Physics:Magnetic resonance elastography
Magnetic resonance elastography (MRE) is a form of elastography that specifically leverages MRI to quantify and subsequently map the mechanical properties (elasticity or stiffness) of soft tissue. First developed and described at Mayo Clinic by Muthupillai et al. in 1995, MRE has emerged as a powerful, non-invasive diagnostic tool, namely as an alternative to biopsy and serum tests for staging liver fibrosis.[1][2][3][4][5] Diseased tissue (e.g. a breast tumor) is often stiffer than the surrounding normal (fibroglandular) tissue,[6] providing motivation to assess tissue stiffness.[7] This principle of operation is the basis for the longstanding practice of palpation, which, however, is limited (except at surgery) to superficial organs and pathologies, and by its subjective, qualitative nature, depending on the skill and touch sensitivity of the practitioner. Conventional imaging techniques of CT, MRI, US, and nuclear medicine are unable to offer any insight on the elastic modulus of soft tissue.[2] MRE, as a quantitative method of assessing tissue stiffness, provides reliable insight to visualize a variety of disease processes which affect tissue stiffness in the liver, brain, heart, pancreas, kidney, spleen, breast, uterus, prostate, and skeletal muscle.[2][3][8]
MRE is conducted in three steps: first, a mechanical vibrator is used on the surface of the patient's body to generate shear waves that travel into the patient's deeper tissues; second, an MRI acquisition sequence measures the propagation and velocity of the waves; and finally this information is processed by an inversion algorithm to quantitatively infer and map tissue stiffness in 3-D.[2][3] This stiffness map is called an elastogram, and is the final output of MRE, along with conventional 3-D MRI images as shown on the right.[2]
Mechanics of soft tissue
MRE quantitatively determines the stiffness of biological tissues by measuring its mechanical response to an external stress.[3] Specifically, MRE calculates the shear modulus of a tissue from its shear-wave displacement measurements.[7] The elastic modulus quantifies the stiffness of a material, or how well it resists elastic deformation as a force is applied. For elastic materials, strain is directly proportional to stress within an elastic region. The elastic modulus is seen as the proportionality constant between stress and strain within this region. Unlike purely elastic materials, biological tissues are viscoelastic, meaning that it has characteristics of both elastic solids and viscous liquids. Their mechanical responses depend on the magnitude of the applied stress as well as the strain rate. The stress-strain curve for a viscoelastic material exhibits hysteresis. The area of the hysteresis loop represents the amount of energy lost as heat when a viscoelastic material undergoes an applied stress and is distorted. For these materials, the elastic modulus is complex and can be separated into two components: a storage modulus and a loss modulus. The storage modulus expresses the contribution from elastic solid behavior while the loss modulus expresses the contribution from viscous liquid behavior. Conversely, elastic materials exhibit a pure solid response. When a force is applied, these materials elastically store and release energy, which does not result in energy loss in the form of heat.[9]
Yet, MRE and other elastography imaging techniques typically utilize a mechanical parameter estimation that assumes biological tissues to be linearly elastic and isotropic for simplicity purposes.[10] The effective shear modulus [math]\displaystyle{ \mu }[/math] can be expressed with the following equation:
[math]\displaystyle{ \mu=E/[2(1+\nu)] }[/math]
where [math]\displaystyle{ E }[/math] is the elastic modulus of the material and [math]\displaystyle{ \nu }[/math] is the Poisson’s ratio.
The Poisson’s ratio for soft tissues is approximated to equal 0.5, resulting in the ratio between the elastic modulus and shear modulus to equal 3.[11] This relationship can be used to estimate the stiffness of biological tissues based on the calculated shear modulus from shear-wave propagation measurements. A driver system produces and transmits acoustic waves set at a specific frequency (50–500 Hz) to the tissue sample. At these frequencies, the velocity of shear waves can be about 1–10 m/s.[12][13] The effective shear modulus can be calculated from the shear wave velocity with the following:[14]
[math]\displaystyle{ \mu=\rho{v_s}^2 }[/math]
where [math]\displaystyle{ \rho }[/math] is the tissue density and [math]\displaystyle{ v_s }[/math] is the shear wave velocity.
