Biology:Endophyte
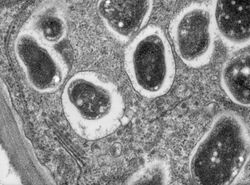
An endophyte is an endosymbiont, often a bacterium or fungus, that lives within a plant for at least part of its life cycle without causing apparent disease. Endophytes are ubiquitous and have been found in all species of plants studied to date; however, most of the endophyte/plant relationships are not well understood. Some endophytes may enhance host growth, nutrient acquisition and improve the plant's ability to tolerate abiotic stresses, such as drought and decrease biotic stresses by enhancing plant resistance to insects, pathogens and herbivores. Although endophytic bacteria and fungi are frequently studied, endophytic archaea are increasingly being considered for their role in plant growth promotion as part of the core microbiome of a plant. [1]
History
Endophytes were first described by the German botanist Johann Heinrich Friedrich Link in 1809. They were thought to be plant parasitic fungi and they were later termed as "microzymas" by the French scientist Béchamp. There was a belief that plants were healthy under sterile conditions and it was not until 1887 that Victor Galippe discovered bacteria normally occurring inside plant tissues.[2] Though, most of the endophytic studies reports the mutualistic relationship of bacteria and fungus, Das et al., (2019) reported about endophytic virome and their probable function in plant defense mechanisms.[3]
Transmission
Endophytes may be transmitted either vertically (directly from parent to offspring) or horizontally (among individuals).[4] Vertically transmitted fungal endophytes are typically considered clonal and transmit via fungal hyphae penetrating the embryo within the host's seeds, while reproduction of the fungi through asexual conidia or sexual spores leads to horizontal transmission, where endophytes may spread between plants in a population or community.[5]
Symbiosis
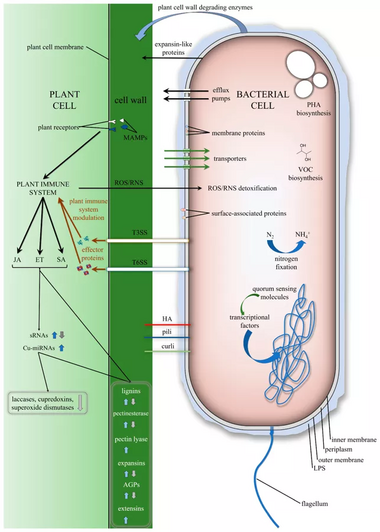
Most endophyte-plant relationships are still not well understood.[7] However, recently it was shown that endophytes are transmitted from one generation to another via seeds, in a process called vertical transmission.[8] Endophytes and plants often engage in mutualism, with endophytes primarily aiding in the health and survival of the host plant with issues such as pathogens and disease,[9] water stress, heat stress, nutrient availability and poor soil quality, salinity, and herbivory.[2] In exchange the endophyte receives carbon for energy from the plant host. Plant-microbe interactions are not strictly mutualistic, as endophytic fungi can potentially become pathogens or saprotrophs, usually when the plant is stressed.[10] Endophytes may become active and reproduce under specific environmental conditions or when their host plants are stressed or begin to senesce, thereby limiting the amount of carbon provided to the endophyte.[11][12]
Endophytes may benefit host plants by preventing other pathogenic or parasitic organisms from colonizing them. Endophytes can extensively colonize plant tissues and competitively exclude other potential pathogens.[13][14] Some fungal and bacterial endophytes have proven to increase plant growth and improve overall plant hardiness.[15]
Studies have shown that endophytic fungi grow in a very intimate interaction with their host plant cells. Fungal hyphae have been seen growing either flattened or wedged against plant cells. This growth pattern indicates that fungal hyphae are substantially attached to the plant host's cell wall, but do not invade plant cells.[16] Endophytic fungal hyphae appear to grow at the same rate as their host leaves, within the intercellular spaces of the plant tissue.[17]
The presence of certain fungal endophytes in host meristems, leaves and reproductive structures has been shown to dramatically enhance the survival of their hosts. This enhanced survivability is largely attributed to endophytic production of secondary metabolites which protect against herbivory as well as increased uptake of nutrients.[16] Studies have also shown that during experimental circumstances endophytes contribute significantly to plant growth and fitness under light-limited conditions, and plants appear to have increased reliance on their endophytic symbiont under these conditions.[18]
There is evidence that plants and endophytes engage in communication with each other that can aid symbiosis. For example, plant chemical signals have been shown to activate gene expression in endophytes. One example of this plant-endosymbiont interaction occurs between dicotyledonous plants in the Convolvulaceae and clavicipitaceous fungi. When the fungus is in the plant it synthesizes ergoline alkaloids at a higher rate, compared to when it is grown apart from the plant. This supports the hypothesis that plant signaling is required in order to induce expression of endophytic secondary metabolites.[19]
Effects on plant behavior
There are various behaviors that have been studied that resulted from endophyte symbiosis with plants. Through association with fungal endophytes, the root and shoot structures of Pseudotsuga menziesii (Douglas-fir) saplings in low-nutrient conditions have been shown to be elongated, as well as undergo overall biomass increases.[20] The proposed mechanisms behind this include high inorganic phosphate solubilization ability by the fungi as well as organic phosphate mineralization, increased mycorrhizal associations through root colonization, and enhanced nitrogen and phosphorus uptake.[20] Specific endophyte species can also stimulate root growth by increasing the flux of auxin to where the endophyte is.[21]
