Biology:Deep-sea gigantism
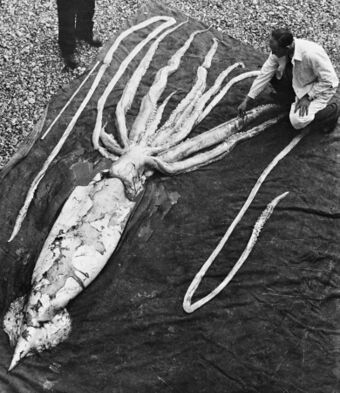
In zoology, deep-sea gigantism or abyssal gigantism is the tendency for species of invertebrates and other deep-sea dwelling animals to be larger than their shallower-water relatives across a large taxonomic range. Proposed explanations for this type of gigantism include colder temperature, food scarcity, reduced predation pressure and increased dissolved oxygen concentrations in the deep sea. The inaccessibility of abyssal habitats has hindered the study of this topic.
Taxonomic range
In marine crustaceans, the trend of increasing size with depth has been observed in mysids, euphausiids, decapods, isopods and amphipods.[1][2] Non-arthropods in which deep-sea gigantism has been observed are cephalopods, cnidarians, and eels from the order Anguilliformes.[3]
“ | Other [animals] attain under them gigantic proportions. It is especially certain crustacea which exhibit this latter peculiarity, but not all crustacea, for the crayfish like forms in the deep sea are of ordinary size. I have already referred to a gigantic Pycnogonid [sea spider] dredged by us. Louis Agassiz dredged a gigantic Isopod 11 inches [28 centimetres] in length. We also dredged a gigantic Ostracod. For over 125 years, scientists have contemplated the extreme size of Bathynomus giganteus. – Henry Nottidge Moseley, 1880[4] | ” |
Examples of deep-sea gigantism include the big red jellyfish,[5] the giant isopod,[4] giant ostracod,[4] the giant sea spider,[4] the giant amphipod, the Japanese spider crab, the giant oarfish, the deepwater stingray, the seven-arm octopus,[6] and a number of squid species: the colossal squid (up to 14 m in length),[7] the giant squid (up to 12 m),[7] Onykia robusta, Taningia danae, Galiteuthis phyllura, Kondakovia longimana, and the bigfin squid.
Deep-sea gigantism is not generally observed in the meiofauna (organisms that pass through a 1 mm mesh), which actually exhibit the reverse trend of decreasing size with depth.[8]
Explanations
Lower temperature
In crustaceans, it has been proposed that the explanation for the increase in size with depth is similar to that for the increase in size with latitude (Bergmann's rule): both trends involve increasing size with decreasing temperature.[1] The trend with latitude has been observed in some of the same groups, both in comparisons of related species, as well as within widely distributed species.[1] Decreasing temperature is thought to result in increased cell size and increased life span (the latter also being associated with delayed sexual maturity[8]), both of which lead to an increase in maximum body size (continued growth throughout life is characteristic of crustaceans).[1] In Arctic and Antarctic seas where there is a reduced vertical temperature gradient, there is also a reduced trend towards increased body size with depth, arguing against hydrostatic pressure being an important parameter.[1]
Temperature does not appear to have a similar role in influencing the size of giant tube worms. Riftia pachyptila, which lives in hydrothermal vent communities at ambient temperatures of 2–30 °C,[9] reaches lengths of 2.7 m, comparable to those of Lamellibrachia luymesi, which lives in cold seeps. The former, however, has rapid growth rates and short life spans of about 2 years,[10] while the latter is slow growing and may live over 250 years.[11]
Food scarcity
Food scarcity at depths greater than 400 m is also thought to be a factor, since larger body size can improve ability to forage for widely scattered resources.[8] In organisms with planktonic eggs or larvae, another possible advantage is that larger offspring, with greater initial stored food reserves, can drift for greater distances.[8] As an example of adaptations to this situation, giant isopods gorge on food when available, distending their bodies to the point of compromising ability to locomote;[12] they can also survive 5 years without food in captivity.[13][14]
According to Kleiber's law,[15] the larger an animal gets, the more efficient its metabolism becomes; i.e., an animal's basal metabolic rate scales to roughly the ¾ power of its mass. Under conditions of limited food supply, this may provide additional benefit to large size.
