Medicine:Living medicine

A living medicine is a type of biologic that consists of a living organism that is used to treat a disease. This usually takes the form of a cell (animal, bacterial, or fungal) or a virus that has been genetically engineered to possess therapeutic properties that is injected into a patient.[2][3] Perhaps the oldest use of a living medicine is the use of leeches for bloodletting, though living medicines have advanced tremendously since that time.
Examples of living medicines include cellular therapeutics (including immunotherapeutics), phage therapeutics, and bacterial therapeutics, a subset of the latter being probiotics.
Development of living medicines

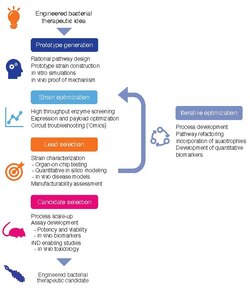
Development of living medicines is an extremely active research area in the fields of synthetic biology and microbiology.[6][7][8][9][10][11][12][13][14] Currently, there is a large focus on: 1) identifying microbes that naturally produce therapeutic effects (for example, probiotic bacteria), and 2) genetically programming organisms to produce therapeutic effects.[15][16][17]
Applications
Cancer therapy

There is tremendous interest in using bacteria as a therapy to treat tumors. In particular, tumor-homing bacteria that thrive in hypoxic environments are particularly attractive for this purpose, as they will tend to migrate to, invade (through the leaky vasculature in the tumor microenvironment) and colonize tumors. This property tends to increase their residence time in the tumor, giving them longer to exert their therapeutic effects, in contrast to other bacteria that would be quickly cleared by the immune system.[19][20][21][22]
References
- ↑
This article incorporates text by Pichet Praveschotinunt, Anna M. Duraj-Thatte, Ilia Gelfat, Franziska Bahl, David B. Chou & Neel S. Joshi available under the CC BY 4.0 license.
- ↑ editor, Ian Sample Science (16 January 2019). "'Living medicine' helps make toxic ammonia breakthrough". The Guardian. https://www.theguardian.com/science/2019/jan/16/living-medicine-helps-make-toxic-ammonia-breakthrough. Retrieved 5 April 2020.
- ↑ "Engineering Living Medicines for Chronic Diseases | SBE | Society for Biological Engineering". https://www.aiche.org/sbe/conferences/microbiome-engineering/2019/proceeding/paper/engineering-living-medicines-chronic-diseases.
- ↑
This article incorporates text by Mark R. Charbonneau, Vincent M. Isabella, Ning Li & Caroline B. Kurtz available under the CC BY 4.0 license.
- ↑
This article incorporates text by Mark R. Charbonneau, Vincent M. Isabella, Ning Li & Caroline B. Kurtz available under the CC BY 4.0 license.
- ↑ Weber, Wilfried; Fussenegger, Martin (January 2012). "Emerging biomedical applications of synthetic biology" (in en). Nature Reviews Genetics 13 (1): 21–35. doi:10.1038/nrg3094. ISSN 1471-0056. PMID 22124480.
- ↑ Fischbach, M. A.; Bluestone, J. A.; Lim, W. A. (2013-04-03). "Cell-Based Therapeutics: The Next Pillar of Medicine" (in en). Science Translational Medicine 5 (179): 179ps7. doi:10.1126/scitranslmed.3005568. ISSN 1946-6234. PMID 23552369.
- ↑ Kitada, Tasuku; DiAndreth, Breanna; Teague, Brian; Weiss, Ron (2018-02-09). "Programming gene and engineered-cell therapies with synthetic biology" (in en). Science 359 (6376): eaad1067. doi:10.1126/science.aad1067. ISSN 0036-8075. PMID 29439214.
- ↑ McCarty, Niko (18 December 2018). "Why 2018 Was the Year of 'Living' Medicine" (in en). Medium. https://medium.com/@NikoMcCarty/why-2018-was-the-year-of-living-medicine-af903df27722. Retrieved 5 April 2020.