Additionally, various reports on endophyte interactions have shown increased photosynthetic capacities of host plants as well as improved water relations.[22] Improvements in water use efficiency were observed in higher CO
2 concentrations and a further increase was seen in water deficit conditions.[22] In addition, other various physiological pathways were activated upon endophytes interactions with host plants, enabling tighter water control and further water management, which are to be the main reasons behind improved water relations.[22] Specifically, evidence points to endophytes producing ABA to affect stomatal conductance as well as microbial respiration and plants recycling CO
2.[23]
However, the specific biochemical mechanisms behind these behavioral changes are still largely unknown and lower-level signal cascades have yet to be discovered. Furthermore, while the benefits of endophyte relations are well-studied, the costs of these relations are less well understood, such as the specific carbon costs, the system of endophyte governance, and the environmental conditions that facilitate a proper plant-endophyte relationship.[22]
In an experiment investigating the interaction between Miscanthus sinensis and the plant endophyte Herbaspirillum frisingense, a roughly 20% increase in fresh biomass was observed in M. sinensis following inoculation with H. frisingense.[24] However, unique to this experiment was the mode by which this was thought to happen. Inoculation saw an upregulation in the genes relevant to jasmonate and ethylene production in the plant roots, although the mechanism to this is still unknown.[24] Specifically, H. frisingense was shown to upregulate ethylene receptors and repress ethylene response factors, overall leading to an increase in root growth.[24] Additionally, H. frisingense is known to produce indoleacetic acid (IAA),[25] and was also shown to manage IAA genes, indicating that there is an intricate balance maintained between ethylene and IAA by H. frisingense.[24]
Diversity
Endophytic species are very diverse; only a small minority of existing endophytes have been characterized.[26][27] Many endophytes are in the phyla Basidiomycota and Ascomycota. Endophytic fungi may be from Hypocreales and Xylariales of the Sordariomycetes (Pyrenomycetes) class or from the class of Loculoascomycetes.[28] One group of fungal endophytes are the arbuscular mycorrhizal fungi involving biotrophic Glomeromycota associated with various plant species.[29] As often with other organisms associated with plants such as mycorrhizal fungus, endophytes gain carbon from their association with the plant host. Bacterial endophytes are polyphyletic, belonging to broad range of taxa, including α-Proteobacteria, β-Proteobacteria, γ-Proteobacteria, Firmicutes, Actinobacteria.[30]
One or more endophytic organisms are found in nearly every land plant.[31] It is suggested that areas of high plant diversity such as tropical rainforests may also contain the highest diversity of endophytic organisms that possess novel and diverse chemical metabolites.[32] It has been estimated that there could be approximately 1 million endophytic fungi that exist in the world.[32]
A diazotrophic bacterium isolated in lodgepole pines (Pinus contorta) in British Columbia, Canada, is Paenibacillus polymyxa, which may help its host by fixing nitrogen.[33][34][35][36][37][38]
Classification
Endophytes include a wide variety of microorganisms including fungi, bacteria and viruses. There are two different means of classifying endophytes.
Systemic and non-systemic
The first method divides endophytes into two categories: systemic (true) and nonsystemic (transient). These categories are based on the endophyte's genetics, biology, and mechanism of transmission from host to host.[39] Systemic endophytes are defined as organisms that live within plant tissues for the entirety of its life cycle and participate in a symbiotic relationship without causing disease or harm to the plant at any point. Additionally, systemic endophytes concentrations and diversity do not change in a host with changing environmental conditions.[39] Non-systemic or transient endophytes on the other hand vary in number and diversity within their plant hosts under changing environmental conditions. Non-systemic endophytes have also been shown to become pathogenic to their host plants under stressful or resource limited growing conditions.[39] An example of this would be Colletotrichum fioriniae, which is an endophyte of many temperate broadleaved trees and shrubs, but can also be a pathogen on many fruits and some leaves.[40][41]
Clavicipitaceous and non-clavicipitaceous
The second method divides fungal endophytes into four groups based on taxonomy and six other criteria: host range, host tissues colonized, in planta colonization, in planta biodiversity, mode of transmission and fitness benefits.[42] These four groups are divided into clavicipitaceous endophytes (Class 1) and non-clavicipitaceous endophytes (Class 2, 3, and 4).
