Physics:Magnetic resonance imaging
Magnetic resonance imaging | |
---|---|
Medical diagnostics | |
File:Structural MRI animation.ogv Para-sagittal MRI of the head, with aliasing artifacts (nose and forehead appear at the back of the head) | |
Synonyms | Nuclear magnetic resonance imaging (NMRI), magnetic resonance tomography (MRT) |
ICD-9-CM | 88.91 |
MeSH | D008279 |
MedlinePlus | 003335 |
Magnetic resonance imaging (MRI) is a medical imaging technique used in radiology to form pictures of the anatomy and the physiological processes of the body. MRI scanners use strong magnetic fields, magnetic field gradients, and radio waves to generate images of the organs in the body. MRI does not involve X-rays or the use of ionizing radiation, which distinguishes it from computed tomography (CT) and positron emission tomography (PET) scans. MRI is a medical application of nuclear magnetic resonance (NMR) which can also be used for imaging in other NMR applications, such as NMR spectroscopy.[1]
MRI is widely used in hospitals and clinics for medical diagnosis, staging and follow-up of disease. Compared to CT, MRI provides better contrast in images of soft tissues, e.g. in the brain or abdomen. However, it may be perceived as less comfortable by patients, due to the usually longer and louder measurements with the subject in a long, confining tube, although "open" MRI designs mostly relieve this. Additionally, implants and other non-removable metal in the body can pose a risk and may exclude some patients from undergoing an MRI examination safely.
MRI was originally called NMRI (nuclear magnetic resonance imaging), but "nuclear" was dropped to avoid negative associations.[2] Certain atomic nuclei are able to absorb radio frequency (RF) energy when placed in an external magnetic field; the resultant evolving spin polarization can induce a RF signal in a radio frequency coil and thereby be detected.[3] In clinical and research MRI, hydrogen atoms are most often used to generate a macroscopic polarization that is detected by antennas close to the subject being examined.[3] Hydrogen atoms are naturally abundant in humans and other biological organisms, particularly in water and fat. For this reason, most MRI scans essentially map the location of water and fat in the body. Pulses of radio waves excite the nuclear spin energy transition, and magnetic field gradients localize the polarization in space. By varying the parameters of the pulse sequence, different contrasts may be generated between tissues based on the relaxation properties of the hydrogen atoms therein.
Since its development in the 1970s and 1980s, MRI has proven to be a versatile imaging technique. While MRI is most prominently used in diagnostic medicine and biomedical research, it also may be used to form images of non-living objects, such as mummies. Diffusion MRI and functional MRI extend the utility of MRI to capture neuronal tracts and blood flow respectively in the nervous system, in addition to detailed spatial images. The sustained increase in demand for MRI within health systems has led to concerns about cost effectiveness and overdiagnosis.[4][5][dubious ]
Mechanism
Construction and physics
In most medical applications, hydrogen nuclei, which consist solely of a proton, that are in tissues create a signal that is processed to form an image of the body in terms of the density of those nuclei in a specific region. Given that the protons are affected by fields from other atoms to which they are bonded, it is possible to separate responses from hydrogen in specific compounds. To perform a study, the person is positioned within an MRI scanner that forms a strong magnetic field around the area to be imaged. First, energy from an oscillating magnetic field is temporarily applied to the patient at the appropriate resonance frequency. Scanning with X and Y gradient coils causes a selected region of the patient to experience the exact magnetic field required for the energy to be absorbed. The atoms are excited by a RF pulse and the resultant signal is measured by a receiving coil. The RF signal may be processed to deduce position information by looking at the changes in RF level and phase caused by varying the local magnetic field using gradient coils. As these coils are rapidly switched during the excitation and response to perform a moving line scan, they create the characteristic repetitive noise of an MRI scan as the windings move slightly due to magnetostriction. The contrast between different tissues is determined by the rate at which excited atoms return to the equilibrium state. Exogenous contrast agents may be given to the person to make the image clearer.[6]
The major components of an MRI scanner are the main magnet, which polarizes the sample, the shim coils for correcting shifts in the homogeneity of the main magnetic field, the gradient system which is used to localize the region to be scanned and the RF system, which excites the sample and detects the resulting NMR signal. The whole system is controlled by one or more computers.
MRI requires a magnetic field that is both strong and uniform to a few parts per million across the scan volume. The field strength of the magnet is measured in teslas – and while the majority of systems operate at 1.5 T, commercial systems are available between 0.2 and 7 T. Whole-body MRI systems for research applications operate in e.g. 9.4T,[7][8] 10.5T,[9] 11.7T.[10] Even higher field whole-body MRI systems e.g. 14 T and beyond are in conceptual proposal[11] or in engineering design.[12] Most clinical magnets are superconducting magnets, which require liquid helium to keep them at low temperatures. Lower field strengths can be achieved with permanent magnets, which are often used in "open" MRI scanners for claustrophobic patients.[13] Lower field strengths are also used in a portable MRI scanner approved by the FDA in 2020.[14] Recently, MRI has been demonstrated also at ultra-low fields, i.e., in the microtesla-to-millitesla range, where sufficient signal quality is made possible by prepolarization (on the order of 10–100 mT) and by measuring the Larmor precession fields at about 100 microtesla with highly sensitive superconducting quantum interference devices (SQUIDs).[15][16][17]
T1 and T2
Each tissue returns to its equilibrium state after excitation by the independent relaxation processes of T1 (spin-lattice; that is, magnetization in the same direction as the static magnetic field) and T2 (spin-spin; transverse to the static magnetic field). To create a T1-weighted image, magnetization is allowed to recover before measuring the MR signal by changing the repetition time (TR). This image weighting is useful for assessing the cerebral cortex, identifying fatty tissue, characterizing focal liver lesions, and in general, obtaining morphological information, as well as for post-contrast imaging. To create a T2-weighted image, magnetization is allowed to decay before measuring the MR signal by changing the echo time (TE). This image weighting is useful for detecting edema and inflammation, revealing white matter lesions, and assessing zonal anatomy in the prostate and uterus.
The information from MRI scans comes in the form of image contrasts based on differences in the rate of relaxation of nuclear spins following their perturbation by an oscillating magnetic field (in the form of radiofrequency pulses through the sample).[18] The relaxation rates are a measure of the time it takes for a signal to decay back to an equilibrium state from either the longitudinal or transverse plane.
Magnetization builds up along the z-axis in the presence of a magnetic field, B0, such that the magnetic dipoles in the sample will, on average, align with the z-axis summing to a total magnetization Mz. This magnetization along z is defined as the equilibrium magnetization; magnetization is defined as the sum of all magnetic dipoles in a sample. Following the equilibrium magnetization, a 90° radiofrequency (RF) pulse flips the direction of the magnetization vector in the xy-plane, and is then switched off. The initial magnetic field B0, however, is still applied. Thus, the spin magnetization vector will slowly return from the xy-plane back to the equilibrium state. The time it takes for the magnetization vector to return to its equilibrium value, Mz, is referred to as the longitudinal relaxation time, T1.[19] Subsequently, the rate at which this happens is simply the reciprocal of the relaxation time: [math]\displaystyle{ \frac {1} {T1} = R1 }[/math]. Similarly, the time in which it takes for Mxy to return to zero is T2, with the rate [math]\displaystyle{ \frac {1} {T2} = R2 }[/math].[20] Magnetization as a function of time is defined by the Bloch equations.
T1 and T2 values are dependent on the chemical environment of the sample; hence their utility in MRI. Soft tissue and muscle tissue relax at different rates, yielding the image contrast in a typical scan.
