Physics:Nicolson–Ross–Weir method
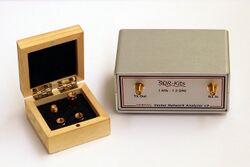
Nicolson–Ross–Weir method is a measurement technique for determination of complex permittivities and permeabilities of material samples for microwave frequencies. The method is based on insertion of a material sample with a known thickness inside a waveguide, such as a coaxial cable or a rectangular waveguide, after which the dispersion data is extracted from the resulting scattering parameters. The method is named after A. M. Nicolson and G. F. Ross,[1] and W. B. Weir,[2] who developed the approach in 1970 and 1974, respectively.
The technique is one of the most common procedures for material characterization in microwave engineering.[3]
Method
The method uses scattering parameters of a material sample embedded in a waveguide, namely [math]\displaystyle{ S_{11} }[/math] and [math]\displaystyle{ S_{21} }[/math], to calculate permittivity and permeability data. [math]\displaystyle{ S_{11} }[/math] and [math]\displaystyle{ S_{21} }[/math] correspond to the cumulative reflection and transmission coefficient of the sample that are referenced to the each sample end, respectively: these parameters account for the multiple internal reflections inside the sample, which is considered to have a thickness of [math]\displaystyle{ d }[/math]. The reflection coefficient of the bulk sample is:[1][2]
- [math]\displaystyle{ \Gamma=X \pm \sqrt{X^2-1} }[/math]
where
- [math]\displaystyle{ X=\frac{1-(S_{21}^2-S_{11}^2)}{2 S_{11}} }[/math]
The sign of the root for the reflection coefficient is chosen appropriately to ensure its passivity ([math]\displaystyle{ |\Gamma| \leq 1 }[/math]). Similarly, the transmission coefficient of the bulk sample can be written as:
- [math]\displaystyle{ T=\frac{S_{11}+S_{21}-\Gamma}{1-(S_{11}+S_{21})\Gamma} }[/math]
Thus, the effective permeability ([math]\displaystyle{ \mu^* }[/math]) and permittivity ([math]\displaystyle{ \varepsilon^* }[/math]) of the material can be written as:
- [math]\displaystyle{ \mu^*=\frac{\lambda_{0g}}{\Lambda} \left( \frac{1+\Gamma}{1-\Gamma} \right) }[/math]
- [math]\displaystyle{ \varepsilon^*=\frac{\lambda^2_0 \left( \frac{1}{\Lambda^2}+\frac{1}{\lambda_c^2} \right)}{\mu^*} }[/math]
where
- [math]\displaystyle{ \frac{1}{\Lambda^2}=-\left[ \frac{1}{2\pi d} ln\frac{1}{T} \right]^2 }[/math]
and
- [math]\displaystyle{ \lambda_0 }[/math] is the free-space wavelength.
- [math]\displaystyle{ \lambda_{0g} }[/math] is the guided mode wavelength of the unfilled transmission line.
- [math]\displaystyle{ \lambda_{c} }[/math] is the cutoff wavelength of the unfilled transmission line
The constitutive relation for [math]\displaystyle{ \Lambda }[/math] admits an infinite number of solutions due to the branches of the complex logarithm. The ambiguity regarding its result can be resolved by taking the group delay into account.[2]
Limitations and extensions
In the case of low material loss, the Nicolson–Ross–Weir method is known to be unstable for sample thicknesses at integer multiples of one half wavelength due to resonance phenomenon. Improvements over the standard algorithm have been presented in engineering literature to alleviate this effect.[4][5][6] Furthermore, complete filling of a waveguide with sample material may pose a particular challenge: presence of gaps during the filling of the waveguide section would excite higher-order modes, which may yield errors in scattering parameter results.[7] In such cases, more advanced methods based on the rigorous modal analysis of partially-filled waveguides[8][9] or optimization methods[10] can be used. A modification of the method for single-port measurements was also reported.[11]
In addition to homogenous materials, the extension of the method was developed to obtain constitutive parameters of isotropic and bianisotropic metamaterials.[12][13][14]
See also
- Fourier-transform spectroscopy
- Microwave radiometer
- Reflection seismology
- Spectroscopy
- Time-domain reflectometer
- Vector network analyzer
References
- ↑ 1.0 1.1 Nicolson, A. M.; Ross, G. F. (November 1970). "Measurement of the intrinsic properties of materials by time-domain techniques". IEEE Transactions on Instrumentation and Measurement 19 (4): 377–382. doi:10.1109/TIM.1970.4313932. Bibcode: 1970ITIM...19..377N.
- ↑ 2.0 2.1 2.2 Weir, W. B. (January 1974). "Automatic measurement of complex dielectric constant and permeability at microwave frequencies". Proceedings of the IEEE 62 (1): 33–36. doi:10.1109/PROC.1974.9382.