Class 1 endophytes are all phylogenetically related and proliferate within cool and warm season grasses. They typically colonize plant shoots where they form a systemic intercellular infection. Class 1 endophytes are mainly transmitted from host to host by vertical transmission, in which maternal plants pass fungi on to their offspring through seeds. Class 1 endophytes can further be divided into Types I, II and III. Among these three types of clavicipitaceous endophytes are different interactions with their plant hosts. These interaction range from pathogenic to symbiotic and symptomatic to asymptomatic. Type III clavicipitaceous endophytes grow within their plant host without manifesting symptoms of disease or harming their host. Class 1 endophytes typically confer benefits on their plant host such as improving plant biomass, increasing drought tolerance and increasing the production of chemicals that are toxic and unappetizing to animals, thereby decreasing herbivory. These benefits can vary depending on the host and environmental conditions.[42]
Non-clavicipitaceous endophytes represent a polyphyletic group of organisms. Non-clavicipitaceous endophytes are typically Ascomycota fungi. The ecological roles of these fungi are diverse and still poorly understood. These endophyte plant interactions are widespread and have been found in nearly all land plants and ecosystems.[42] Many non-clavicipitaceous endophytes have the ability to switch between endophytic behavior and free-living lifestyles. Non-clavicipitaceous endophytes are divided into class 2, 3 and 4. Class 2 endophytes can grow in plant tissues both above and below ground. This class of non-clavicipitaceous endophytes has been the most extensively researched and has been shown to enhance fitness benefits of their plant host as a result of habitat-specific stresses such as pH, temperature and salinity.[42] Class 3 endophytes are restricted to growth in above ground plant tissues and form in localized areas of plant tissue. Class 4 endophytes are restricted to plant tissues below ground and can colonize much more of the plant tissue. These classes of non-clavicipitaceous endophytes have not been as extensively studied to date.[42]
Applications
Endophytes may have potential future applications in agriculture.[43][44][45][46][47][48][49] Use of endophytes might potentially increase crop yields.[50] Turfgrass seed of Festuca and Lolium perenne infected with fungal inoculants, Acremonium coenophialum and A. lolii, is commercially available for use in growing lawns which might require less pesticide use -the grasses are poisonous to cattle and more resistant to some insect damage. As of 1999 this is only available in the afore-mentioned lawn grasses, which are sold as 'low maintenance' cultivars. The fungi cause the grasses to contain toxic alkaloids. The products provide high resistance to foliar lawn pests such as billbugs, chinch bugs, sod webworms, fall army-worms and Argentine stem weevils, but offer little protection to pests of grass roots such as grubs. The endophytes can survive most pesticides and are even resistant to some fungicides, and are very suitable for use in Integrated Pest Management.[51]
Biofuel
A 2008 experiment with an isolate of a fungus called NRRL 50072 found that this strain can produce a small amount of fuel-like hydrocarbon compounds which was promoted as "myco-diesel". It was hoped that perhaps in the future this might provide a possible source of biofuel. It was first misidentified as the endophyte Gliocladium roseum, but later research showed that it was in fact the saprophyte Ascocoryne sarcoides.[52][53]
A strain of endophytic fungi which appeared to be closely related to Nigrograna mackinnonii which was isolated from a stem of the plant Guazuma ulmifolia collected in Ecuador was found to produce a variety of volatile organic compounds including terpenes and odd chain polyenes. The polyenes isolated from the fungus have properties that are sought in gasoline-surrogate biofuels.[54]
Phytoremediation
Plants are potentially able to break down or sequester, or stimulate micro-organisms in the soil to break down or sequester, certain organic pollutants and inorganic pollutants such as nickel in degraded ecosystems, which is known as phytoremediation. In this endophytes may possibly assist plants in converting pollutants into less biologically harmful forms; in one of the few experiments performed a plasmid called TOM from a strain of a bacterium in the Burkholderia genus known as G4 which can break down trichloroethylene (TCE) was transferred to endophytes of popular trees; although it did not help the plants remove more of this chemical than non-inoculated plants, the plants transpired less TCE into the air. In another experiment Burkholderia bacteria with both the TOM plasmid as well as nickel resistance genes was inoculated into yellow lupine; this increased the root mass of the plants, but the amounts of TCE transpired was not statistically significant. Despite these failures, such techniques might lead to some future improvements.[55]
Two strains of the endophytic fungi Pestalotiopsis microspora isolated from stems of plants from the Ecuadorian rainforest were shown in laboratory experiments to be able to digest polyurethane plastic as the fungus's sole carbon source in anaerobic conditions, although many other non-endophytic fungi have demonstrated this ability, and most isolates of endophytic fungi in this experiment could perform this to some degree.[56]
Drug discovery
Endophytes produce a wide variety of secondary metabolites that might be useful as lead compounds in drug discovery.[57][58] Endophyte bioprospecting has already yielded compounds with antibacterial,[59][60] antifungal,[61] antiviral,[61] antiparasitic,[62] cytotoxic,[59][63] neuroprotective,[62] antioxidant,[62] insulin-mimetic,[62] α-glucosidase inhibitory,[61] and immunosuppressant[62] properties. Manipulations of a plant's endosymbiots can affect plant development, growth and ultimately the quality and quantity of compounds harvested from the plant.[11] Studies have shown endophytic fungi are able to produce secondary metabolites previously thought to be manufactured by their plant hosts. The presence of these metabolites in plants could be attributable to endophyte production alone, or to combined endophyte and plant production following transfer of the corresponding genes from endophyte to plant or vice versa.[62]