The standard display of MR images is to represent fluid characteristics in black-and-white images, where different tissues turn out as follows:
Signal | T1-weighted | T2-weighted |
---|---|---|
High |
|
|
Intermediate | Gray matter darker than white matter[23] | White matter darker than grey matter[23] |
Low |
|
Diagnostics
Usage by organ or system
MRI has a wide range of applications in medical diagnosis and more than 25,000 scanners are estimated to be in use worldwide.[24] MRI affects diagnosis and treatment in many specialties although the effect on improved health outcomes is disputed in certain cases.[25][26]
MRI is the investigation of choice in the preoperative staging of rectal and prostate cancer and has a role in the diagnosis, staging, and follow-up of other tumors,[27] as well as for determining areas of tissue for sampling in biobanking.[28][29]
Neuroimaging
MRI is the investigative tool of choice for neurological cancers over CT, as it offers better visualization of the posterior cranial fossa, containing the brainstem and the cerebellum. The contrast provided between grey and white matter makes MRI the best choice for many conditions of the central nervous system, including demyelinating diseases, dementia, cerebrovascular disease, infectious diseases, Alzheimer's disease and epilepsy.[30][31][32] Since many images are taken milliseconds apart, it shows how the brain responds to different stimuli, enabling researchers to study both the functional and structural brain abnormalities in psychological disorders.[33] MRI also is used in guided stereotactic surgery and radiosurgery for treatment of intracranial tumors, arteriovenous malformations, and other surgically treatable conditions using a device known as the N-localizer.[34][35][36] New tools that implement artificial intelligence in healthcare have demonstrated higher image quality and morphometric analysis in neuroimaging with the application of a denoising system.[37]
The record for the highest spatial resolution of a whole intact brain (postmortem) is 100 microns, from Massachusetts General Hospital. The data was published in NATURE on 30 October 2019.[38][39]
Cardiovascular
Cardiac MRI is complementary to other imaging techniques, such as echocardiography, cardiac CT, and nuclear medicine. It can be used to assess the structure and the function of the heart.[40] Its applications include assessment of myocardial ischemia and viability, cardiomyopathies, myocarditis, iron overload, vascular diseases, and congenital heart disease.[41]
Musculoskeletal
Applications in the musculoskeletal system include spinal imaging, assessment of joint disease, and soft tissue tumors.[42] Also, MRI techniques can be used for diagnostic imaging of systemic muscle diseases including genetic muscle diseases.[43][44]
Swallowing movement of throat and oesophagus can cause motion artifact over the imaged spine. Therefore, a saturation pulse[clarification needed] applied over this region the throat and oesophagus can help to avoid this artifact. Motion artifact arising due to pumping of the heart can be reduced by timing the MRI pulse according to heart cycles.[45] Blood vessels flow artifacts can be reduced by applying saturation pulses above and below the region of interest.[46]
Liver and gastrointestinal
Hepatobiliary MR is used to detect and characterize lesions of the liver, pancreas, and bile ducts. Focal or diffuse disorders of the liver may be evaluated using diffusion-weighted, opposed-phase imaging and dynamic contrast enhancement sequences. Extracellular contrast agents are used widely in liver MRI, and newer hepatobiliary contrast agents also provide the opportunity to perform functional biliary imaging. Anatomical imaging of the bile ducts is achieved by using a heavily T2-weighted sequence in magnetic resonance cholangiopancreatography (MRCP). Functional imaging of the pancreas is performed following administration of secretin. MR enterography provides non-invasive assessment of inflammatory bowel disease and small bowel tumors. MR-colonography may play a role in the detection of large polyps in patients at increased risk of colorectal cancer.[47][48][49][50]
Angiography
Magnetic resonance angiography (MRA) generates pictures of the arteries to evaluate them for stenosis (abnormal narrowing) or aneurysms (vessel wall dilatations, at risk of rupture). MRA is often used to evaluate the arteries of the neck and brain, the thoracic and abdominal aorta, the renal arteries, and the legs (called a "run-off"). A variety of techniques can be used to generate the pictures, such as administration of a paramagnetic contrast agent (gadolinium) or using a technique known as "flow-related enhancement" (e.g., 2D and 3D time-of-flight sequences), where most of the signal on an image is due to blood that recently moved into that plane (see also FLASH MRI).[51]
Techniques involving phase accumulation (known as phase contrast angiography) can also be used to generate flow velocity maps easily and accurately. Magnetic resonance venography (MRV) is a similar procedure that is used to image veins. In this method, the tissue is now excited inferiorly, while the signal is gathered in the plane immediately superior to the excitation plane—thus imaging the venous blood that recently moved from the excited plane.[52]
Contrast agents
MRI for imaging anatomical structures or blood flow do not require contrast agents since the varying properties of the tissues or blood provide natural contrasts. However, for more specific types of imaging, exogenous contrast agents may be given intravenously, orally, or intra-articularly.[6] Most contrast agents are either paramagnetic (e.g.: gadolinium, manganese, europium), and are used to shorten T1 in the tissue they accumulate in, or super-paramagnetic (SPIONs), and are used to shorten T2 and T2* in healthy tissue reducing its signal intensity (negative contrast agents). The most commonly used intravenous contrast agents are based on chelates of gadolinium, which is highly paramagnetic.[53] In general, these agents have proved safer than the iodinated contrast agents used in X-ray radiography or CT. Anaphylactoid reactions are rare, occurring in approx. 0.03–0.1%.[54] Of particular interest is the lower incidence of nephrotoxicity, compared with iodinated agents, when given at usual doses—this has made contrast-enhanced MRI scanning an option for patients with renal impairment, who would otherwise not be able to undergo contrast-enhanced CT.[55]
Gadolinium-based contrast reagents are typically octadentate complexes of gadolinium(III). The complex is very stable (log K > 20) so that, in use, the concentration of the un-complexed Gd3+ ions should be below the toxicity limit. The 9th place in the metal ion's coordination sphere is occupied by a water molecule which exchanges rapidly with water molecules in the reagent molecule's immediate environment, affecting the magnetic resonance relaxation time.[56] For details see MRI contrast agent.
In December 2017, the Food and Drug Administration (FDA) in the United States announced in a drug safety communication that new warnings were to be included on all gadolinium-based contrast agents (GBCAs). The FDA also called for increased patient education and requiring gadolinium contrast vendors to conduct additional animal and clinical studies to assess the safety of these agents.[57] Although gadolinium agents have proved useful for patients with kidney impairment, in patients with severe kidney failure requiring dialysis there is a risk of a rare but serious illness, nephrogenic systemic fibrosis, which may be linked to the use of certain gadolinium-containing agents. The most frequently linked is gadodiamide, but other agents have been linked too.[58] Although a causal link has not been definitively established, current guidelines in the United States are that dialysis patients should only receive gadolinium agents where essential and that dialysis should be performed as soon as possible after the scan to remove the agent from the body promptly.[59][60]
In Europe, where more gadolinium-containing agents are available, a classification of agents according to potential risks has been released.[61][62] In 2008, a new contrast agent named gadoxetate, brand name Eovist (US) or Primovist (EU), was approved for diagnostic use: This has the theoretical benefit of a dual excretion path.[63]
Sequences
An MRI sequence is a particular setting of radiofrequency pulses and gradients, resulting in a particular image appearance.[64] The T1 and T2 weighting can also be described as MRI sequences.
Overview table
This table does not include uncommon and experimental sequences.
Group | Sequence | Abbr. | Physics | Main clinical distinctions | Example |
---|---|---|---|---|---|
Spin echo | T1 weighted | T1 | Measuring spin–lattice relaxation by using a short repetition time (TR) and echo time (TE). |
Standard foundation and comparison for other sequences |
![]() |
T2 weighted | T2 | Measuring spin–spin relaxation by using long TR and TE times |
Standard foundation and comparison for other sequences |
![]() | |
Proton density weighted | PD | Long TR (to reduce T1) and short TE (to minimize T2).[67] | Joint disease and injury.[68]
|
![]() | |
Gradient echo (GRE) | Steady-state free precession | SSFP | Maintenance of a steady, residual transverse magnetisation over successive cycles.[70] | Creation of cardiac MRI videos (pictured).[70] | ![]() |
Effective T2 or "T2-star" |
T2* | Postexcitation refocused GRE with small flip angle.[71] | Low signal from hemosiderin deposits (pictured) and hemorrhages.[71] | ![]() | |
Inversion recovery | Short tau inversion recovery | STIR | Fat suppression by setting an inversion time where the signal of fat is zero.[72] | High signal in edema, such as in more severe stress fracture.[73] Shin splints pictured: | ![]() |
Fluid-attenuated inversion recovery | FLAIR | Fluid suppression by setting an inversion time that nulls fluids | High signal in lacunar infarction, multiple sclerosis (MS) plaques, subarachnoid haemorrhage and meningitis (pictured).[74] | ![]() | |
Double inversion recovery | DIR | Simultaneous suppression of cerebrospinal fluid and white matter by two inversion times.[75] | High signal of multiple sclerosis plaques (pictured).[75] | ![]() | |
Diffusion weighted (DWI) | Conventional | DWI | Measure of Brownian motion of water molecules.[76] | High signal within minutes of cerebral infarction (pictured).[77] | ![]() |
Apparent diffusion coefficient | ADC | Reduced T2 weighting by taking multiple conventional DWI images with different DWI weighting, and the change corresponds to diffusion.[78] | Low signal minutes after cerebral infarction (pictured).[79] | ![]() | |
Diffusion tensor | DTI | Mainly tractography (pictured) by an overall greater Brownian motion of water molecules in the directions of nerve fibers.[80] |
|
![]() | |
Perfusion weighted (PWI) | Dynamic susceptibility contrast | DSC | Gadolinium contrast is injected, and rapid repeated imaging (generally gradient-echo echo-planar T2 weighted) quantifies susceptibility-induced signal loss.[82] | In cerebral infarction, the infarcted core and the penumbra have decreased perfusion (pictured).[83] | ![]() |
Dynamic contrast enhanced | DCE | Measuring shortening of the spin–lattice relaxation (T1) induced by a gadolinium contrast bolus.[84] | |||
Arterial spin labelling | ASL | Magnetic labeling of arterial blood below the imaging slab, which subsequently enters the region of interest.[85] It does not need gadolinium contrast.[86] | |||
Functional MRI (fMRI) | Blood-oxygen-level dependent imaging | BOLD | Changes in oxygen saturation-dependent magnetism of hemoglobin reflects tissue activity.[87] | Localizing highly active brain areas before surgery, also used in research of cognition.[88] | ![]() |
Magnetic resonance angiography (MRA) and venography | Time-of-flight | TOF | Blood entering the imaged area is not yet magnetically saturated, giving it a much higher signal when using short echo time and flow compensation. | Detection of aneurysm, stenosis, or dissection[89] | ![]() |
Phase-contrast magnetic resonance imaging | PC-MRA | Two gradients with equal magnitude, but opposite direction, are used to encode a phase shift, which is proportional to the velocity of spins.[90] | Detection of aneurysm, stenosis, or dissection (pictured).[89] | ![]() (VIPR) | |
Susceptibility-weighted | SWI | Sensitive for blood and calcium, by a fully flow compensated, long echo, gradient recalled echo (GRE) pulse sequence to exploit magnetic susceptibility differences between tissues | Detecting small amounts of hemorrhage (diffuse axonal injury pictured) or calcium.[91] | ![]() |
Other specialized configurations
Magnetic resonance spectroscopy
Magnetic resonance spectroscopy (MRS) is used to measure the levels of different metabolites in body tissues, which can be achieved through a variety of single voxel or imaging-based techniques.[92] The MR signal produces a spectrum of resonances that corresponds to different molecular arrangements of the isotope being "excited". This signature is used to diagnose certain metabolic disorders, especially those affecting the brain,[93] and to provide information on tumor metabolism.[94]
Magnetic resonance spectroscopic imaging (MRSI) combines both spectroscopic and imaging methods to produce spatially localized spectra from within the sample or patient. The spatial resolution is much lower (limited by the available SNR), but the spectra in each voxel contains information about many metabolites. Because the available signal is used to encode spatial and spectral information, MRSI requires high SNR achievable only at higher field strengths (3 T and above).[95] The high procurement and maintenance costs of MRI with extremely high field strengths[96] inhibit their popularity. However, recent compressed sensing-based software algorithms (e.g., SAMV[97]) have been proposed to achieve super-resolution without requiring such high field strengths.