Recent studies have been focused on incorporating mechanical parameter estimations into post-processing inverse algorithms that account for the complex viscoelastic behavior of soft tissues. Creating new parameters could potentially increase the specificity of MRE measurements and diagnostic testing.[15][16]
Applications
Liver

Liver fibrosis is a common condition arising in many liver diseases. Progression of fibrosis can lead to cirrhosis and end-stage liver disease. MRE-based measurement of liver stiffness has emerged as the most accurate non-invasive technique for detecting and staging liver fibrosis. MRE provides quantitative maps of tissue stiffness over large regions of the liver. Abnormally increased liver stiffness is a direct consequence of liver fibrosis. The diagnostic performance of MRE in assessing liver fibrosis has been established in multiple studies.[17][18][16][19]
Liver MRE examinations are performed in MRI systems that have been equipped for the technique. Patients should fast for 3 to 4 hours prior to their MRE exam to allow for the most accurate measurement of liver stiffness.[20][21][22] Patients lie supine in the MRI scanner for the examination. A special device is placed on the right side of the chest wall over the liver to apply gentle vibration which generates propagating shear waves in the liver. Imaging is for MRE is very quick, with data acquired in a series of 1-4 periods of breath-holding, each lasting 15–20 seconds.
A standardized approach for performing and analyzing liver MRE exams has been documented by the RSNA Quantitative Imaging Biomarkers Alliance.[23] The technical success rate of Liver MRE is very high (95-100%)[24][25][26]
Brain
Magnetic resonance elastography | |
---|---|
Medical diagnostics | |
![]() Magnetic resonance elastography of the brain. A T1 weighted anatomical image is shown in the top-left, and the corresponding T2 weighted image from the MRE data is shown in the bottom-left. The wave image used to make the elastogram is shown in the top-right, and the resulting elastogram is in the bottom-right. | |
Purpose | measures the mechanical properties of soft tissues |
MRE of the brain [27] was first presented in the early 2000s.[28][29] Elastogram measures have been correlated with memory tasks,[30] fitness measures,[31] and progression of various neurodegenerative conditions.[27] For example, regional and global decreases in brain viscoelasticity have been observed in Alzheimer’s disease[32][33] and multiple sclerosis.[34][35] It has been found that as the brain ages, it loses its viscoelastic integrity due to degeneration of neurons and oligodendrocytes.[36][37] A recent study looked into both the isotropic and anisotropic stiffness in brain and found a correlation between the two and with age, particularly in gray matter.[38]
MRE may also have applications for understanding the adolescent brain. Recently, it was found that adolescents have regional differences in brain viscoelasticity relative to adults.[39][40]
MRE has also been applied to functional neuroimaging. Whereas functional magnetic resonance imaging (fMRI) infers brain activity by detecting relatively slow changes in blood flow, functional MRE is capable of detecting neuromechanical changes in the brain related to neuronal activity occurring on the 100-millisecond scale.[41]
Kidney
MRE has also been applied to investigate the biomechanical properties of the kidney. The feasibility of clinical renal MRE was first reported in 2011 for healthy volunteers [42] and in 2012 for renal transplant patients.[43] Renal MRE is more challenging than MRE of larger organs such as the brain or liver due to fine mechanical features in the renal cortex and medulla as well as the acoustically shielded position of the kidneys within the abdominal cavity. To overcome these challenges, researchers have been looking at different passive drivers and imaging techniques to best deliver shear waves to the kidneys.[44][45][46][47][48] Studies investigating renal diseases such as renal allograft dysfunction,[49][50][51][52] lupus nephritis,[53] immunoglobulin A nephropathy (IgAN),[54] diabetic nephrology,[55] renal tumors[56] and chronic kidney disease[57] demonstrate that kidney stiffness is sensitive to kidney function[58][59] and renal perfusion.[58][60]