A well known example of the discovery of chemicals derived from endophytic fungi is from the fungus Taxomyces andreanae isolated from the pacific yew Taxus brevifolia. T. andreanae produces paclitaxel, also known as taxol. This drug is important for the treatment of cancer. Other endophytes since have been discovered that also produce paclitaxel in other host species, but to date there has been no successful industrial source of paclitaxel created.[62]
Endophytes have been discovered with various anti-tumor properties. Endophytic fungi produce many secondary compounds such as alkaloids, triterpenes and steroids which have been shown to have anti-tumor effects.[59] The alkaloid beauvericin has been isolated from the fungus Fusarium oxysporum and has shown cytotoxicity against the tumor cells PC3, PANC-1, and A549.[64][65] Two fusarubin derivatives: anhydrofusarubin and methyl ether of fusarubin were isolated from endophytic fungus Cladosporium sp. and have shown cytotoxicity against human leukemia (K-562).[59] Three triterpenes were found in the endophyte Xylarialean sp., all three of these compounds displayed mild cytotoxic effects on tumor cells.[65]
Some of the antimicrobial compounds produced by endophytic fungi are of interest in their effectiveness against pathogens which have developed resistances to antibiotics. Different fractions of Cladosporium sp. including secondary metabolite-methyl ether of fusarubin have shown antibacterial activity against Staphylococcus aureus, E. coli, P. aeruginosa, and Bacillus megaterium.[59] Several isolates from the ascomycota Pestalotiopsis sp. have been shown to have a broad range of antimicrobial effects,[32] even against methicillin-resistant Staphylococcus aureus.[66] Also, compounds from the marine fungus Nigrospora sp. have activity against strains of multi drug-resistant Mycobacterium tuberculosis.[67]
An endophytic fungus of the genus Pseudomassaria has been found in the rainforest of the Democratic Republic of the Congo. This fungus yields a metabolite that shows potential as an antidiabetic, also known as an insulin mimetic. This compound acts like insulin and has been shown to lower blood glucose levels in mouse model experiments.[31]
Agriculture
Among the many promising applications of endophytic microbes are those intended to increase agricultural use of endophytes to produce crops that grow faster and are more resistant and hardier than crops lacking endophytes.[68] Epichloë endophytes are being widely used commercially in turf grasses to enhance the performance of the turf and its resistance to biotic and abiotic stresses.[69] Piriformospora indica is an interesting endophytic fungus of the order Sebacinales, the fungus is capable of colonising roots and forming symbiotic relationship with many plants.[70]
Endophytes appear to enhance the growth of their plant host symbionts. Endophytes also provide their hosts with an increased resilience to both abiotic and biotic stressors such as drought, poor soils and herbivory. The increased growth and resilience is likely caused by the endophytes ability to improve plant nutrition or secondary metabolite production, as in the case of Phoma eupatorii's inhibition of the phytopathogen Phytophthora infestans.[71] Endophytes accomplish this by increasing the uptake of valuable land limited nutrients from the soil such as phosphorus and making other plant nutrients available to plants such as rock phosphate and atmospheric nitrogen which are normally trapped in forms that are inaccessible to plants.[43]
Many endophytes protect plants from herbivory from both insects and animals by producing secondary metabolites that are either unappetizing or toxic to the herbivore.[72] Increasingly there has been great importance placed on endophytes that protect valuable crops from invasive insects. One example of an endophyte-plant-insect interaction is located in the New Zealand grasslands, where endophytes, known as AR1 and AR37 are utilized to protect valuable ryegrass from the Argentine stem weevil but remain palatable to another important food source, livestock.[73]
There are several endophytes that have been discovered that exhibit insecticidal properties. One such endophyte comes from the Nodulisporium sp. which was first harvested from the plant Bontia daphnoides. Indole diterpenes, known as nodulisporic acids, have been harvested from this endophyte which have effective insecticidal properties against the blowfly larvae.[31]
There are many obstacles to successfully implementing the use of endophytes in agriculture. Despite the many known benefits that endophytes may confer to their plant hosts, conventional agricultural practices continue to take priority. Current agriculture relies heavily on fungicides and high levels of chemical fertilizers. The use of fungicides has a negative effect on endophytic fungi and fertilizers reduce a plant's dependence on its endophytic symbiont.[73] Despite this, the interest and use of bio-insecticides and using endophytes to aid in plant growth is increasing as organic and sustainable agriculture is considered more important. As humans become more aware of the damage that synthetic insecticides cause to the environment and beneficial insects such as bees and butterflies biological insecticides may become more important to the agricultural industry.[31]
See also
- Biofertilizer
- List of endophytes
- Plant use of endophytic fungi in defense
- Arbuscular mycorrhiza
- Mycorrhiza
- Rhizobia
References
- ↑ Chow, Chanelle; Padda, Kiran Preet; Puri, Akshit; Chanway, Chris P. (2022-09-20). "An Archaic Approach to a Modern Issue: Endophytic Archaea for Sustainable Agriculture" (in en). Current Microbiology 79 (11): 322. doi:10.1007/s00284-022-03016-y. ISSN 1432-0991. PMID 36125558. https://doi.org/10.1007/s00284-022-03016-y.
- ↑ 2.0 2.1 "The Hidden World within Plants: Ecological and Evolutionary Considerations for Defining Functioning of Microbial Endophytes". Microbiology and Molecular Biology Reviews 79 (3): 293–320. September 2015. doi:10.1128/MMBR.00050-14. PMID 26136581.
- ↑ Das, Saurav; Barooah, Madhumita; Thakur, Nagendra (2019-04-17). "Endophytic Virome" (in en). bioRxiv: 602144. doi:10.1101/602144. https://www.biorxiv.org/content/10.1101/602144v2.