Real-time MRI
File:Real-time MRI - Thorax.ogv
Real-time MRI refers to the continuous imaging of moving objects (such as the heart) in real time. One of the many different strategies developed since the early 2000s is based on radial FLASH MRI, and iterative reconstruction. This gives a temporal resolution of 20–30 ms for images with an in-plane resolution of 1.5–2.0 mm.[98] Balanced steady-state free precession (bSSFP) imaging has a better image contrast between the blood pool and myocardium than the FLASH MRI, yet it will produce severe banding artifact when the B0 inhomogeneity is strong. Real-time MRI is likely to add important information on diseases of the heart and the joints, and in many cases may make MRI examinations easier and more comfortable for patients, especially for the patients who cannot hold their breathings[99] or who have arrhythmia.
Interventional MRI
The lack of harmful effects on the patient and the operator make MRI well-suited for interventional radiology, where the images produced by an MRI scanner guide minimally invasive procedures. Such procedures use no ferromagnetic instruments.[100]
A specialized growing subset of interventional MRI is intraoperative MRI, in which an MRI is used in surgery. Some specialized MRI systems allow imaging concurrent with the surgical procedure. More typically, the surgical procedure is temporarily interrupted so that MRI can assess the success of the procedure or guide subsequent surgical work.[101]
Magnetic resonance guided focused ultrasound
In guided therapy, high-intensity focused ultrasound (HIFU) beams are focused on a tissue, that are controlled using MR thermal imaging. Due to the high energy at the focus, the temperature rises to above 65 °C (150 °F) which completely destroys the tissue. This technology can achieve precise ablation of diseased tissue. MR imaging provides a three-dimensional view of the target tissue, allowing for the precise focusing of ultrasound energy. The MR imaging provides quantitative, real-time, thermal images of the treated area. This allows the physician to ensure that the temperature generated during each cycle of ultrasound energy is sufficient to cause thermal ablation within the desired tissue and if not, to adapt the parameters to ensure effective treatment.[102]
Multinuclear imaging
Hydrogen has the most frequently imaged nucleus in MRI because it is present in biological tissues in great abundance, and because its high gyromagnetic ratio gives a strong signal. However, any nucleus with a net nuclear spin could potentially be imaged with MRI. Such nuclei include helium-3, lithium-7, carbon-13, fluorine-19, oxygen-17, sodium-23, phosphorus-31 and xenon-129. 23Na and 31P are naturally abundant in the body, so they can be imaged directly. Gaseous isotopes such as 3He or 129Xe must be hyperpolarized and then inhaled as their nuclear density is too low to yield a useful signal under normal conditions. 17O and 19F can be administered in sufficient quantities in liquid form (e.g. 17O-water) that hyperpolarization is not a necessity.[103] Using helium or xenon has the advantage of reduced background noise, and therefore increased contrast for the image itself, because these elements are not normally present in biological tissues.[104]
Moreover, the nucleus of any atom that has a net nuclear spin and that is bonded to a hydrogen atom could potentially be imaged via heteronuclear magnetization transfer MRI that would image the high-gyromagnetic-ratio hydrogen nucleus instead of the low-gyromagnetic-ratio nucleus that is bonded to the hydrogen atom.[105] In principle, heteronuclear magnetization transfer MRI could be used to detect the presence or absence of specific chemical bonds.[106][107]
Multinuclear imaging is primarily a research technique at present. However, potential applications include functional imaging and imaging of organs poorly seen on 1H MRI (e.g., lungs and bones) or as alternative contrast agents. Inhaled hyperpolarized 3He can be used to image the distribution of air spaces within the lungs. Injectable solutions containing 13C or stabilized bubbles of hyperpolarized 129Xe have been studied as contrast agents for angiography and perfusion imaging. 31P can potentially provide information on bone density and structure, as well as functional imaging of the brain. Multinuclear imaging holds the potential to chart the distribution of lithium in the human brain, this element finding use as an important drug for those with conditions such as bipolar disorder.[108]
Molecular imaging by MRI
MRI has the advantages of having very high spatial resolution and is very adept at morphological imaging and functional imaging. MRI does have several disadvantages though. First, MRI has a sensitivity of around 10−3 mol/L to 10−5 mol/L, which, compared to other types of imaging, can be very limiting. This problem stems from the fact that the population difference between the nuclear spin states is very small at room temperature. For example, at 1.5 teslas, a typical field strength for clinical MRI, the difference between high and low energy states is approximately 9 molecules per 2 million. Improvements to increase MR sensitivity include increasing magnetic field strength and hyperpolarization via optical pumping or dynamic nuclear polarization. There are also a variety of signal amplification schemes based on chemical exchange that increase sensitivity.[109]
To achieve molecular imaging of disease biomarkers using MRI, targeted MRI contrast agents with high specificity and high relaxivity (sensitivity) are required. To date, many studies have been devoted to developing targeted-MRI contrast agents to achieve molecular imaging by MRI. Commonly, peptides, antibodies, or small ligands, and small protein domains, such as HER-2 affibodies, have been applied to achieve targeting. To enhance the sensitivity of the contrast agents, these targeting moieties are usually linked to high payload MRI contrast agents or MRI contrast agents with high relaxivities.[110] A new class of gene targeting MR contrast agents has been introduced to show gene action of unique mRNA and gene transcription factor proteins.[111][112] These new contrast agents can trace cells with unique mRNA, microRNA and virus; tissue response to inflammation in living brains.[113] The MR reports change in gene expression with positive correlation to TaqMan analysis, optical and electron microscopy.[114]
Parallel MRI
It takes time to gather MRI data using sequential applications of magnetic field gradients. Even for the most streamlined of MRI sequences, there are physical and physiologic limits to the rate of gradient switching. Parallel MRI circumvents these limits by gathering some portion of the data simultaneously, rather than in a traditional sequential fashion. This is accomplished using arrays of radiofrequency (RF) detector coils, each with a different 'view' of the body. A reduced set of gradient steps is applied, and the remaining spatial information is filled in by combining signals from various coils, based on their known spatial sensitivity patterns. The resulting acceleration is limited by the number of coils and by the signal to noise ratio (which decreases with increasing acceleration), but two- to four-fold accelerations may commonly be achieved with suitable coil array configurations, and substantially higher accelerations have been demonstrated with specialized coil arrays. Parallel MRI may be used with most MRI sequences.
After a number of early suggestions for using arrays of detectors to accelerate imaging went largely unremarked in the MRI field, parallel imaging saw widespread development and application following the introduction of the SiMultaneous Acquisition of Spatial Harmonics (SMASH) technique in 1996–7.[115] The SENSitivity Encoding (SENSE)[116] and Generalized Autocalibrating Partially Parallel Acquisitions (GRAPPA)[117] techniques are the parallel imaging methods in most common use today. The advent of parallel MRI resulted in extensive research and development in image reconstruction and RF coil design, as well as in a rapid expansion of the number of receiver channels available on commercial MR systems. Parallel MRI is now used routinely for MRI examinations in a wide range of body areas and clinical or research applications.
Quantitative MRI
Most MRI focuses on qualitative interpretation of MR data by acquiring spatial maps of relative variations in signal strength which are "weighted" by certain parameters.[118] Quantitative methods instead attempt to determine spatial maps of accurate tissue relaxometry parameter values or magnetic field, or to measure the size of certain spatial features.