- ↑ Kelly, Jason (12 June 2019). "The Era of Living Medicines". Ginkgo Bioworks. https://www.ginkgobioworks.com/2019/06/12/the-era-of-living-medicines/. Retrieved 5 April 2020.
- ↑ ServiceFeb. 18, Robert F. (18 February 2020). "From 'living' cement to medicine-delivering biofilms, biologists remake the material world" (in en). AAAS. https://www.science.org/content/article/living-cement-medicine-delivering-biofilms-biologists-remake-material-world. Retrieved 5 April 2020.
- ↑ Kurtz, Caroline B.; Millet, Yves A.; Puurunen, Marja K.; Perreault, Mylène; Charbonneau, Mark R.; Isabella, Vincent M.; Kotula, Jonathan W.; Antipov, Eugene et al. (2019-01-16). "An engineered E. coli Nissle improves hyperammonemia and survival in mice and shows dose-dependent exposure in healthy humans" (in en). Science Translational Medicine 11 (475): eaau7975. doi:10.1126/scitranslmed.aau7975. ISSN 1946-6234. PMID 30651324.
- ↑ Charbonneau, Mark R.; Isabella, Vincent M.; Li, Ning; Kurtz, Caroline B. (2020-04-08). "Developing a new class of engineered live bacterial therapeutics to treat human diseases" (in en). Nature Communications 11 (1): 1738. doi:10.1038/s41467-020-15508-1. ISSN 2041-1723. PMID 32269218. Bibcode: 2020NatCo..11.1738C.
- ↑ "Gene Circuits Empower Next-Generation Cell and Gene Therapies". GEN - Genetic Engineering and Biotechnology News. 1 February 2020. https://www.genengnews.com/insights/gene-circuits-empower-next-generation-cell-and-gene-therapies/. Retrieved 5 April 2020.
- ↑ "Why now is the time for programmable living medicines: insights from Jim Collins, Aoife Brennan, and Jason Kelly". SynBioBeta. 2 April 2019. https://synbiobeta.com/why-now-is-the-time-for-programmable-living-medicines/. Retrieved 5 April 2020.
- ↑ Costa, Kevin (20 February 2019). "Living medicines: Ginkgo's machine to disrupt the pharma industry". SynBioBeta. https://synbiobeta.com/living-medicines-ginkgos-machine-to-disrupt-the-pharma-industry/. Retrieved 5 April 2020.
- ↑ Gurbatri, Candice R.; Arpaia, Nicholas; Danino, Tal (25 November 2022). "Engineering bacteria as interactive cancer therapies". Science 378 (6622): 858–864. doi:10.1126/science.add9667. PMID 36423303. Bibcode: 2022Sci...378..858G.
- ↑
This article incorporates text by Mai Thi-Quynh Duong, Yeshan Qin, Sung-Hwan You & Jung-Joon Min available under the CC BY 4.0 license.
- ↑ Sieow, Brendan Fu-Long; Wun, Kwok Soon; Yong, Wei Peng; Hwang, In Young; Chang, Matthew Wook (December 2020). "Tweak to Treat: Reprograming Bacteria for Cancer Treatment". Trends in Cancer 7 (5): 447–464. doi:10.1016/j.trecan.2020.11.004. ISSN 2405-8033. PMID 33303401.
- ↑ Duong, Mai Thi-Quynh; Qin, Yeshan; You, Sung-Hwan; Min, Jung-Joon (2019-12-11). "Bacteria-cancer interactions: bacteria-based cancer therapy" (in en). Experimental & Molecular Medicine 51 (12): 1–15. doi:10.1038/s12276-019-0297-0. ISSN 2092-6413. PMID 31827064.
- ↑ Sedighi, Mansour; Zahedi Bialvaei, Abed; Hamblin, Michael R.; Ohadi, Elnaz; Asadi, Arezoo; Halajzadeh, Masoumeh; Lohrasbi, Vahid; Mohammadzadeh, Nima et al. (2019-04-05). "Therapeutic bacteria to combat cancer; current advances, challenges, and opportunities" (in en). Cancer Medicine 8 (6): 3167–3181. doi:10.1002/cam4.2148. ISSN 2045-7634. PMID 30950210.
- ↑ Song, Shiyu; Vuai, Miza S.; Zhong, Mintao (2018-03-15). "The role of bacteria in cancer therapy – enemies in the past, but allies at present" (in en). Infectious Agents and Cancer 13 (1): 9. doi:10.1186/s13027-018-0180-y. ISSN 1750-9378. PMID 29568324.
![]() | Original source: https://en.wikipedia.org/wiki/Living medicine.
Read more |