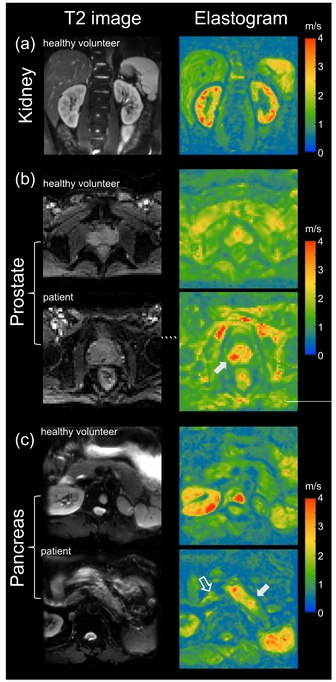
Prostate
The prostate can also be examined by MRE, in particular for the detection and diagnosis of prostate cancer.[61] To ensure good shear wave penetration in the prostate gland, different actuator systems were designed and evaluated.[62][63] Preliminary results in patients with prostate cancer showed that changes in stiffness allowed differentiation of cancerous tissue from normal tissue.[64] Magnetic Resonance Elastography has been successfully used in patients with prostate cancer showing high specificity and sensitivity in differentiating prostate cancer from benign prostatic diseases [65][66] (see figure on right (b)). Even higher specificity of 95% for prostate cancer was achieved when Magnetic Resonance Elastography was combined with systematic image interpretation using PI-RADS (version 2.1).[66][67]
Pancreas
The pancreas is one of the softest tissues in the abdomen. Given that pancreatic diseases including pancreatitis and pancreatic cancer significantly increase stiffness, MRE is a promising tool for diagnosing benign and malignant conditions of the pancreas. Abnormally high pancreatic stiffness was detected by MRE in patients with both acute and chronic pancreatitis.[68] Pancreatic stiffness was also used to distinguish pancreatic malignancy from benign masses [69] and to predict the occurrence of pancreatic fistula after pancreaticoenteric anastomosis.[70] Quantification of the volume of pancreatic tumors based on tomoelastographic measurement of stiffness was found to be excellently correlated with tumor volumes estimated by contrast-enhanced computed tomography.[71] In patients with pancreatic ductal adenocarcinoma stiffness was found to be elevated in the tumor as well as in pancreatic parenchyma distal to the tumor, suggesting heterogeneous pancreatic involvement [72] (figure on right (c)).
See also
References
- ↑ Hirsch, Sebastian; Braun, Jürgen; Sack, Ingolf (2016). Magnetic Resonance Elastography | Wiley Online Books. doi:10.1002/9783527696017. ISBN 9783527696017. https://www.onlinelibrary.wiley.com/doi/book/10.1002/9783527696017.
- ↑ 2.0 2.1 2.2 2.3 2.4 "Magnetic resonance elastography: a review". Clin Anat 23 (5): 497–511. 2010. doi:10.1002/ca.21006. PMID 20544947.
- ↑ 3.0 3.1 3.2 3.3 "Review of MR elastography applications and recent developments". J Magn Reson Imaging 36 (4): 757–74. 14 September 2012. doi:10.1002/jmri.23597. PMID 22987755.
- ↑ "MR Elastography of Liver Disease: State of the Art". Appl Radiol 42 (4): 5–12. 2013. doi:10.37549/AR1982. PMID 26366024.
- ↑ Ingolf Sack: Magnetic resonance elastography from fundamental soft-tissue mechanics to diagnostic imaging. In: Nature Reviews Physics. 5, 2023, S. 25, doi:10.1038/s42254-022-00543-2.
- ↑ "Magnetic resonance elastography (MRE) in cancer: Technique, analysis, and applications.". Prog Nucl Magn Reson Spectrosc 90-91: 32–48. 2015. doi:10.1016/j.pnmrs.2015.06.001. PMID 26592944.
- ↑ 7.0 7.1 "Magnetic resonance elastography by direct visualization of propagating acoustic strain waves". Science 269 (5232): 1854–7. September 1995. doi:10.1126/science.7569924. PMID 7569924. Bibcode: 1995Sci...269.1854M.
- ↑ Wang, Jin; Deng, Ying; Jondal, Danielle; Woodrum, David M.; Shi, Yu; Yin, Meng; Venkatesh, Sudhakar K. (2018). "New and Emerging Applications of Magnetic Resonance Elastography of Other Abdominal Organs". Topics in Magnetic Resonance Imaging 27 (5): 335–352. doi:10.1097/RMR.0000000000000182. ISSN 0899-3459. PMID 30289829.
- ↑ "Nonlinear Viscoelastic Solids—A Review" (in en). Mathematics and Mechanics of Solids 14 (3): 300–366. 2009. doi:10.1177/1081286509103660. ISSN 1081-2865. http://journals.sagepub.com/doi/10.1177/1081286509103660.
- ↑ "Magnetic resonance elastography: a review". Clinical Anatomy 23 (5): 497–511. July 2010. doi:10.1002/ca.21006. PMID 20544947.