Examples of quantitative MRI methods are:
- T1-mapping (notably used in cardiac magnetic resonance imaging[119])
- T2-mapping[120]
- Quantitative susceptibility mapping (QSM)
- Quantitative fluid flow MRI (i.e. some cerebrospinal fluid flow MRI[121])
- Magnetic resonance elastography (MRE)[122]
Quantitative MRI aims to increase the reproducibility of MR images and interpretations, but has historically require longer scan times.[118]
Quantitative MRI (or qMRI) sometimes more specifically refers to multi-parametric quantitative MRI, the mapping of multiple tissue relaxometry parameters in a single imaging session.[123] Efforts to make multi-parametric quantitative MRI faster have produced sequences which map multiple parameters simultaneously, either by building separate encoding methods for each parameter into the sequence,[124] or by fitting MR signal evolution to a multi-parameter model.[125][126]
Hyperpolarized gas MRI
Traditional MRI generates poor images of lung tissue because there are fewer water molecules with protons that can be excited by the magnetic field. Using hyperpolarized gas an MRI scan can identify ventilation defects in the lungs. Before the scan, a patient is asked to inhale hyperpolarized xenon mixed with a buffer gas of helium or nitrogen. The resulting lung images are much higher quality than with tranditional MRI.
Safety
MRI is, in general, a safe technique, although injuries may occur as a result of failed safety procedures or human error.[127] Contraindications to MRI include most cochlear implants and cardiac pacemakers, shrapnel, and metallic foreign bodies in the eyes. Magnetic resonance imaging in pregnancy appears to be safe, at least during the second and third trimesters if done without contrast agents.[128] Since MRI does not use any ionizing radiation, its use is generally favored in preference to CT when either modality could yield the same information.[129] Some patients experience claustrophobia and may require sedation or shorter MRI protocols.[130][131] Amplitude and rapid switching of gradient coils during image acquisition may cause peripheral nerve stimulation.[132]
MRI uses powerful magnets and can therefore cause magnetic materials to move at great speeds, posing a projectile risk, and may cause fatal accidents.[133] However, as millions of MRIs are performed globally each year,[134] fatalities are extremely rare.[135]
MRI machines can produce loud noise, up to 120 dB(A).[136] This can cause hearing loss, tinnitus and hyperacusis, so appropriate hearing protection is essential for anyone inside the MRI scanner room during the examination.
Overuse
Medical societies issue guidelines for when physicians should use MRI on patients and recommend against overuse. MRI can detect health problems or confirm a diagnosis, but medical societies often recommend that MRI not be the first procedure for creating a plan to diagnose or manage a patient's complaint. A common case is to use MRI to seek a cause of low back pain; the American College of Physicians, for example, recommends against imaging (including MRI) as unlikely to result in a positive outcome for the patient.[25][26]
Artifacts
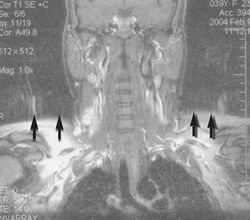
An MRI artifact is a visual artifact, that is, an anomaly during visual representation. Many different artifacts can occur during magnetic resonance imaging (MRI), some affecting the diagnostic quality, while others may be confused with pathology. Artifacts can be classified as patient-related, signal processing-dependent and hardware (machine)-related.[137]
Non-medical use
MRI is used industrially mainly for routine analysis of chemicals. The nuclear magnetic resonance technique is also used, for example, to measure the ratio between water and fat in foods, monitoring of flow of corrosive fluids in pipes, or to study molecular structures such as catalysts.[1]
Being non-invasive and non-damaging, MRI can be used to study the anatomy of plants, their water transportation processes and water balance.[138] It is also applied to veterinary radiology for diagnostic purposes. Outside this, its use in zoology is limited due to the high cost; but it can be used on many species.[139]
In palaeontology it is used to examine the structure of fossils.[140]
Forensic imaging provides graphic documentation of an autopsy, which manual autopsy does not. CT scanning provides quick whole-body imaging of skeletal and parenchymal alterations, whereas MR imaging gives better representation of soft tissue pathology.[141] All that being said, MRI is more expensive, and more time-consuming to utilize.[141] Moreover, the quality of MR imaging deteriorates below 10 °C.[142]
History
In 1971 at Stony Brook University, Paul Lauterbur applied magnetic field gradients in all three dimensions and a back-projection technique to create NMR images. He published the first images of two tubes of water in 1973 in the journal Nature,[143] followed by the picture of a living animal, a clam, and in 1974 by the image of the thoracic cavity of a mouse. Lauterbur called his imaging method zeugmatography, a term which was replaced by (N)MR imaging.[1] In the late 1970s, physicists Peter Mansfield and Paul Lauterbur developed MRI-related techniques, like the echo-planar imaging (EPI) technique.[144]
Raymond Damadian’s work into nuclear magnetic resonance (NMR) has been incorporated into MRI, having built one of the first scanners.[145]
Advances in semiconductor technology were crucial to the development of practical MRI, which requires a large amount of computational power. This was made possible by the rapidly increasing number of transistors on a single integrated circuit chip.[146] Mansfield and Lauterbur were awarded the 2003 Nobel Prize in Physiology or Medicine for their "discoveries concerning magnetic resonance imaging".[147]
See also
- Amplified magnetic resonance imaging
- Electron paramagnetic resonance
- High-definition fiber tracking
- High-resolution computed tomography
- History of neuroimaging
- International Society for Magnetic Resonance in Medicine
- Jemris
- List of neuroimaging software
- Magnetic immunoassay
- Magnetic particle imaging
- Magnetic resonance elastography
- Magnetic Resonance Imaging (journal)
- Magnetic resonance microscopy
- Nobel Prize controversies – Physiology or medicine
- Rabi cycle
- Robinson oscillator
- Sodium MRI
- Virtopsy
References
- ↑ 1.0 1.1 1.2 Rinck, Peter A. (2024). Magnetic Resonance in Medicine. A critical introduction. e-Textbook. (14th ed.). TRTF – The Round Table Foundation: TwinTree Media. "Magnetic Resonance in Medicine". http://magnetic-resonance.org/.
- ↑ MRI from Picture to Proton. Cambridge University Press. 2007. p. 1. ISBN 978-1-139-45719-4.
- ↑ 3.0 3.1 "NMR Signal Reception: Virtual Photons and Coherent Spontaneous Emission". Concepts in Magnetic Resonance 9 (5): 277–297. 1998. doi:10.1002/(SICI)1099-0534(1997)9:5<277::AID-CMR1>3.0.CO;2-W.
- ↑ [irrelevant citation]"Use of diagnostic imaging studies and associated radiation exposure for patients enrolled in large integrated health care systems, 1996-2010". JAMA 307 (22): 2400–9. June 2012. doi:10.1001/jama.2012.5960. PMID 22692172.
- ↑ Health at a glance 2009 OECD indicators. 2009. doi:10.1787/health_glance-2009-en. ISBN 978-92-64-07555-9.
- ↑ 6.0 6.1 MRI from picture to proton. Cambridge, UK; New York: Cambridge University Press. 2007. ISBN 978-0-521-68384-5.
- ↑ "Tesla Engineering Ltd - Magnet Division - MRI Supercon" (in en). http://www.tesla.co.uk/magnet/phone/tesla-engineering-magnet-division-superconducting-magnets-mobile/tesla-engineering-magnet-division-mri-magnets-mobile.html.
- ↑ Qiuliang, Wang (January 2022). "Successful Development of a 9.4T/800mm Whole-body MRI Superconducting Magnet at IEE CAS". https://snf.ieeecsc.org/sites/ieeecsc.org/files/documents/snf/abstracts/HP149-9.4T%20whole-body%20MRI%20superconducting%20magnet.pdf.
- ↑ Nowogrodzki, Anna (2018-10-31). "The world's strongest MRI machines are pushing human imaging to new limits" (in en). Nature 563 (7729): 24–26. doi:10.1038/d41586-018-07182-7. PMID 30382222. Bibcode: 2018Natur.563...24N. https://www.nature.com/articles/d41586-018-07182-7.
- ↑ CEA (2021-10-07). "The most powerful MRI scanner in the world delivers its first images!" (in en). https://www.cea.fr/english/Pages/News/premieres-images-irm-iseult-2021.aspx.
- ↑ Budinger, Thomas F.; Bird, Mark D. (2018-03-01). "MRI and MRS of the human brain at magnetic fields of 14T to 20T: Technical feasibility, safety, and neuroscience horizons" (in en). NeuroImage. Neuroimaging with Ultra-high Field MRI: Present and Future 168: 509–531. doi:10.1016/j.neuroimage.2017.01.067. ISSN 1053-8119. PMID 28179167.
- ↑ Li, Yi; Roell, Stefan (2021-12-01). "Key designs of a short-bore and cryogen-free high temperature superconducting magnet system for 14 T whole-body MRI". Superconductor Science and Technology 34 (12): 125005. doi:10.1088/1361-6668/ac2ec8. ISSN 0953-2048. Bibcode: 2021SuScT..34l5005L. https://iopscience.iop.org/article/10.1088/1361-6668/ac2ec8.
- ↑ "MR of the shoulder with a 0.2-T permanent-magnet unit". AJR. American Journal of Roentgenology 154 (4): 777–8. April 1990. doi:10.2214/ajr.154.4.2107675. PMID 2107675.
- ↑ "Guildford company gets FDA approval for bedside MRI". New Haven Register. 12 February 2020. https://www.ctinsider.com/business/nhregister/article/Guilford-company-gets-FDA-approval-for-bedside-MRI-15050860.php.
- ↑ "Microtesla MRI with a superconducting quantum interference device". Proceedings of the National Academy of Sciences of the United States of America 101 (21): 7857–61. May 2004. doi:10.1073/pnas.0402382101. PMID 15141077. Bibcode: 2004PNAS..101.7857M.
