018). "Mass Loss of Totten and Moscow University Glaciers, East Antarctica, Using Regionally Optimized GRACE Mascons". Geophysical Research Letters 45 (14): 7010–7018. doi:10.1029/2018GL078173. Bibcode: 2018GeoRL..45.7010M. https://escholarship.org/uc/item/21c3r9dv.
- ↑ Greene, Chad A.; Young, Duncan A.; Gwyther, David E.; Galton-Fenzi, Benjamin K.; Blankenship, Donald D. (2018). "Seasonal dynamics of Totten Ice Shelf controlled by sea ice buttressing" (in en). The Cryosphere 12 (9): 2869–2882. doi:10.5194/tc-12-2869-2018. ISSN 1994-0416. Bibcode: 2018TCry...12.2869G.
- ↑ Roberts, Jason; Galton-Fenzi, Benjamin K.; Paolo, Fernando S.; Donnelly, Claire; Gwyther, David E.; Padman, Laurie; Young, Duncan; Warner, Roland et al. (2017-08-23). "Ocean forced variability of Totten Glacier mass loss". Geological Society, London, Special Publications 461 (1): 175–186. doi:10.1144/sp461.6. ISSN 0305-8719. Bibcode: 2018GSLSP.461..175R. https://eprints.utas.edu.au/25611/1/SP461.6.full.pdf.
- ↑ Greene, Chad A.; Blankenship, Donald D.; Gwyther, David E.; Silvano, Alessandro; Wijk, Esmee van (2017-11-01). "Wind causes Totten Ice Shelf melt and acceleration" (in en). Science Advances 3 (11): e1701681. doi:10.1126/sciadv.1701681. ISSN 2375-2548. PMID 29109976. Bibcode: 2017SciA....3E1681G.
- ↑ Naughten, Kaitlin A.; Holland, Paul R.; De Rydt, Jan (23 October 2023). "Unavoidable future increase in West Antarctic ice-shelf melting over the twenty-first century". Nature Climate Change 13 (11): 1222–1228. doi:10.1038/s41558-023-01818-x. https://www.nature.com/articles/s41558-023-01818-x.
Further reading
External links
![]() | Original source: https://en.wikipedia.org/wiki/Marine ice sheet instability.
Read more |