- ↑ "General review of magnetic resonance elastography". World Journal of Radiology 8 (1): 59–72. January 2016. doi:10.4329/wjr.v8.i1.59. PMID 26834944.
- ↑ "Biophysical Bases of Elasticity Imaging". Acoustical Imaging. 21. Springer US. 1995. pp. 223–240. doi:10.1007/978-1-4615-1943-0_23. ISBN 978-1-4613-5797-1.
- ↑ Cameron, John (1991). "Physical Properties of Tissue. A Comprehensive Reference Book, edited by Francis A. Duck". Medical Physics 18 (4): 834. doi:10.1118/1.596734. Bibcode: 1991MedPh..18..834C.
- ↑ "Medical ultrasound: imaging of soft tissue strain and elasticity". Journal of the Royal Society, Interface 8 (64): 1521–49. November 2011. doi:10.1016/S1361-8415(00)00039-6. PMID 21680780.
- ↑ "Imaging anisotropic and viscous properties of breast tissue by magnetic resonance-elastography". Magnetic Resonance in Medicine 53 (2): 372–87. February 2005. doi:10.1002/mrm.20355. PMID 15678538.
- ↑ 16.0 16.1 "Viscoelasticity-based staging of hepatic fibrosis with multifrequency MR elastography". Radiology 257 (1): 80–6. October 2010. doi:10.1148/radiol.10092489. PMID 20679447.
- ↑ "Assessment of hepatic fibrosis with magnetic resonance elastography". Clinical Gastroenterology and Hepatology 5 (10): 1207–1213.e2. October 2007. doi:10.1016/j.cgh.2007.06.012. PMID 17916548.
- ↑ "Magnetic resonance elastography for the noninvasive staging of liver fibrosis". Gastroenterology 135 (1): 32–40. July 2008. doi:10.1053/j.gastro.2008.03.076. PMID 18471441.
- ↑ "Magnetic resonance elastography of liver: technique, analysis, and clinical applications". Journal of Magnetic Resonance Imaging 37 (3): 544–55. March 2013. doi:10.1002/jmri.23731. PMID 23423795.
- ↑ Jajamovich, Guido H.; Dyvorne, Hadrien; Donnerhack, Claudia; Taouli, Bachir (2014-05-19). "Quantitative Liver MRI Combining Phase Contrast Imaging, Elastography, and DWI: Assessment of Reproducibility and Postprandial Effect at 3.0 T". PLOS ONE 9 (5): e97355. doi:10.1371/journal.pone.0097355. ISSN 1932-6203. PMID 24840288. Bibcode: 2014PLoSO...997355J.
- ↑ Obrzut, Marzanna; Atamaniuk, Vitaliy; Chen, Jun; Obrzut, Bogdan; Ehman, Richard L.; Cholewa, Marian; Palusińska, Agnieszka; Gutkowski, Krzysztof (2021-10-05). "Postprandial hepatic stiffness changes on magnetic resonance elastography in healthy volunteers". Scientific Reports 11 (1): 19786. doi:10.1038/s41598-021-99243-7. ISSN 2045-2322. PMID 34611231. Bibcode: 2021NatSR..1119786O.
- ↑ Yin, Meng; Talwalkar, Jayant A.; Glaser, Kevin J.; Venkatesh, Sudhakar K.; Chen, Jun; Manduca, Armando; Ehman, Richard L. (July 2011). "Dynamic Postprandial Hepatic Stiffness Augmentation Assessed With MR Elastography in Patients With Chronic Liver Disease". AJR. American Journal of Roentgenology 197 (1): 64–70. doi:10.2214/AJR.10.5989. ISSN 0361-803X. PMID 21701012.
- ↑ "Profiles - QIBA Wiki". https://qibawiki.rsna.org/index.php/Profiles.
- ↑ Singh, Siddharth; Venkatesh, Sudhakar K.; Wang, Zhen; Miller, Frank H.; Motosugi, Utaroh; Low, Russell N.; Hassanein, Tarek; Asbach, Patrick et al. (March 2015). "Diagnostic Performance of Magnetic Resonance Elastography in Staging Liver Fibrosis: A Systematic Review and Meta-analysis of Individual Participant Data". Clinical Gastroenterology and Hepatology 13 (3): 440–451.e6. doi:10.1016/j.cgh.2014.09.046. ISSN 1542-3565. PMID 25305349.