- ↑ "SQUID-based instrumentation for ultralow-field MRI". Superconductor Science and Technology 20 (11): S367–73. 2007. doi:10.1088/0953-2048/20/11/S13. Bibcode: 2007SuScT..20S.367Z.
- ↑ "Hybrid ultra-low-field MRI and magnetoencephalography system based on a commercial whole-head neuromagnetometer". Magnetic Resonance in Medicine 69 (6): 1795–804. June 2013. doi:10.1002/mrm.24413. PMID 22807201.
- ↑ De Leon-Rodriguez, L.M. (2015). "Basic MR Relaxation Mechanisms and Contrast Agent Design". Journal of Magnetic Resonance Imaging 42 (3): 545–565. doi:10.1002/jmri.24787. PMID 25975847.
- ↑ "Error: no
|title=
specified when using {{Cite web}}". http://imserc.northwestern.edu/downloads/nmr-t1.pdf. - ↑ McHale, J. (2017). Molecular Spectroscopy. CRC Press/Taylor and Francis Group. p. 73-80.
- ↑ 21.0 21.1 21.2 21.3 21.4 21.5 21.6 "Magnetic Resonance Imaging". University of Wisconsin. https://www.radiology.wisc.edu/education/med_students/neuroradiology/NeuroRad/Intro/MRIintro.htm.
- ↑ 22.00 22.01 22.02 22.03 22.04 22.05 22.06 22.07 22.08 22.09 22.10 22.11 22.12 22.13 "Basic proton MR imaging. Tissue Signal Characteristics". http://www.med.harvard.edu/aanlib/basicsMR.html.[unreliable medical source?]
- ↑ 23.0 23.1 "MRI sequences". 2013-01-18. http://www.slideshare.net/DrTusharPatil/mri-sequences.
- ↑ "Magnetic Resonance, a critical peer-reviewed introduction". European Magnetic Resonance Forum. http://www.magnetic-resonance.org/ch/21-01.html.
- ↑ 25.0 25.1 Consumer Reports; American College of Physicians. presented by ABIM Foundation. "Five Things Physicians and Patients Should Question". Choosing Wisely. http://choosingwisely.org/wp-content/uploads/2012/04/5things_12_factsheet_Amer_College_Phys.pdf. Retrieved August 14, 2012.
- ↑ 26.0 26.1 Consumer Reports; American College of Physicians (April 2012). "Imaging tests for lower-back pain: Why you probably don't need them". High Value Care. http://consumerhealthchoices.org/wp-content/uploads/2012/04/High-Value-Care-Back-Pain-ACP.pdf. Retrieved August 14, 2012.
- ↑ Recommendations for Cross-Sectional Imaging in Cancer Management: Computed Tomography – CT Magnetic Resonance Imaging – MRI Positron Emission Tomography – PET-CT. Royal College of Radiologists. 2008. ISBN 978-1-905034-13-0. http://www.rcr.ac.uk/docs/oncology/pdf/Cross_Sectional_Imaging_12.pdf. Retrieved 2014-05-29.
- ↑ "PEOPLE: PatiEnt prOstate samPLes for rEsearch, a tissue collection pathway utilizing magnetic resonance imaging data to target tumor and benign tissue in fresh radical prostatectomy specimens". The Prostate 79 (7): 768–777. May 2019. doi:10.1002/pros.23782. PMID 30807665.
- ↑ "Use of Magnetic Resonance Imaging and Biopsy Data to Guide Sampling Procedures for Prostate Cancer Biobanking". Journal of Visualized Experiments (152). October 2019. doi:10.3791/60216. PMID 31657791.
- ↑ American Society of Neuroradiology (2013). "ACR-ASNR Practice Guideline for the Performance and Interpretation of Magnetic Resonance Imaging (MRI) of the Brain". http://www.acr.org/~/media/ACR/Documents/PGTS/guidelines/MRI_Brain.pdf.
- ↑ "An improved MRI segmentation for atrophy assessment". International Journal of Computer Science Issues (IJCSI) 9 (3). May 2012.
- ↑ "Regional atrophy analysis of MRI for early detection of alzheimer's disease". International Journal of Signal Processing, Image Processing and Pattern Recognition 6 (1): 49–53. February 2013.
- ↑ Abnormal Psychology (Sixth ed.). New York: McGraw-Hill Education. 2014. p. 67.
- ↑ "The Invention and Early History of the N-Localizer for Stereotactic Neurosurgery". Cureus 8 (6): e642. June 2016. doi:10.7759/cureus.642. PMID 27462476.
- ↑ "Stereotaxis and nuclear magnetic resonance". Journal of Neurology, Neurosurgery, and Psychiatry 48 (1): 14–8. January 1985. doi:10.1136/jnnp.48.1.14. PMID 3882889.
- ↑ "Brown-Roberts-Wells stereotactic frame modifications to accomplish magnetic resonance imaging guidance in three planes". Applied Neurophysiology 50 (1–6): 143–52. 1987. doi:10.1159/000100700. PMID 3329837.
- ↑ Kanemaru, Noriko; Takao, Hidemasa; Amemiya, Shiori; Abe, Osamu (2 December 2021). "The effect of a post-scan processing denoising system on image quality and morphometric analysis". Journal of Neuroradiology 49 (2): 205–212. doi:10.1016/j.neurad.2021.11.007. PMID 34863809.
- ↑ "100-Hour-Long MRI of Human Brain Produces Most Detailed 3D Images Yet". 10 July 2019. https://www.sciencealert.com/100-hour-mri-marathon-gives-the-world-its-closest-ever-3d-view-of-the-human-brain.
- ↑ "Team publishes on highest resolution brain MRI scan". https://medicalxpress.com/news/2019-10-team-publishes-highest-resolution-brain.html.
- ↑ "Reference ranges for cardiac structure and function using cardiovascular magnetic resonance (CMR) in Caucasians from the UK Biobank population cohort". Journal of Cardiovascular Magnetic Resonance (Springer Science and Business Media LLC) 19 (1): 18. February 2017. doi:10.1186/s12968-017-0327-9. PMID 28178995.
- ↑ American College of Radiology; Society of Cardiovascular Computed Tomography; Society for Cardiovascular Magnetic Resonance; American Society of Nuclear Cardiology; North American Society for Cardiac Imaging; Society for Cardiovascular Angiography Interventions; Society of Interventional Radiology (October 2006). "ACCF/ACR/SCCT/SCMR/ASNC/NASCI/SCAI/SIR 2006 appropriateness criteria for cardiac computed tomography and cardiac magnetic resonance imaging. A report of the American College of Cardiology Foundation Quality Strategic Directions Committee Appropriateness Criteria Working Group". Journal of the American College of Radiology 3 (10): 751–71. doi:10.1016/j.jacr.2006.08.008. PMID 17412166.
- ↑ Musculoskeletal MRI. Saunders. 2008. ISBN 978-1-4160-5534-1.
- ↑ Aivazoglou, LU; Guimarães, JB; Link, TM; Costa, MAF; Cardoso, FN; de Mattos Lombardi Badia, B; Farias, IB; de Rezende Pinto, WBV et al. (21 April 2021). "MR imaging of inherited myopathies: a review and proposal of imaging algorithms.". European Radiology 31 (11): 8498–8512. doi:10.1007/s00330-021-07931-9. PMID 33881569.
- ↑ "Whole-body imaging of the musculoskeletal system: the value of MR imaging". Skeletal Radiology (Springer Nature) 36 (12): 1109–19. December 2007. doi:10.1007/s00256-007-0323-5. PMID 17554538.
- ↑ "Are Movement Artifacts in Magnetic Resonance Imaging a Real Problem?-A Narrative Review". Frontiers in Neurology 8: 232. 2017. doi:10.3389/fneur.2017.00232. PMID 28611728.
- ↑ Taber, K H; Herrick, R C; Weathers, S W; Kumar, A J; Schomer, D F; Hayman, L A (November 1998). "Pitfalls and artifacts encountered in clinical MR imaging of the spine." (in en). RadioGraphics 18 (6): 1499–1521. doi:10.1148/radiographics.18.6.9821197. ISSN 0271-5333. PMID 9821197. http://pubs.rsna.org/doi/10.1148/radiographics.18.6.9821197.
- ↑ "Hepatobiliary MR imaging with gadolinium-based contrast agents". Journal of Magnetic Resonance Imaging 35 (3): 492–511. March 2012. doi:10.1002/jmri.22833. PMID 22334493.
- ↑ "State-of-the-art pancreatic MRI". AJR. American Journal of Roentgenology 195 (1): 42–53. July 2010. doi:10.2214/ajr.195.3_supplement.0s42. PMID 20566796.
- ↑ "MR imaging of the small bowel". Radiology 264 (2): 333–48. August 2012. doi:10.1148/radiol.12111658. PMID 22821694.
- ↑ "Magnetic resonance (MR) colonography in the detection of colorectal lesions: a systematic review of prospective studies". European Radiology 20 (5): 1031–46. May 2010. doi:10.1007/s00330-009-1663-4. PMID 19936754.
- ↑ "Non-contrast enhanced MR angiography: physical principles". Journal of Magnetic Resonance Imaging (Wiley) 36 (2): 286–304. August 2012. doi:10.1002/jmri.23641. PMID 22807222.