- ↑ "Endophyte research: going beyond isolation and metabolite documentation". Fungal Ecology 6 (6): 561–568. 2013-12-01. doi:10.1016/j.funeco.2013.09.007.
- ↑ "Epichloë spp. associated with grasses: new insights on life cycles, dissemination and evolution". Mycologia 106 (2): 181–201. 2014-03-01. doi:10.3852/106.2.181. PMID 24877257.
- ↑ Pinski, Artur; Betekhtin, Alexander; Hupert-Kocurek, Katarzyna; Mur, Luis A. J.; Hasterok, Robert (2019). "Defining the Genetic Basis of Plant–Endophytic Bacteria Interactions". International Journal of Molecular Sciences 20 (8): 1947. doi:10.3390/ijms20081947. PMID 31010043. 50px Material was copied from this source, which is available under a Creative Commons Attribution 4.0 International License.
- ↑ "Exploring the evolutionary ecology of fungal endophytes in agricultural systems: using functional traits to reveal mechanisms in community processes". Evolutionary Applications 3 (5–6): 525–37. September 2010. doi:10.1111/j.1752-4571.2010.00141.x. PMID 25567944.
- ↑ Abdelfattah, Ahmed; Wisniewski, Michael; Schena, Leonardo; Tack, Ayco J. M. (2021). "Experimental evidence of microbial inheritance in plants and transmission routes from seed to phyllosphere and root" (in en). Environmental Microbiology 23 (4): 2199–2214. doi:10.1111/1462-2920.15392. ISSN 1462-2920. PMID 33427409.
- ↑ "Bacterial seed endophyte shapes disease resistance in rice". Nature Plants 7 (1): 60–72. January 2021. doi:10.1038/s41477-020-00826-5. PMID 33398157.
- ↑ (in en) Radical Mycology: A Treatise on Seeing & Working with Fungi. Chthaeus Press. 2016. p. 177. ISBN 9780986399602. https://archive.org/stream/radicalmicologytexts/Radical_Mycology_A_treatise_on_seeing_and_working_with_fungi.
- ↑ 11.0 11.1 "A Friendly Relationship between Endophytic Fungi and Medicinal Plants: A Systematic Review". Frontiers in Microbiology 7: 906. 2016-06-09. doi:10.3389/fmicb.2016.00906. PMID 27375610.
- ↑ "Plant-fungal interactions: What triggers the fungi to switch among lifestyles?". Critical Reviews in Microbiology 42 (3): 428–38. May 2016. doi:10.3109/1040841X.2014.958052. PMID 25383649.
- ↑ "Clavicipitaceous endophytes: Their ability to enhance resistance of grasses to multiple stresses". Biological Control. Special Issue: Endophytes 46 (1): 57–71. 2008-07-01. doi:10.1016/j.biocontrol.2008.01.023.
- ↑ "Fungal endophytes and their interaction with plant pathogens: a review" (in En). Spanish Journal of Agricultural Research 6 (S1): 138–146. 2008-02-01. doi:10.5424/sjar/200806S1-382. ISSN 2171-9292.
- ↑ "Properties of bacterial endophytes and their proposed role in plant growth" (in en). Trends in Microbiology 16 (10): 463–71. October 2008. doi:10.1016/j.tim.2008.07.008. PMID 18789693. https://pure.rug.nl/ws/files/234979633/Properties_of_bacterial_endophytes_and_their_proposed_role_in_plant_growth.pdf.
- ↑ 16.0 16.1 "Epichloë endophytes grow by intercalary hyphal extension in elongating grass leaves". Fungal Genetics and Biology 45 (2): 84–93. February 2008. doi:10.1016/j.fgb.2007.07.013. PMID 17919950.
- ↑ "In planta regulation of extension of an endophytic fungus and maintenance of high metabolic rates in its mycelium in the absence of apical extension". Applied and Environmental Microbiology 67 (12): 5377–83. December 2001. doi:10.1128/aem.67.12.5377-5383.2001. PMID 11722882. Bibcode: 2001ApEnM..67.5377T.
- ↑ "Do the costs and benefits of fungal endophyte symbiosis vary with light availability?". The New Phytologist 188 (3): 824–34. November 2010. doi:10.1111/j.1469-8137.2010.03428.x. PMID 20955417.
- ↑ "Chemical ecology of endophytic fungi: origins of secondary metabolites". Chemistry & Biology 19 (7): 792–8. July 2012. doi:10.1016/j.chembiol.2012.06.004. PMID 22840767.
- ↑ 20.0 20.1 Khan, Zareen; Kandel, Shyam L.; Ramos, Daniela N.; Ettl, Gregory J.; Kim, Soo-Hyung; Doty, Sharon L. (October 2015). "Increased Biomass of Nursery-Grown Douglas-Fir Seedlings upon Inoculation with Diazotrophic Endophytic Consortia" (in en). Forests 6 (10): 3582–3593. doi:10.3390/f6103582.
