Biology:Phase precession
Phase precession is a neurophysiological process in which the firing of action potentials by individual neurons is timed in relation to the phase of neural oscillations in the surrounding cells. In place cells, a type of neuron found in the hippocampal region of the brain, phase precession is believed to play a major role in the neural coding of information. John O'Keefe, who later shared the 2014 Nobel Prize in Physiology or Medicine for his discovery that place cells help form a "map" of the body's position in space, co-discovered phase precession with Michael Recce in 1993.[1][2]
Place cells
Pyramidal cells in the hippocampus called place cells play a significant role in self-location during movement over short distances.[3] As a rat moves along a path, individual place cells fire action potentials at an increased rate at particular positions along the path, termed "place fields". Each place cell's maximum firing rate – with action potentials occurring in rapid bursts – occurs at the position encoded by that cell; and that cell fires only occasionally when the animal is at other locations.[4] Within a relatively small path, the same cells are repeatedly activated as the animal returns to the same position.
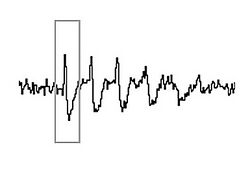
Although simple rate coding (the coding of information based on whether neurons fire more rapidly or more slowly) resulting from these changes in firing rates may account for some of the neural coding of position, there is also a prominent role for the timing of the action potentials of a single place cell, relative to the firing of nearby cells in the local population.[5][6] As the larger population of cells fire occasionally when the rat is outside of the cells' individual place fields, the firing patterns are organized to occur synchronously, forming wavelike voltage oscillations. These oscillations are measurable in local field potentials and electroencephalography (EEG). In the CA1 region of the hippocampus, where the place cells are located, these firing patterns give rise to theta waves.[7] Theta oscillations have classically been described in rats, but evidence is emerging that they also occur in humans.[8]
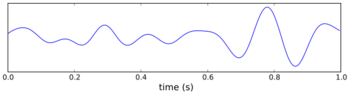
In 1993, O'Keefe and Recce discovered a relationship between the theta wave and the firing patterns of individual place cells.[1] Although the occasional action potentials of cells when rats were outside of the place fields occurred in phase with (at the peaks of) the theta waves, the bursts of more rapid spikes elicited when the rats reached the place fields were out of synchrony with the oscillation. As a rat approached the place field, the corresponding place cell would fire slightly in advance of the theta wave peak. As the rat moved closer and closer, each successive action potential occurred earlier and earlier within the wave cycle. At the center of the place field, when the cell would fire at its maximal rate, the firing had been advanced sufficiently to be anti-phase to the theta potential (at the bottom, rather than at the peak, of the theta waveform). Then, as the rat continued to move on past the place field and the cell firing slowed, the action potentials continued to occur progressively earlier relative to the theta wave, until they again became synchronous with the wave, aligned now with one wave peak earlier than before. O'Keefe and Recce termed this advancement relative to the wave phase "phase precession". Subsequent studies showed that each time a rat entered a completely different area and the place fields would be remapped, place cells would again become phase-locked to the theta rhythm.[9] It is now widely accepted that the anti-phase cell firing that results from phase precession is an important component of information coding about place.[3][5][6][7][10]
Other systems
There have been conflicting theories of how neurons in and around the hippocampus give rise to theta waves and consequently give rise to phase precession. As these mechanisms became better understood, the existence of phase precession was increasingly accepted by researchers.[10] This, in turn, gave rise to the question of whether phase precession could be observed in any other regions of the brain, with other kinds of cell circuits, or whether phase precession was a peculiar property of hippocampal tissue.[10] The finding that theta wave phase precession is also a property of grid cells in the entorhinal cortex demonstrated that the phenomenon exists in other parts of the brain that also mediate information about movement.[11]
Theta wave phase precession in the hippocampus also plays a role in some brain functions that are unrelated to spatial location. When rats were trained to jump up to the rim of a box, place cells displayed phase precession much as they do during movement along a path, but a subset of the place cells showed phase precession that was related to initiating the jump, independently of spatial location, and not related to the position during the jump.[12]
References
- ↑ 1.0 1.1 "Phase relationship between hippocampal place units and the EEG theta rhythm". Hippocampus 3 (3): 317–30. July 1993. doi:10.1002/hipo.450030307. PMID 8353611. Archived from the original on 29 January 2018. https://web.archive.org/web/20180129182136/http://onlinelibrary.wiley.com/doi/10.1002/hipo.450030307/abstract;jsessionid=D7DEB7FEC2C5F67890498798FC9414DE.f01t01?system.