- ↑ Magnetic resonance imaging: Physical principles and sequence design. New York: J. Wiley & Sons. 1999. ISBN 978-0-471-35128-3.[page needed]
- ↑ "Chapter 13: Contrast Agents". Magnetic Resonance in Medicine. 2014. http://www.magnetic-resonance.org/ch/13-01.html.
- ↑ "Adverse reactions to gadolinium contrast media: a review of 36 cases". AJR. American Journal of Roentgenology 167 (4): 847–9. October 1996. doi:10.2214/ajr.167.4.8819369. PMID 8819369.
- ↑ "ACR guideline". 2005. http://www.guideline.gov/summary/summary.aspx?doc_id=8283.
- ↑ Sergey Shugaev and Peter Caravan, Chapter 1: "Metal Ions in Bio-imaging Techniques: A Short Overview", pp 1-37 in "Metal Ions in Bio-Imaging Techniques" (2021). Editors: Astrid Sigel, Eva Freisinger and Roland K.O. Sigel. Publisher: Walter de Gruyter, Berlin.
de Gruyter.com/document/doi/10.1515/9783110685701-007 DOI 10.1515/9783110685701-007 - ↑ "FDA Drug Safety Communication: FDA warns that gadolinium-based contrast agents (GBCAs) are retained in the body; requires new class warnings". 2018-05-16. https://www.fda.gov/drugs/drug-safety-and-availability/fda-drug-safety-communication-fda-warns-gadolinium-based-contrast-agents-gbcas-are-retained-body.
- ↑ "Is there a causal relation between the administration of gadolinium based contrast media and the development of nephrogenic systemic fibrosis (NSF)?". Clinical Radiology 61 (11): 905–6. November 2006. doi:10.1016/j.crad.2006.09.003. PMID 17018301.
- ↑ "FDA Drug Safety Communication: New warnings for using gadolinium-based contrast agents in patients with kidney dysfunction". U.S. Food and Drug Administration. 23 December 2010. https://www.fda.gov/Drugs/DrugSafety/ucm223966.htm.
- ↑ "FDA Public Health Advisory: Gadolinium-containing Contrast Agents for Magnetic Resonance Imaging". https://www.fda.gov/cder/drug/advisory/gadolinium_agents.htm.
- ↑ "Gadolinium-containing contrast agents: new advice to minimise the risk of nephrogenic systemic fibrosis". Drug Safety Update 3 (6): 3. January 2010. https://www.gov.uk/drug-safety-update/gadolinium-containing-contrast-agents-new-advice-to-minimise-the-risk-of-nephrogenic-systemic-fibrosis.
- ↑ "MRI Questions and Answers". Concord, CA: International Society for Magnetic Resonance in Medicine. http://www.ismrm.org/special/EMEA2.pdf.
- ↑ "Response to the FDA's May 23, 2007, Nephrogenic Systemic Fibrosis Update1 — Radiology". Radiological Society of North America. 2007-09-12. http://radiology.rsna.org/content/246/1/11.full?searchid=1&HITS=10&hits=10&sortspec=relevance&resourcetype=HWCIT&maxtoshow=&RESULTFORMAT=&author1=kanal&FIRSTINDEX=0.
- ↑ "MRI sequences (overview)". https://radiopaedia.org/articles/mri-sequences-overview.
- ↑ 65.0 65.1 65.2 65.3 "Magnetic Resonance Imaging". University of Wisconsin. https://www.radiology.wisc.edu/education/med_students/neuroradiology/NeuroRad/Intro/MRIintro.htm. Retrieved 2016-03-14.
- ↑ 66.0 66.1 66.2 66.3 Johnson, Keith A.. "Basic proton MR imaging. Tissue Signal Characteristics". Harvard Medical School. Archived from the original on 2016-03-05. https://web.archive.org/web/20160305000107/http://www.med.harvard.edu/aanlib/basicsMR.html. Retrieved 2016-03-14.
- ↑ Principles and Applications of Radiological Physics E-Book (6 ed.). Elsevier Health Sciences. 2011-05-31. p. 292. ISBN 978-0-7020-4614-8.}
- ↑ "MRI sequences (overview)". https://radiopaedia.org/articles/mri-sequences-overview. Retrieved 2017-01-13.
- ↑ "A Current Review of the Meniscus Imaging: Proposition of a Useful Tool for Its Radiologic Analysis". Radiology Research and Practice 2016: 8329296. 2016. doi:10.1155/2016/8329296. PMID 27057352.
- ↑ 70.0 70.1 "Steady-state free precession MRI". https://radiopaedia.org/articles/steady-state-free-precession-mri-2. Retrieved 2017-10-13.
- ↑ 71.0 71.1 "Principles, techniques, and applications of T2*-based MR imaging and its special applications". Radiographics 29 (5): 1433–49. 2009. doi:10.1148/rg.295095034. PMID 19755604.
- ↑ "Short tau inversion recovery". https://radiopaedia.org/articles/short-tau-inversion-recovery. Retrieved 2017-10-13.
- ↑ "Stress fractures". Radiology Society of the Netherlands. http://www.radiologyassistant.nl/en/p4615feaee7e0a/stress-fractures.html. Retrieved 2017-10-13.
- ↑ "Fluid attenuation inversion recoveryg". http://radiopaedia.org/articles/fluid-attenuation-inversion-recovery. Retrieved 2015-12-03.
- ↑ 75.0 75.1 "Double inversion recovery sequence". https://radiopaedia.org/articles/double-inversion-recovery-sequence. Retrieved 2017-10-13.
- ↑ "Diffusion weighted imaging". https://radiopaedia.org/articles/diffusion-weighted-imaging-1. Retrieved 2017-10-13.
- ↑ "Ischaemic stroke". https://radiopaedia.org/articles/ischaemic-stroke. Retrieved 2017-10-15.
- ↑ Hammer, Mark. "MRI Physics: Diffusion-Weighted Imaging". http://xrayphysics.com/dwi.html#adc. Retrieved 2017-10-15.
- ↑ "Signal evolution and infarction risk for apparent diffusion coefficient lesions in acute ischemic stroke are both time- and perfusion-dependent". Stroke 42 (5): 1276–81. May 2011. doi:10.1161/STROKEAHA.110.610501. PMID 21454821.
- ↑ 80.0 80.1 "Diffusion tensor imaging". https://radiopaedia.org/articles/diffusion-tensor-imaging. Retrieved 2017-10-13.
- ↑ "Diffusion tensor imaging in mild cognitive impairment and Alzheimer's disease: a review". Current Opinion in Neurology 21 (1): 83–92. February 2008. doi:10.1097/WCO.0b013e3282f4594b. PMID 18180656.
- ↑ "Dynamic susceptibility contrast (DSC) MR perfusion". https://radiopaedia.org/articles/dynamic-susceptibility-contrast-dsc-mr-perfusion. Retrieved 2017-10-14.
- ↑ "Magnetic resonance diffusion-perfusion mismatch in acute ischemic stroke: An update". World Journal of Radiology 4 (3): 63–74. March 2012. doi:10.4329/wjr.v4.i3.63. PMID 22468186.
- ↑ "Dynamic contrast enhanced (DCE) MR perfusion". https://radiopaedia.org/articles/dynamic-contrast-enhanced-dce-mr-perfusion-1. Retrieved 2017-10-15.
- ↑ "Arterial spin labeling". http://fmri.research.umich.edu/research/main_topics/asl.php. Retrieved 2017-10-27.
- ↑ "Arterial spin labelling (ASL) MR perfusion". https://radiopaedia.org/articles/arterial-spin-labelling-asl-mr-perfusion-1. Retrieved 2017-10-15.
- ↑ Chou, I-han. "Milestone 19: (1990) Functional MRI". Nature. http://www.nature.com/milestones/milespin/full/milespin19.html. Retrieved 9 August 2013.
- ↑ "Functional MRI". https://radiopaedia.org/articles/functional-mri. Retrieved 2017-10-16.
- ↑ 89.0 89.1 "Magnetic Resonance Angiography (MRA)". http://www.hopkinsmedicine.org/healthlibrary/test_procedures/cardiovascular/magnetic_resonance_angiography_mra_135,14. Retrieved 2017-10-15.
- ↑ "Phase contrast imaging". https://radiopaedia.org/articles/phase-contrast-imaging. Retrieved 2017-10-15.
- ↑ "Susceptibility weighted imaging". https://radiopaedia.org/articles/susceptibility-weighted-imaging-1. Retrieved 2017-10-15.
- ↑ "Theoretical description of modern 1 H in Vivo magnetic resonance spectroscopic pulse sequences". Journal of Magnetic Resonance Imaging 51 (4): 1008–1029. April 2020. doi:10.1002/jmri.26846. PMID 31273880.
- ↑ "Recent advances in magnetic resonance neurospectroscopy". Neurotherapeutics 4 (3): 330–45. July 2007. doi:10.1016/j.nurt.2007.04.009. PMID 17599700.
- ↑ "Magnetic resonance spectroscopy in clinical oncology". Onkologie 27 (3): 304–9. June 2004. doi:10.1159/000077983. PMID 15249722.