- ↑ Kennedy, Paul; Wagner, Mathilde; Castéra, Laurent; Hong, Cheng William; Johnson, Curtis L.; Sirlin, Claude B.; Taouli, Bachir (March 2018). "Quantitative Elastography Methods in Liver Disease: Current Evidence and Future Directions". Radiology 286 (3): 738–763. doi:10.1148/radiol.2018170601. ISSN 0033-8419. PMID 29461949.
- ↑ Joshi, Madalsa; Dillman, Jonathan R.; Towbin, Alexander J.; Serai, Suraj D.; Trout, Andrew T. (June 2017). "MR elastography: high rate of technical success in pediatric and young adult patients". Pediatric Radiology 47 (7): 838–843. doi:10.1007/s00247-017-3831-z. ISSN 1432-1998. PMID 28367603. https://pubmed.ncbi.nlm.nih.gov/28367603.
- ↑ 27.0 27.1 "Magnetic resonance elastography (MRE) of the human brain: technique, findings and clinical applications". Phys Med Biol 61 (24): R401–R437. December 2016. doi:10.1088/0031-9155/61/24/R401. PMID 27845941. Bibcode: 2016PMB....61R.401H. https://www.pure.ed.ac.uk/ws/files/28342950/Hiscox2016_BrainMRE.PDF.
- ↑ "An overlapping subzone technique for MR-based elastic property reconstruction". Magnetic Resonance in Medicine 42 (4): 779–86. October 1999. doi:10.1002/(SICI)1522-2594(199910)42:4<779::AID-MRM21>3.0.CO;2-Z. PMID 10502768.
- ↑ "Three-dimensional subzone-based reconstruction algorithm for MR elastography". Magnetic Resonance in Medicine 45 (5): 827–37. May 2001. doi:10.1002/mrm.1111. PMID 11323809.
- ↑ "Medial temporal lobe viscoelasticity and relational memory performance". NeuroImage 132: 534–541. May 2016. doi:10.1016/j.neuroimage.2016.02.059. PMID 26931816.
- ↑ "Aerobic fitness, hippocampal viscoelasticity, and relational memory performance". NeuroImage 153: 179–188. June 2017. doi:10.1016/j.neuroimage.2017.03.061. PMID 28366763.
- ↑ "Decreased brain stiffness in Alzheimer's disease determined by magnetic resonance elastography". Journal of Magnetic Resonance Imaging 34 (3): 494–8. September 2011. doi:10.1002/jmri.22707. PMID 21751286.
- ↑ "Regional brain stiffness changes across the Alzheimer's disease spectrum". NeuroImage. Clinical 10: 283–90. 2016. doi:10.1016/j.nicl.2015.12.007. PMID 26900568.
- ↑ "Brain viscoelasticity alteration in chronic-progressive multiple sclerosis". PLOS ONE 7 (1): e29888. 2012. doi:10.1371/journal.pone.0029888. PMID 22276134. Bibcode: 2012PLoSO...729888S.
- ↑ "Exercise training effects on memory and hippocampal viscoelasticity in multiple sclerosis: a novel application of magnetic resonance elastography". Neuroradiology 59 (1): 61–67. January 2017. doi:10.1007/s00234-016-1767-x. PMID 27889837.
- ↑ "The impact of aging and gender on brain viscoelasticity". NeuroImage 46 (3): 652–7. July 2009. doi:10.1016/j.neuroimage.2009.02.040. PMID 19281851.
- ↑ "The influence of physiological aging and atrophy on brain viscoelastic properties in humans". PLOS ONE 6 (9): e23451. 2011. doi:10.1371/journal.pone.0023451. PMID 21931599. Bibcode: 2011PLoSO...623451S.
- ↑ "Magnetic resonance elastography of brain: Comparison between anisotropic and isotropic stiffness and its correlation to age". Magnetic Resonance in Medicine 82 (2): 671–679. August 2019. doi:10.1002/mrm.27757. PMID 30957304.
- ↑ "Magnetic resonance elastography for examining developmental changes in the mechanical properties of the brain". Developmental Cognitive Neuroscience 33: 176–181. October 2018. doi:10.1016/j.dcn.2017.08.010. PMID 29239832.
- ↑ "Mechanical properties of the in vivo adolescent human brain". Developmental Cognitive Neuroscience 34: 27–33. November 2018. doi:10.1016/j.dcn.2018.06.001. PMID 29906788.