- ↑ Kandel, Shyam L.; Firrincieli, Andrea; Joubert, Pierre M.; Okubara, Patricia A.; Leston, Natalie D.; McGeorge, Kendra M.; Mugnozza, Giuseppe S.; Harfouche, Antoine et al. (2017). "An In vitro Study of Bio-Control and Plant Growth Promotion Potential of Salicaceae Endophytes" (in English). Frontiers in Microbiology 8: 386. doi:10.3389/fmicb.2017.00386. ISSN 1664-302X. PMID 28348550.
- ↑ 22.0 22.1 22.2 22.3 Rho, Hyungmin; Kim, Soo-Hyung (2017), Doty, Sharon Lafferty, ed., "Endophyte Effects on Photosynthesis and Water Use of Plant Hosts: A Meta-Analysis" (in en), Functional Importance of the Plant Microbiome: Implications for Agriculture, Forestry and Bioenergy (Cham: Springer International Publishing): pp. 43–69, doi:10.1007/978-3-319-65897-1_4, ISBN 978-3-319-65897-1, https://doi.org/10.1007/978-3-319-65897-1_4, retrieved 2021-06-10
- ↑ Rho, Hyungmin; Van Epps, Victor; Wegley, Nicholas; Doty, Sharon L.; Kim, Soo-Hyung (2018). "Salicaceae Endophytes Modulate Stomatal Behavior and Increase Water Use Efficiency in Rice" (in English). Frontiers in Plant Science 9: 188. doi:10.3389/fpls.2018.00188. ISSN 1664-462X. PMID 29552021.
- ↑ 24.0 24.1 24.2 24.3 Straub, Daniel; Yang, Huaiyu; Liu, Yan; Tsap, Tatsiana; Ludewig, Uwe (2013-11-01). "Root ethylene signalling is involved in Miscanthus sinensis growth promotion by the bacterial endophyte Herbaspirillum frisingense GSF30T". Journal of Experimental Botany 64 (14): 4603–4615. doi:10.1093/jxb/ert276. ISSN 0022-0957. PMID 24043849. PMC 3808336. https://doi.org/10.1093/jxb/ert276.
- ↑ Rothballer, Michael; Eckert, Barbara; Schmid, Michael; Fekete, Agnes; Schloter, Michael; Lehner, Angelika; Pollmann, Stephan; Hartmann, Anton (2008-10-01). "Endophytic root colonization of gramineous plants by Herbaspirillum frisingense". FEMS Microbiology Ecology 66 (1): 85–95. doi:10.1111/j.1574-6941.2008.00582.x. ISSN 0168-6496. PMID 18761671.
- ↑ "Endophytic Microbes in Crops: Diversity and Beneficial Impact for Sustainable Agriculture". Microbial Inoculants in Sustainable Agricultural Productivity. Springer India. 2016-01-01. pp. 117–143. doi:10.1007/978-81-322-2647-5_7. ISBN 9788132226451.
- ↑ "Fungal endophytes: diversity and functional roles". The New Phytologist 182 (2): 314–30. 2009. doi:10.1111/j.1469-8137.2009.02773.x. PMID 19236579.
- ↑ "Diversity of Fungal Endophytes in Temperate Forest Trees" (in en). Endophytes of Forest Trees. Forestry Sciences. 80. Springer Netherlands. 2011-01-01. pp. 31–46. doi:10.1007/978-94-007-1599-8_2. ISBN 978-94-007-1598-1.
- ↑ "Mycorrhizal ecology and evolution: the past, the present, and the future". The New Phytologist 205 (4): 1406–23. March 2015. doi:10.1111/nph.13288. PMID 25639293.
- ↑ "Bacterial endophytes in agricultural crops and their role in stress tolerance: a review" (in en-US). Zemdirbyste-Agriculture 102 (4): 465–478. 2015. doi:10.13080/z-a.2015.102.060.
- ↑ 31.0 31.1 31.2 31.3 "Bioprospecting for microbial endophytes and their natural products". Microbiology and Molecular Biology Reviews 67 (4): 491–502. December 2003. doi:10.1128/mmbr.67.4.491-502.2003. PMID 14665674.
- ↑ 32.0 32.1 32.2 "Endophytic fungi: a reservoir of antibacterials" (in en). Frontiers in Microbiology 5: 715. 2015. doi:10.3389/fmicb.2014.00715. PMID 25620957.
- ↑ "Isolation and identification of endophytic diazotrophs from lodgepole pine trees growing at unreclaimed gravel mining pits in central interior British Columbia, Canada". Canadian Journal of Forest Research 48 (12): 1601–1606. 2018-09-20. doi:10.1139/cjfr-2018-0347.
- ↑ "Endophytic nitrogen fixation - a possible 'hidden' source of nitrogen for lodgepole pine trees growing at unreclaimed gravel mining sites". FEMS Microbiology Ecology 95 (11). November 2019. doi:10.1093/femsec/fiz172. PMID 31647534.
- ↑ "Evidence of endophytic diazotrophic bacteria in lodgepole pine and hybrid white spruce trees growing in soils with different nutrient statuses in the West Chilcotin region of British Columbia, Canada" (in en). Forest Ecology and Management 430: 558–565. 2018-12-15. doi:10.1016/j.foreco.2018.08.049.