- ↑ "Comparison of 1.5 and 8 tesla high-resolution magnetic resonance imaging of lacunar infarcts". Journal of Computer Assisted Tomography 26 (4): 628–32. 2002. doi:10.1097/00004728-200207000-00027. PMID 12218832.
- ↑ "MRI-scanner van 7 miljoen in gebruik" (in nl). Medisch Contact. December 5, 2007. https://www.medischcontact.nl/nieuws/laatste-nieuws/artikel/mri-scanner-van-7-miljoen-in-gebruik.htm.
- ↑ "Iterative Sparse Asymptotic Minimum Variance Based Approaches for Array Processing". IEEE Transactions on Signal Processing 61 (4): 933–44. 2013. doi:10.1109/tsp.2012.2231676. Bibcode: 2013ITSP...61..933A.
- ↑ "Real-time MRI at a resolution of 20 ms". NMR in Biomedicine 23 (8): 986–94. October 2010. doi:10.1002/nbm.1585. PMID 20799371.
- ↑ "Applying a Level Set Method for Resolving Physiologic Motions in Free-Breathing and Non-gated Cardiac MRI". Functional Imaging and Modeling of the Heart. Lecture Notes in Computer Science. 7945. 2013. pp. 466–473. doi:10.1007/978-3-642-38899-6_55. ISBN 978-3-642-38898-9.
- ↑ "Interventional MR imaging: concepts, systems, and applications in neuroradiology". AJNR. American Journal of Neuroradiology 20 (5): 735–48. May 1999. PMID 10369339. PMC 7056143. http://www.ajnr.org/content/20/5/735.
- ↑ The Gale Encyclopedia of Nursing and Allied Health (3rd ed.). Farmington, MI: Gale. 2013. ISBN 9781414498881. https://archive.org/details/galeencyclopedia0001unse_r6b8.
- ↑ "MR-guided focused ultrasound surgery". Journal of Computer Assisted Tomography 16 (6): 956–65. 1992. doi:10.1097/00004728-199211000-00024. PMID 1430448.
- ↑ "Molecular imaging without radiopharmaceuticals?". Journal of Nuclear Medicine (Society of Nuclear Medicine) 50 (6): 999–1007. June 2009. doi:10.2967/jnumed.108.059576. PMID 19443583.
- ↑ "Hyperpolarized Noble Gas MRI Laboratory: Hyperpolarized Xenon MR Imaging of the Brain". Harvard Medical School. http://www.spl.harvard.edu/archive/HypX/currentresult0.html.
- ↑ "Gradient-enhanced proton-detected heteronuclear multiple-quantum coherence spectroscopy". Journal of Magnetic Resonance 91 (3): 648–53. 1991. doi:10.1016/0022-2364(91)90395-a. Bibcode: 1991JMagR..91..648H.
- ↑ "A Test for Scaler Coupling between Heteronuclei Using Gradient-Enhanced Proton-Detected HMQC Spectroscopy". Journal of Magnetic Resonance, Series A 113 (1): 117–19. 1995. doi:10.1006/jmra.1995.1064. Bibcode: 1995JMagR.113..117B.
- ↑ "Measurement of the degree of coupled isotopic enrichment of different positions in an antibiotic peptide by NMR". Journal of Magnetic Resonance 125 (1): 120–31. March 1997. doi:10.1006/jmre.1997.1107. PMID 9245367. Bibcode: 1997JMagR.125..120M.
- ↑ "White matter microstructural properties in bipolar disorder in relationship to the spatial distribution of lithium in the brain". Journal of Affective Disorders 253: 224–231. June 2019. doi:10.1016/j.jad.2019.04.075. PMID 31054448.
- ↑ "An introduction to functional and molecular imaging with MRI". Clinical Radiology 65 (7): 557–66. July 2010. doi:10.1016/j.crad.2010.04.006. PMID 20541655.
- ↑ "Design of a novel class of protein-based magnetic resonance imaging contrast agents for the molecular imaging of cancer biomarkers". Wiley Interdisciplinary Reviews. Nanomedicine and Nanobiotechnology 5 (2): 163–79. 2013. doi:10.1002/wnan.1205. PMID 23335551.
- ↑ "Imaging cerebral gene transcripts in live animals". The Journal of Neuroscience 27 (3): 713–22. January 2007. doi:10.1523/JNEUROSCI.4660-06.2007. PMID 17234603.
- ↑ "Intracellular gene transcription factor protein-guided MRI by DNA aptamers in vivo". FASEB Journal 28 (1): 464–73. January 2014. doi:10.1096/fj.13-234229. PMID 24115049.
- ↑ "Diffusion-weighted magnetic resonance imaging reversal by gene knockdown of matrix metalloproteinase-9 activities in live animal brains". The Journal of Neuroscience 29 (11): 3508–17. March 2009. doi:10.1523/JNEUROSCI.5332-08.2009. PMID 19295156.
- ↑ "MRI reveals differential effects of amphetamine exposure on neuroglia in vivo". FASEB Journal 27 (2): 712–24. February 2013. doi:10.1096/fj.12-220061. PMID 23150521.
- ↑ "Simultaneous acquisition of spatial harmonics (SMASH): fast imaging with radiofrequency coil arrays". Magnetic Resonance in Medicine 38 (4): 591–603. October 1997. doi:10.1002/mrm.1910380414. PMID 9324327. https://doi.org/10.1002/mrm.1910380414.
- ↑ "SENSE: sensitivity encoding for fast MRI". Magnetic Resonance in Medicine 42 (5): 952–62. November 1999. doi:10.1002/(SICI)1522-2594(199911)42:5<952::AID-MRM16>3.0.CO;2-S. PMID 10542355.
- ↑ "Generalized autocalibrating partially parallel acquisitions (GRAPPA)". Magnetic Resonance in Medicine 47 (6): 1202–10. June 2002. doi:10.1002/mrm.10171. PMID 12111967.
- ↑ 118.0 118.1 Gulani, Vikas; Nicole, Sieberlich (2020). "Quantitative MRI: Rationale and Challenges". Quantitative Magnetic Resonance Imaging. Academic Press. p. xxxvii-li. doi:10.1016/B978-0-12-817057-1.00001-9. ISBN 9780128170571.
- ↑ Captur, G; Manisty, C; Moon, JC (2016). "Cardiac MRI evaluation of myocardial disease.". Heart 102 (18): 1429–35. doi:10.1136/heartjnl-2015-309077. PMID 27354273. https://pubmed.ncbi.nlm.nih.gov/27354273.
- ↑ Cobianchi Bellisari, F et al. (2021). "T2-mapping MRI evaluation of patellofemoral cartilage in patients submitted to intra-articular platelet-rich plasma (PRP) injections.". Radiol Med 126 (8): 1085–1094. doi:10.1007/s11547-021-01372-6. PMID 34008045.
- ↑ Gaillard, Frank; Knipe, Henry (13 Oct 2021) (in en-US). CSF flow studies | Radiology Reference Article. doi:10.53347/rID-37401. https://radiopaedia.org/articles/csf-flow-studies?lang=us. Retrieved 2021-11-24.
- ↑ Hirsch, Sebastian; Braun, Jürgen; Sack, Ingolf (2016). Magnetic Resonance Elastography | Wiley Online Books. doi:10.1002/9783527696017. ISBN 9783527696017. https://www.onlinelibrary.wiley.com/doi/book/10.1002/9783527696017. Retrieved 2022-03-06.
- ↑ Seiler A, Nöth U, Hok P, Reiländer A, Maiworm M, Baudrexel S (2021). "Multiparametric Quantitative MRI in Neurological Diseases.". Front Neurol 12: 640239. doi:10.3389/fneur.2021.640239. PMID 33763021.
- ↑ Warntjes JB, Leinhard OD, West J, Lundberg P (2008). "Rapid magnetic resonance quantification on the brain: Optimization for clinical usage.". Magn Reson Med 60 (2): 320–9. doi:10.1002/mrm.21635. PMID 18666127.
- ↑ Ehses P, Seiberlich N, Ma D, Breuer FA, Jakob PM, Griswold MA (2013). "IR TrueFISP with a golden-ratio-based radial readout: fast quantification of T1, T2, and proton density.". Magn Reson Med 69 (1): 71–81. doi:10.1002/mrm.24225. PMID 22378141.
- ↑ Ma D, Gulani V, Seiberlich N, Liu K, Sunshine JL, Duerk JL (2013). "Magnetic resonance fingerprinting.". Nature 495 (7440): 187–92. doi:10.1038/nature11971. PMID 23486058. Bibcode: 2013Natur.495..187M.
- ↑ "Lessons Learned from MRI Safety Events". Current Radiology Reports 3 (10). 2015. doi:10.1007/s40134-015-0122-z.
- ↑ "MRI in pregnancy: Indications and practical considerations". Journal of Magnetic Resonance Imaging 49 (3): 621–631. March 2019. doi:10.1002/jmri.26317. PMID 30701610.
- ↑ "iRefer". Royal College of Radiologists. http://www.rcr.ac.uk/content.aspx?PageID=995.
- ↑ "Adult claustrophobia, anxiety and sedation in MRI". Magnetic Resonance Imaging (Elsevier BV) 15 (1): 51–4. 1997. doi:10.1016/s0730-725x(96)00351-7. PMID 9084025.