- ↑ Costa, Filippo; Borgese, Michele; Degiorgi, Marco; Monorchio, Agostino (2017). "Electromagnetic Characterisation of Materials by Using Transmission/Reflection (T/R) Devices". Electronics 6 (4): 95. doi:10.3390/electronics6040095.
- ↑ Baker-Jarvis, J.; Vanzura, E. J.; Kissick, W. A. (August 1990). "Improved technique for determining complex permittivity with the transmission/reflection method". IEEE Transactions on Microwave Theory and Techniques 38 (8): 1096–1103. doi:10.1109/22.57336. Bibcode: 1990ITMTT..38.1096B. https://zenodo.org/record/1261115.
- ↑ Boughriet, A.-H.; Legrand, C.; Chapoton, A. (January 1997). "Noniterative stable transmission/reflection method for low-loss material complex permittivity determination". IEEE Transactions on Microwave Theory and Techniques 45 (1): 52–57. doi:10.1109/22.552032. Bibcode: 1997ITMTT..45...52B.
- ↑ Luukkonen, Olli; Maslovski, Stanislav I.; Tretyakov, Sergei A. (11 November 2011). "A Stepwise Nicolson–Ross–Weir-Based Material Parameter Extraction Method". IEEE Antennas and Wireless Propagation Letters 10: 1295–1298. doi:10.1109/LAWP.2011.2175897. Bibcode: 2011IAWPL..10.1295L.
- ↑ Champlin, K. S.; Glover, G. H. (August 1966). ""Gap Effect" in Measurement of Large Permittivities (Correspondence)". IEEE Transactions on Microwave Theory and Techniques 14 (8): 397–398. doi:10.1109/TMTT.1966.1126280. Bibcode: 1966ITMTT..14..397C.
- ↑ Catala-Civera, J. M.; Canos, A. J.; Penaranda-Foix, F. L.; de los Reyes Davo, E. (14 January 2003). "Accurate determination of the complex permittivity of materials with transmission reflection measurements in partially filled rectangular waveguides". IEEE Transactions on Microwave Theory and Techniques 51 (1): 16–24. doi:10.1109/TMTT.2002.806940. Bibcode: 2003ITMTT..51...16C.
- ↑ Wilson, S. B. (April 1988). "Modal analysis of the 'gap effect' in waveguide dielectric measurements". IEEE Transactions on Microwave Theory and Techniques 36 (4): 752–756. doi:10.1109/22.3581. Bibcode: 1988ITMTT..36..752W.
- ↑ Requena-Perez, M. E.; Albero-Ortiz, A.; Monzo-Cabrera, J.; Diaz-Morcillo, A. (615–624). "Combined use of genetic algorithms and gradient descent optmization methods for accurate inverse permittivity measurement". IEEE Transactions on Microwave Theory and Techniques 54 (2): 615–624. doi:10.1109/TMTT.2005.862671. Bibcode: 2006ITMTT..54..615R.
- ↑ Sahin, Seckin; Nahar, Niru K.; Sertel, Kubilay (July 2020). "A Simplified Nicolson–Ross–Weir Method for Material Characterization Using Single-Port Measurements". IEEE Transactions on Terahertz Science and Technology 10 (4): 404–410. doi:10.1109/TTHZ.2020.2980442. Bibcode: 2020ITTST..10..404S.
- ↑ Smith, D. R.; Schultz, S.; Markoš, P.; Soukoulis, C. M. (2002). "Determination of effective permittivity and permeability of metamaterials from reflection and transmission coefficients". Physical Review B 65 (19): 195104. doi:10.1103/PhysRevB.65.195104. Bibcode: 2002PhRvB..65s5104S.
- ↑ Chen, Xudong; Grzegorczyk, Tomasz M.; Wu, Bae-Ian; Pacheco, Jr., Joe; Kong, Jin Au (26 July 2004). "Robust method to retrieve the constitutive effective parameters of metamaterials". Physical Review E 70 (1 Pt 2): 016608. doi:10.1103/PhysRevE.70.016608. PMID 15324190. Bibcode: 2004PhRvE..70a6608C.
- ↑ Li, Zhaofeng; Aydin, Koray; Ozbay, Ekmel (2009). "Determination of the effective constitutive parameters of bianisotropic metamaterials from reflection and transmission coefficients". Physical Review E 79 (2): 026610. doi:10.1103/PhysRevE.79.026610. PMID 19391866. Bibcode: 2009PhRvE..79b6610L.
Further reading
- Chen, L. F.; Ong, C. K.; Neo, C. P.; Varadan, V. V.; Varadan, V. K. (2004). Microwave electronics: measurement and materials characterization. John Wiley & Sons. ISBN 9780470020456.
![]() | Original source: https://en.wikipedia.org/wiki/Nicolson–Ross–Weir method.
Read more |