- ↑ "Can naturally-occurring endophytic nitrogen-fixing bacteria of hybrid white spruce sustain boreal forest tree growth on extremely nutrient-poor soils?" (in en). Soil Biology and Biochemistry 140: 107642. 2020-01-01. doi:10.1016/j.soilbio.2019.107642.
- ↑ "In vitro and in vivo analyses of plant-growth-promoting potential of bacteria naturally associated with spruce trees growing on nutrient-poor soils" (in en). Applied Soil Ecology 149: 103538. 2020-05-01. doi:10.1016/j.apsoil.2020.103538.
- ↑ "Sustaining the growth of Pinaceae trees under nutrient-limited edaphic conditions via plant-beneficial bacteria". PLOS ONE 15 (8): e0238055. 2020-08-26. doi:10.1371/journal.pone.0238055. PMID 32845898. Bibcode: 2020PLoSO..1538055P.
- ↑ 39.0 39.1 39.2 "Plant-endophyte symbiosis, an ecological perspective". Applied Microbiology and Biotechnology 99 (7): 2955–65. April 2015. doi:10.1007/s00253-015-6487-3. PMID 25750045.
- ↑ Lin, Shan; Peduto Hand, Francesca (2019). "Determining the Sources of Primary and Secondary Inoculum and Seasonal Inoculum Dynamics of Fungal Pathogens Causing Fruit Rot of Deciduous Holly" (in en). Plant Disease 103 (5): 951–958. doi:10.1094/PDIS-09-18-1694-RE. ISSN 0191-2917. PMID 30880556.
- ↑ Martin, Phillip L.; Peter, Kari A. (2021). "Quantification of Colletotrichum fioriniae in Orchards and Deciduous Forests Indicates It Is Primarily a Leaf Endophyte" (in en). Phytopathology 111 (2): 333–344. doi:10.1094/PHYTO-05-20-0157-R. ISSN 0031-949X. PMID 32729787.
- ↑ 42.0 42.1 42.2 42.3 42.4 "Fungal endophytes: diversity and functional roles". The New Phytologist 182 (2): 314–30. 2009-04-01. doi:10.1111/j.1469-8137.2009.02773.x. PMID 19236579.
- ↑ 43.0 43.1 "Fungal growth promotor endophytes: a pragmatic approach towards sustainable food and agriculture" (in en). Symbiosis 62 (2): 63–79. 2014-02-01. doi:10.1007/s13199-014-0273-3.
- ↑ "Seedling growth promotion and nitrogen fixation by a bacterial endophyte Paenibacillus polymyxa P2b-2R and its GFP derivative in corn in a long-term trial". Symbiosis 69 (2): 123–129. 2016-06-01. doi:10.1007/s13199-016-0385-z. ISSN 1878-7665.
- ↑ "Plant growth promotion and nitrogen fixation in canola (Brassica napus) by an endophytic strain of Paenibacillus polymyxa and its GFP-tagged derivative in a long-term study". Botany 94 (12): 1209–1217. 2016-07-07. doi:10.1139/cjb-2016-0075.
- ↑ "Can a diazotrophic endophyte originally isolated from lodgepole pine colonize an agricultural crop (corn) and promote its growth?" (in en). Soil Biology and Biochemistry 89: 210–216. 2015-10-01. doi:10.1016/j.soilbio.2015.07.012. ISSN 0038-0717.
- ↑ "Effect of GFP tagging of Paenibacillus polymyxa P2b-2R on its ability to promote growth of canola and tomato seedlings". Biology and Fertility of Soils 52 (3): 377–387. 2016-04-01. doi:10.1007/s00374-015-1083-3.
- ↑ "Evidence of nitrogen fixation and growth promotion in canola (Brassica napus L.) by an endophytic diazotroph Paenibacillus polymyxa P2b-2R". Biology and Fertility of Soils 52 (1): 119–125. 2016-01-01. doi:10.1007/s00374-015-1051-y.
- ↑ "Effect of GFP-tagging on nitrogen fixation and plant growth promotion of an endophytic diazotrophic strain of Paenibacillus polymyxa". Botany 95 (9): 933–942. 2017-07-14. doi:10.1139/cjb-2017-0056.
- ↑ "Plant growth-promoting bacteria in the rhizo- and endosphere of plants: Their role, colonization, mechanisms involved and prospects for utilization". Soil Biology and Biochemistry 42 (5): 669–678. 2010-05-01. doi:10.1016/j.soilbio.2009.11.024. http://repository.edulll.gr/2323.
- ↑ "University of Rhode Island GreenShare Factsheets: Endopyhte-Enhanced Grasses". http://www.uri.edu/ce/factsheets/sheets/endophyte.html.
- ↑ "The production of myco-diesel hydrocarbons and their derivatives by the endophytic fungus Gliocladium roseum (NRRL 50072)". Microbiology 154 (Pt 11): 3319–3328. November 2008. doi:10.1099/mic.0.2008/022186-0. PMID 18957585.
- ↑ "Volatile organic compound production by organisms in the genus Ascocoryne and a re-evaluation of myco-diesel production by NRRL 50072". Microbiology 156 (Pt 12): 3814–3829. December 2010. doi:10.1099/mic.0.041327-0. PMID 20705658.