- ↑ Bridger, Haley (17 April 2019). "Seeing brain activity in 'almost real time'". Harvard Gazette. https://news.harvard.edu/gazette/story/2019/04/harvard-unveils-new-technique-60-times-faster-than-traditional-fmri/.
- ↑ "Magnetic resonance elastography of the kidneys: feasibility and reproducibility in young healthy adults". J Magn Reson Imaging 34 (4): 880–6. October 2011. doi:10.1002/jmri.22670. PMID 21769970.
- ↑ "MR elastography in renal transplant patients and correlation with renal allograft biopsy: a feasibility study". Acad Radiol 19 (7): 834–41. July 2012. doi:10.1016/j.acra.2012.03.003. PMID 22503893.
- ↑ Bensamoun, Sabine F.; Robert, Ludovic; Leclerc, Gwladys E.; Debernard, Laëtitia; Charleux, Fabrice (July 2011). "Stiffness imaging of the kidney and adjacent abdominal tissues measured simultaneously using magnetic resonance elastography". Clinical Imaging 35 (4): 284–287. doi:10.1016/j.clinimag.2010.07.009. ISSN 1873-4499. PMID 21724121. https://pubmed.ncbi.nlm.nih.gov/21724121.
- ↑ Low, Gavin; Owen, Nicola E.; Joubert, Ilse; Patterson, Andrew J.; Graves, Martin J.; Alexander, Graeme J. M.; Lomas, David J. (October 2015). "Magnetic resonance elastography in the detection of hepatorenal syndrome in patients with cirrhosis and ascites". European Radiology 25 (10): 2851–2858. doi:10.1007/s00330-015-3723-2. ISSN 1432-1084. PMID 25903705. https://pubmed.ncbi.nlm.nih.gov/25903705.
- ↑ Zhang, Jiong; Yu, Yuanmeng; Liu, Xiaoshuang; Tang, Xiong; Xu, Feng; Zhang, Mingchao; Xie, Guotong; Zhang, Longjiang et al. (March 2021). "Evaluation of Renal Fibrosis by Mapping Histology and Magnetic Resonance Imaging". Kidney Diseases (Basel, Switzerland) 7 (2): 131–142. doi:10.1159/000513332. ISSN 2296-9381. PMID 33824869.
- ↑ Gandhi, Deep; Kalra, Prateek; Raterman, Brian; Mo, Xiaokui; Dong, Huiming; Kolipaka, Arunark (November 2019). "Magnetic Resonance Elastography of kidneys: SE-EPI MRE reproducibility and its comparison to GRE MRE". NMR in Biomedicine 32 (11): e4141. doi:10.1002/nbm.4141. ISSN 1099-1492. PMID 31329347.
- ↑ Low, Gavin; Owen, Nicola E.; Joubert, Ilse; Patterson, Andrew J.; Graves, Martin J.; Glaser, Kevin J.; Alexander, Graeme J. M.; Lomas, David J. (September 2015). "Reliability of magnetic resonance elastography using multislice two-dimensional spin-echo echo-planar imaging (SE-EPI) and three-dimensional inversion reconstruction for assessing renal stiffness". Journal of Magnetic Resonance Imaging 42 (3): 844–850. doi:10.1002/jmri.24826. ISSN 1522-2586. PMID 25537823.
- ↑ Marticorena Garcia, Stephan Rodrigo; Fischer, Thomas; Dürr, Michael; Gültekin, Emin; Braun, Jürgen; Sack, Ingolf; Guo, Jing (September 2016). "Multifrequency Magnetic Resonance Elastography for the Assessment of Renal Allograft Function". Investigative Radiology 51 (9): 591–595. doi:10.1097/RLI.0000000000000271. ISSN 1536-0210. PMID 27504796. https://pubmed.ncbi.nlm.nih.gov/27504796.
- ↑ Kim, J. K.; Yuen, D. A.; Leung, G.; Jothy, S.; Zaltzman, J.; Ramesh Prasad, G. V.; Prabhudesai, V.; Mnatzakanian, G. et al. (September 2017). "Role of Magnetic Resonance Elastography as a Noninvasive Measurement Tool of Fibrosis in a Renal Allograft: A Case Report". Transplantation Proceedings 49 (7): 1555–1559. doi:10.1016/j.transproceed.2017.04.002. ISSN 1873-2623. PMID 28838439. https://pubmed.ncbi.nlm.nih.gov/28838439.