- ↑ "The Nobel Prize in Physiology or Medicine 2014: John O'Keefe – Biograhpical". Archived from the original on 29 September 2017. https://web.archive.org/web/20170929044010/https://www.nobelprize.org/nobel_prizes/medicine/laureates/2014/okeefe-bio.html. Retrieved 27 January 2018.
- ↑ 3.0 3.1 "Place cells, grid cells, and the brain's spatial representation system". Annual Review of Neuroscience 31: 69–89. 19 February 2008. doi:10.1146/annurev.neuro.31.061307.090723. PMID 18284371. Archived from the original on 24 November 2016. https://web.archive.org/web/20161124223905/http://www.annualreviews.org/doi/10.1146/annurev.neuro.31.061307.090723.
- ↑ "Place cells and place navigation". Proceedings of the National Academy of Sciences of the United States of America 94 (1): 343–50. January 1997. doi:10.1073/pnas.94.1.343. PMID 8990211.
- ↑ 5.0 5.1 "Dual phase and rate coding in hippocampal place cells: theoretical significance and relationship to entorhinal grid cells". Hippocampus 15 (7): 853–66. 6 September 2005. doi:10.1002/hipo.20115. PMID 16145693. Archived from the original on 29 January 2018. https://web.archive.org/web/20180129175126/http://onlinelibrary.wiley.com/doi/10.1002/hipo.20115/abstract?system.
- ↑ 6.0 6.1 "Neuronal computations underlying the firing of place cells and their role in navigation". Hippocampus 6 (6): 749–62. 1996. doi:10.1002/(SICI)1098-1063(1996)6:6<749::AID-HIPO16>3.0.CO;2-0. PMID 9034860. Archived from the original on 29 January 2018. https://web.archive.org/web/20180129175123/http://onlinelibrary.wiley.com/doi/10.1002/(SICI)1098-1063(1996)6:6%3C749::AID-HIPO16%3E3.0.CO;2-0/abstract?system.
- ↑ 7.0 7.1 "Theta phase precession in hippocampal neuronal populations and the compression of temporal sequences". Hippocampus 6 (2): 149–72. 1996. doi:10.1002/(SICI)1098-1063(1996)6:2<149::AID-HIPO6>3.0.CO;2-K. PMID 8797016. Archived from the original on 29 January 2018. https://web.archive.org/web/20180129180632/http://onlinelibrary.wiley.com/doi/10.1002/(SICI)1098-1063(1996)6:2%3C149::AID-HIPO6%3E3.0.CO;2-K/abstract?system.
- ↑ "Low-frequency theta oscillations in the human hippocampus during real-world and virtual navigation". Nature Communications 8: 14415. February 2017. doi:10.1038/ncomms14415. PMID 28195129.
- ↑ "Phase precession and phase-locking of hippocampal pyramidal cells". Hippocampus 11 (3): 204–15. 19 June 2001. doi:10.1002/hipo.1038. PMID 11769305. Archived from the original on 29 January 2018. https://web.archive.org/web/20180129175120/http://onlinelibrary.wiley.com/doi/10.1002/hipo.1038/abstract?system.
- ↑ 10.0 10.1 10.2 Buzsáki, György (2006). Rhythms of the Brain. Oxford University Press. pp. 308–26. ISBN 0195301064. https://books.google.com/books?id=ldz58irprjYC&pg=PA308&.
- ↑ "Hippocampus-independent phase precession in entorhinal grid cells". Nature 453 (7199): 1248–52. June 2008. doi:10.1038/nature06957. PMID 18480753. Archived from the original on 30 January 2018. https://web.archive.org/web/20180130000010/http://www.nature.com/articles/nature06957.
- ↑ "Discharge properties of hippocampal neurons during performance of a jump avoidance task". The Journal of Neuroscience 28 (27): 6773–86. July 2008. doi:10.1523/JNEUROSCI.5329-07.2008. PMID 18596153.
![]() | Original source: https://en.wikipedia.org/wiki/Phase precession.
Read more |