- ↑ "Biosynthesis and genomic analysis of medium-chain hydrocarbon production by the endophytic fungal isolate Nigrograna mackinnonii E5202H". Applied Microbiology and Biotechnology 99 (8): 3715–28. April 2015. doi:10.1007/s00253-014-6206-5. PMID 25672844.
- ↑ Plant Biotechnology and Agriculture: Prospects for the 21st Century. Academic press. 2011. pp. 319. ISBN 9780123814661. OCLC 858878994.
- ↑ "Biodegradation of polyester polyurethane by endophytic fungi". Applied and Environmental Microbiology 77 (17): 6076–84. September 2011. doi:10.1128/AEM.00521-11. PMID 21764951. Bibcode: 2011ApEnM..77.6076R.
- ↑ "Fungal endophytes as prolific source of phytochemicals and other bioactive natural products: A review". Microbial Pathogenesis 82: 50–9. May 2015. doi:10.1016/j.micpath.2015.04.001. PMID 25865953.
- ↑ "Marine Algicolous Endophytic Fungi - A Promising Drug Resource of the Era". Journal of Microbiology and Biotechnology 27 (6): 1039–1052. June 2017. doi:10.4014/jmb.1701.01036. PMID 28376612.
- ↑ 59.0 59.1 59.2 59.3 59.4 "Cytotoxic and antibacterial naphthoquinones from an endophytic fungus, Cladosporium sp.". Toxicology Reports 3: 861–865. Oct 2016. doi:10.1016/j.toxrep.2016.10.005. PMID 28959613.
- ↑ "Bioprospecting for antibacterial drugs: a multidisciplinary perspective on natural product source material, bioassay selection and avoidable pitfalls". Pharmaceutical Research 37 (7): Article 125. June 2020. doi:10.1007/s11095-020-02849-1. PMID 32529587. https://zenodo.org/record/3909383.
- ↑ 61.0 61.1 61.2 "Lead compounds from mangrove-associated microorganisms". Marine Drugs 16 (9): Article 319. September 2018. doi:10.3390/md16090319. PMID 30205507.
- ↑ 62.0 62.1 62.2 62.3 62.4 62.5 62.6 "Fungal endophytes: unique plant inhabitants with great promises". Applied Microbiology and Biotechnology 90 (6): 1829–45. June 2011. doi:10.1007/s00253-011-3270-y. PMID 21523479.
- ↑ El-Hawary, S.s.; Mohammed, R.; AbouZid, S.f.; Bakeer, W.; Ebel, R.; Sayed, A.m.; Rateb, M.e. (2016-04-01). "Solamargine production by a fungal endophyte of Solanum nigrum" (in en). Journal of Applied Microbiology 120 (4): 900–911. doi:10.1111/jam.13077. ISSN 1365-2672. PMID 26811095.
- ↑ "Chemical constituents from endophytic fungus Fusarium oxysporum". Fitoterapia 82 (5): 777–81. July 2011. doi:10.1016/j.fitote.2011.04.002. PMID 21497643.
- ↑ 65.0 65.1 "Endophytic fungi with antitumor activities: Their occurrence and anticancer compounds". Critical Reviews in Microbiology 42 (3): 454–73. May 2016. doi:10.3109/1040841x.2014.959892. PMID 25343583.
- ↑ "On the antibiotic and antifungal activity of pestalone, pestalachloride A, and structurally related compounds" (in en-US). Journal of Natural Products 76 (8): 1519–22. August 2013. doi:10.1021/np400301d. PMID 23905700.
- ↑ "Anti-mycobacterial activity of marine fungus-derived 4-deoxybostrycin and nigrosporin". Molecules 18 (2): 1728–40. January 2013. doi:10.3390/molecules18021728. PMID 23434859.
- ↑ "Microbial Endophytes: Future Challenges". Advances in Endophytic Research. Springer India. 2014-01-01. pp. 441–451. doi:10.1007/978-81-322-1575-2_22. ISBN 978-81-322-1574-5.
- ↑ Chapter 20: Biology and Applications of Fungal Endophytes in Turfgrasses. American Society of Agronomy. 2012. pp. Chapter 20.
- ↑ "Piriformospora indica-a mutualistic basidiomycete with an exceptionally large plant host range". Molecular Plant Pathology 13 (5): 508–18. June 2012. doi:10.1111/j.1364-3703.2011.00764.x. PMID 22111580.
- ↑ "Broad-spectrum inhibition of Phytophthora infestans by fungal endophytes". FEMS Microbiology Ecology 94 (4). April 2018. doi:10.1093/femsec/fiy037. PMID 29528408.
- ↑ Vicari, Mark; Bazely, Dawn R. (1993-04-01). "Do grasses fight back? The case for antiherbivore defences" (in en). Trends in Ecology & Evolution 8 (4): 137–141. doi:10.1016/0169-5347(93)90026-L. ISSN 0169-5347. PMID 21236130. https://dx.doi.org/10.1016/0169-5347%2893%2990026-L.
- ↑ 73.0 73.1 "Exploitation of endophytes for sustainable agricultural intensification". Molecular Plant Pathology 18 (3): 469–473. April 2017. doi:10.1111/mpp.12483. PMID 27559722.
![]() | Original source: https://en.wikipedia.org/wiki/Endophyte.
Read more |