- ↑ Shahrouki, Puja; Nguyen, Kim-Lien; Moriarty, John M.; Plotnik, Adam N.; Yoshida, Takegawa; Finn, J. Paul (2021-09-01). "Minimizing table time in patients with claustrophobia using focused ferumoxytol-enhanced MR angiography ( f -FEMRA): a feasibility study" (in en). The British Journal of Radiology 94 (1125): 20210430. doi:10.1259/bjr.20210430. ISSN 0007-1285. PMID 34415199.
- ↑ "Investigating cardiac stimulation limits of MRI gradient coils using electromagnetic and electrophysiological simulations in human and canine body models". Magnetic Resonance in Medicine 85 (2): 1047–1061. February 2021. doi:10.1002/mrm.28472. PMID 32812280.
- ↑ Agence France-Presse (30 January 2018). "Man dies after being sucked into MRI scanner at Indian hospital". The Guardian. https://www.theguardian.com/world/2018/jan/30/mri-scanner-india-death.
- ↑ "Magnetic Resonance Imaging (MRI) Exams per 1,000 Population, 2014". OECD. 2016. https://international.commonwealthfund.org/stats/mri_exams/.
- ↑ "Rates of safety incident reporting in MRI in a large academic medical center". Journal of Magnetic Resonance Imaging (John Wiley and Sons) 43 (4): 998–1007. April 2016. doi:10.1002/jmri.25055. PMID 26483127.
- ↑ Price, D. L.; De Wilde, J. P.; Papadaki, A. M.; Curran, J. S.; Kitney, R. I. (February 2001). "Investigation of acoustic noise on 15 MRI scanners from 0.2 T to 3 T". Journal of Magnetic Resonance Imaging: JMRI 13 (2): 288–293. doi:10.1002/1522-2586(200102)13:2<288::aid-jmri1041>3.0.co;2-p. ISSN 1053-1807. PMID 11169836.
- ↑ 137.0 137.1 "A short overview of MRI artefacts". South African Journal of Radiology 8 (2): 13. 2004. doi:10.4102/sajr.v8i2.127.
- ↑ "Intact plant MRI for the study of cell water relations, membrane permeability, cell-to-cell and long distance water transport". Journal of Experimental Botany (Oxford University Press (OUP)) 58 (4): 743–56. 2006-11-30. doi:10.1093/jxb/erl157. PMID 17175554.
- ↑ "Application of magnetic resonance imaging in zoology". Zoomorphology (Springer Science and Business Media LLC) 130 (4): 227–254. 2011-10-13. doi:10.1007/s00435-011-0138-8. ISSN 0720-213X.
- ↑ "Magnetic resonance spectroscopy and imaging for the study of fossils". Magnetic Resonance Imaging (Elsevier BV) 34 (6): 730–742. July 2016. doi:10.1016/j.mri.2016.03.010. PMID 26979538.
- ↑ 141.0 141.1 "A Practical Guide to Virtual Autopsy: Why, When and How". Seminars in Ultrasound, CT, and MR 40 (1): 56–66. February 2019. doi:10.1053/j.sult.2018.10.011. PMID 30686369.
- ↑ "Essentials of forensic post-mortem MR imaging in adults". The British Journal of Radiology 87 (1036): 20130567. April 2014. doi:10.1259/bjr.20130567. PMID 24191122.
- ↑ LAUTERBUR, P. C. (1973). "Image Formation by Induced Local Interactions: Examples Employing Nuclear Magnetic Resonance". Nature (Springer Science and Business Media LLC) 242 (5394): 190–191. doi:10.1038/242190a0. ISSN 0028-0836. Bibcode: 1973Natur.242..190L.
- ↑ ""Diffraction" and microscopy in solids and liquids by NMR". Physical Review B 12 (9): 3618–34. 1975. doi:10.1103/physrevb.12.3618. Bibcode: 1975PhRvB..12.3618M.
- ↑ Sandomir, Richard (August 17, 2022). "Raymond Damadian, Creator of the First M.R.I. Scanner, Dies at 86". The New York Times. https://www.nytimes.com/2022/08/17/science/raymond-damadian-dead.html.
- ↑ Quantum Enigma: Physics Encounters Consciousness. Oxford University Press. 2011. p. 127. ISBN 9780199792955. https://books.google.com/books?id=I9kGFX1_oGAC&pg=PT127.
- ↑ "The Nobel Prize in Physiology or Medicine 2003". Nobel Foundation. http://www.nobelprize.org/nobel_prizes/medicine/laureates/2003/index.html.
Further reading
- Blümer, Peter (1998). Spatially Resolved Magnetic Resonance: Methods, Materials, Medicine, Biology, Rheology, Geology, Ecology, Hardware. Wiley-VCH. ISBN 978-3-527-29637-8.
- Blümich, Bernhard; Kuhn, Winfried (1992). Magnetic Resonance Microscopy: Methods and Applications in Materials Science, Agriculture and Biomedicine. Wiley. ISBN 978-3-527-28403-0.
- Blümich, Bernhard (2000). NMR Imaging of Materials. Clarendon Press. ISBN 978-0-19-850683-6.
- "Whole body magnetic resonance imaging". BMJ 328 (7453): 1387–8. June 2004. doi:10.1136/bmj.328.7453.1387. PMID 15191954.
- Farhat, Imad Akil; Belton, P.S.; Webb, Graham Alan (2007). Magnetic Resonance in Food Science: From Molecules to Man. Royal Society of Chemistry. ISBN 978-0-85404-340-8.
- Fukushima, Eiichi (1989). NMR in Biomedicine: The Physical Basis. Springer Science & Business Media. ISBN 978-0-88318-609-1.
- Haacke, E Mark; Brown, Robert F; Thompson, Michael; Venkatesan, Ramesh (1999). Magnetic resonance imaging: Physical principles and sequence design. New York: J. Wiley & Sons. ISBN 978-0-471-35128-3.
- Jin (1998). Electromagnetic Analysis and Design in Magnetic Resonance Imaging. CRC Press. ISBN 978-0-8493-9693-9.
- Kuperman, Vadim (2000). Magnetic Resonance Imaging: Physical Principles and Applications. Academic Press. ISBN 978-0-08-053570-8.
- "One micrometer resolution NMR microscopy". Journal of Magnetic Resonance 150 (2): 207–13. June 2001. doi:10.1006/jmre.2001.2319. PMID 11384182. Bibcode: 2001JMagR.150..207L.
- Liang, Zhi-Pei; Lauterbur, Paul C. (1999). Principles of Magnetic Resonance Imaging: A Signal Processing Perspective. Wiley. ISBN 978-0-7803-4723-6. https://archive.org/details/principlesofmagn00zhip.
- NMR Imaging in Biomedicine: Supplement 2 Advances in Magnetic Resonance. Elsevier. 1982. ISBN 978-0-323-15406-2.
- "NMR imaging in medicine". Scientific American 246 (5): 78–88. May 1982. doi:10.1038/scientificamerican0582-78. PMID 7079720. Bibcode: 1982SciAm.246e..78P.
- "The history of MRI". TRTF/EMRF. http://www.magnetic-resonance.org/ch/20-01.html.
- Sakr, HM; Fahmy, N; Elsayed, NS; Abdulhady, H; El-Sobky, TA; Saadawy, AM; Beroud, C; Udd, B (1 July 2021). "Whole-body muscle MRI characteristics of LAMA2-related congenital muscular dystrophy children: An emerging pattern.". Neuromuscular Disorders 31 (9): 814–823. doi:10.1016/j.nmd.2021.06.012. PMID 34481707.
- Schmitt, Franz; Stehling, Michael K.; Turner, Robert (1998). Echo-Planar Imaging: Theory, Technique and Application. Springer Berlin Heidelberg. ISBN 978-3-540-63194-1. https://archive.org/details/springer_10.1007-978-3-642-80443-4.
- Simon, Merrill; Mattson, James S (1996). The pioneers of NMR and magnetic resonance in medicine: The story of MRI. Ramat Gan, Israel: Bar-Ilan University Press. ISBN 978-0-9619243-1-7. https://archive.org/details/pioneersofnmrmag0000matt.
- Sprawls, Perry (2000). Magnetic Resonance Imaging: Principles, Methods, and Techniques. Medical Physics Publishing. ISBN 978-0-944838-97-6. http://www.sprawls.org/mripmt/index.html.
External links
- "MRI: A Peer-Reviewed, Critical Introduction". European Magnetic Resonance Forum (EMRF)/The Round Table Foundation (TRTF). http://www.magnetic-resonance.org.
- A Guided Tour of MRI: An introduction for laypeople National High Magnetic Field Laboratory
- The Basics of MRI. Underlying physics and technical aspects.
- Video: What to Expect During Your MRI Exam from the Institute for Magnetic Resonance Safety, Education, and Research (IMRSER)
- Royal Institution Lecture – MRI: A Window on the Human Body
- A Short History of Magnetic Resonance Imaging from a European Point of View
- How MRI works explained simply using diagrams
- Real-time MRI videos: Biomedizinische NMR Forschungs GmbH.
- Paul C. Lauterbur, Genesis of the MRI (Magnetic Resonance Imaging) notebook, September 1971 (all pages freely available for download in variety of formats from Science History Institute Digital Collections at digital.sciencehistory.org)
![]() | Original source: https://en.wikipedia.org/wiki/Magnetic resonance imaging.
Read more |