- ↑ Kirpalani, Anish; Hashim, Eyesha; Leung, General; Kim, Jin K.; Krizova, Adriana; Jothy, Serge; Deeb, Maya; Jiang, Nan N. et al. (2017-10-06). "Magnetic Resonance Elastography to Assess Fibrosis in Kidney Allografts". Clinical Journal of the American Society of Nephrology 12 (10): 1671–1679. doi:10.2215/CJN.01830217. ISSN 1555-905X. PMID 28855238.
- ↑ Marticorena Garcia, Stephan R.; Althoff, Christian E.; Dürr, Michael; Halleck, Fabian; Budde, Klemens; Grittner, Ulrike; Burkhardt, Christian; Jöhrens, Korinna et al. (2021-02-01). "Tomoelastography for Longitudinal Monitoring of Viscoelasticity Changes in the Liver and in Renal Allografts after Direct-Acting Antiviral Treatment in 15 Kidney Transplant Recipients with Chronic HCV Infection". Journal of Clinical Medicine 10 (3): 510. doi:10.3390/jcm10030510. ISSN 2077-0383. PMID 33535495.
- ↑ Marticorena Garcia, Stephan Rodrigo; Grossmann, Markus; Bruns, Anne; Dürr, Michael; Tzschätzsch, Heiko; Hamm, Bernd; Braun, Jürgen; Sack, Ingolf et al. (February 2019). "Tomoelastography Paired With T2* Magnetic Resonance Imaging Detects Lupus Nephritis With Normal Renal Function". Investigative Radiology 54 (2): 89–97. doi:10.1097/RLI.0000000000000511. ISSN 1536-0210. PMID 30222647. https://pubmed.ncbi.nlm.nih.gov/30222647.
- ↑ Lang, Sophia Theresa; Guo, Jing; Bruns, Anne; Dürr, Michael; Braun, Jürgen; Hamm, Bernd; Sack, Ingolf; Marticorena Garcia, Stephan Rodrigo (October 2019). "Multiparametric Quantitative MRI for the Detection of IgA Nephropathy Using Tomoelastography, DWI, and BOLD Imaging". Investigative Radiology 54 (10): 669–674. doi:10.1097/RLI.0000000000000585. ISSN 1536-0210. PMID 31261295. https://pubmed.ncbi.nlm.nih.gov/31261295.
- ↑ Brown, Robert S.; Sun, Maryellen R. M.; Stillman, Isaac E.; Russell, Teresa L.; Rosas, Sylvia E.; Wei, Jesse L. (2020-06-01). "The utility of magnetic resonance imaging for noninvasive evaluation of diabetic nephropathy". Nephrology, Dialysis, Transplantation 35 (6): 970–978. doi:10.1093/ndt/gfz066. ISSN 1460-2385. PMID 31329940.
- ↑ Prezzi, Davide; Neji, Radhouene; Kelly-Morland, Christian; Verma, Hema; OʼBrien, Tim; Challacombe, Ben; Fernando, Archana; Chandra, Ashish et al. (June 2018). "Characterization of Small Renal Tumors With Magnetic Resonance Elastography: A Feasibility Study". Investigative Radiology 53 (6): 344–351. doi:10.1097/RLI.0000000000000449. ISSN 1536-0210. PMID 29462024. https://pubmed.ncbi.nlm.nih.gov/29462024.
- ↑ Han, Jun Hee; Ahn, Jhii-Hyun; Kim, Jae-Seok (December 2020). "Magnetic resonance elastography for evaluation of renal parenchyma in chronic kidney disease: a pilot study". La Radiologia Medica 125 (12): 1209–1215. doi:10.1007/s11547-020-01210-1. ISSN 1826-6983. PMID 32367323. https://pubmed.ncbi.nlm.nih.gov/32367323.
- ↑ 58.0 58.1 Güven, Alper Tuna; Idilman, Ilkay S.; Cebrayilov, Cebrayil; Önal, Ceren; Kibar, Müge Üzerk; Sağlam, Arzu; Yıldırım, Tolga; Yılmaz, Rahmi et al. (January 2022). "Evaluation of renal fibrosis in various causes of glomerulonephritis by MR elastography: a clinicopathologic comparative analysis". Abdominal Radiology 47 (1): 288–296. doi:10.1007/s00261-021-03296-1. ISSN 2366-0058. PMID 34633496. https://pubmed.ncbi.nlm.nih.gov/34633496.
- ↑ Dittmann, Florian; Tzschätzsch, Heiko; Hirsch, Sebastian; Barnhill, Eric; Braun, Jürgen; Sack, Ingolf; Guo, Jing (September 2017). "Tomoelastography of the abdomen: Tissue mechanical properties of the liver, spleen, kidney, and pancreas from single MR elastography scans at different hydration states". Magnetic Resonance in Medicine 78 (3): 976–983. doi:10.1002/mrm.26484. ISSN 1522-2594. PMID 27699875.
- ↑ Marticorena Garcia, Stephan Rodrigo; Grossmann, Markus; Lang, Sophia Theresa; Tzschätzsch, Heiko; Dittmann, Florian; Hamm, Bernd; Braun, Jürgen; Guo, Jing et al. (April 2018). "Tomoelastography of the native kidney: Regional variation and physiological effects on in vivo renal stiffness". Magnetic Resonance in Medicine 79 (4): 2126–2134. doi:10.1002/mrm.26892. ISSN 1522-2594. PMID 28856718. https://pubmed.ncbi.nlm.nih.gov/28856718.
- ↑ "MR elastography of the prostate: initial in-vivo application". RöFo 176 (8): 1094–9. August 2004. doi:10.1055/s-2004-813279. PMID 15346284.
- ↑ "Prostate MR elastography with transperineal electromagnetic actuation and a fast fractionally encoded steady-state gradient echo sequence". NMR Biomed 27 (7): 784–94. July 2014. doi:10.1002/nbm.3118. PMID 24764278.
- ↑ "Incorporating endorectal MR elastography into multi-parametric MRI for prostate cancer imaging: Initial feasibility in volunteers". J Magn Reson Imaging 38 (5): 1251–60. November 2013. doi:10.1002/jmri.24028. PMID 23408516.
- ↑ "MR elastography of prostate cancer: quantitative comparison with histopathology and repeatability of methods". NMR Biomed 28 (1): 124–39. January 2015. doi:10.1002/nbm.3218. PMID 25395244.
- ↑ "In Vivo Quantification of Water Diffusion, Stiffness, and Tissue Fluidity in Benign Prostatic Hyperplasia and Prostate Cancer". Invest Radiol 55 (8): 524–530. August 2020. doi:10.1097/RLI.0000000000000685. PMID 32496317.
- ↑ 66.0 66.1 "Tomoelastography Based on Multifrequency MR Elastography for Prostate Cancer Detection: Comparison with Multiparametric MRI". Radiology 299 (2): 362–370. 2021. doi:10.1148/radiol.2021201852. PMID 33687285.
- ↑ "Tomoelastography of the Prostate: Use of Tissue Stiffness for Improved Cancer Detection". Radiology 299 (2): 371–373. March 2021. doi:10.1148/radiol.2021210292. PMID 33689473.
- ↑ ("3D MR elastography of the pancreas in children". Abdom Radiol (NY) 44 (5): 1834–1840. May 2019. doi:10.1007/s00261-019-01903-w. PMID 30683979.
- ↑ "Differentiation of benign and malignant solid pancreatic masses using magnetic resonance elastography with spin-echo echo planar imaging and three-dimensional inversion reconstruction: a prospective study". Eur Radiol 28 (3): 936–945. March 2018. doi:10.1007/s00330-017-5062-y. PMID 28986646.
- ↑ "Pancreatic Stiffness Quantified with MR Elastography: Relationship to Postoperative Pancreatic Fistula after Pancreaticoenteric Anastomosis". Radiology 288 (2): 476–484. August 2018. doi:10.1148/radiol.2018170450. PMID 29664337.
- ↑ "Tomoelastography for Measurement of Tumor Volume Related to Tissue Stiffness in Pancreatic Ductal Adenocarcinomas". Invest Radiol 55 (12): 769–774. December 2020. doi:10.1097/RLI.0000000000000704. PMID 32796197.
- ↑ "Distinguishing pancreatic cancer and autoimmune pancreatitis with in vivo tomoelastography". Eur Radiol 31 (5): 3366–3374. October 2020. doi:10.1007/s00330-020-07420-5. PMID 33125553.
![]() | Original source: https://en.wikipedia.org/wiki/Magnetic resonance elastography.
Read more |