Reduced predation pressure
An additional possible influence is reduced predation pressure in deeper waters.[16] A study of brachiopods found that predation was nearly an order of magnitude less frequent at the greatest depths than in shallow waters.[16]
Increased dissolved oxygen
Dissolved oxygen levels are also thought to play a role in deep-sea gigantism. A 1999 study of benthic amphipod crustaceans found that maximum potential organism size directly correlates with increased dissolved oxygen levels of deeper waters.[17] The solubility of dissolved oxygen in the oceans is known to increase with depth because of increasing pressure, decreasing salinity levels and temperature.[17]
The proposed theory behind this trend is that deep-sea gigantism could be an adaptive trait to combat asphyxiation in ocean waters.[18] Larger organisms are able to intake more dissolved oxygen within the ocean, allowing for sufficient respiration. However, this increased absorption of oxygen runs the risk of toxicity poisoning where an organism can have oxygen levels that are so high that they become harmful and poisonous.[18]
Gallery
A giant isopod (Bathynomus giganteus) may reach up to 0.76 m (2 ft 6 in) in length.
A Japanese spider crab whose outstretched legs measured 3.7 m (12 ft) across.
A robust clubhook squid, whose mantle reaches 2 m (6 ft 7 in) in length, caught off Alaska.
A 7 m (23 ft) king of herrings oarfish, caught off California .
A Stygiomedusa jellyfish, which can grow up to 10 m (33 ft) in length.
A deepwater stingray, which can reach up to 2.7 m × 1.5 m (8 ft 10 in × 4 ft 11 in) in size.
See also
- Cephalopod size
- Dwarfing
- Island gigantism
- Insular dwarfism
- Largest organisms
- Megafauna
References
- ↑ 1.0 1.1 1.2 1.3 1.4 Timofeev, S. F. (2001). "Bergmann's Principle and Deep-Water Gigantism in Marine Crustaceans". Biology Bulletin of the Russian Academy of Sciences 28 (6): 646–650. doi:10.1023/A:1012336823275.
- ↑ C., McClain; M., Rex (2001-10-01). "The relationship between dissolved oxygen concentration and maximum size in deep-sea turrid gastropods: an application of quantile regression". Marine Biology 139 (4): 681–685. doi:10.1007/s002270100617. ISSN 0025-3162. http://link.springer.com/10.1007/s002270100617.
- ↑ Hanks, Micah. "Deep Sea Gigantism: Curious Cases of Mystery Giant Eels". https://mysteriousuniverse.org/2011/05/deep-sea-gigantism-curious-cases-of-mystery-giant-eels/.
- ↑ 4.0 4.1 4.2 4.3 McClain, Craig (14 January 2015). "Why isn't the Giant Isopod larger?". http://www.deepseanews.com/2015/01/why-isnt-the-giant-isopod-larger/.
- ↑ Smithsonian Oceans. "Big Red Jellyfish". https://ocean.si.edu/ocean-life/invertebrates/big-red-jellyfish.
- ↑ Hoving, H. J. T.; Haddock, S. H. D. (2017-03-27). "The giant deep-sea octopus Haliphron atlanticus forages on gelatinous fauna". Scientific Reports 7: 44952. doi:10.1038/srep44952. PMID 28344325. Bibcode: 2017NatSR...744952H.
- ↑ 7.0 7.1 Anderton, Jim (22 February 2007). "Amazing specimen of world's largest squid in NZ". New Zealand Government. http://www.beehive.govt.nz/node/28451.
- ↑ 8.0 8.1 8.2 8.3 Gad, G. (2005). "Giant Higgins-larvae with paedogenetic reproduction from the deep sea of the Angola Basin? Evidence for a new life cycle and for abyssal gigantism in Loricifera?". Organisms Diversity & Evolution 5: 59–75. doi:10.1016/j.ode.2004.10.005.
- ↑ Bright, M.; Lallier, F. H. (2010). The biology of vestimentiferan tubeworms. Oceanography and Marine Biology - an Annual Review. 48. Taylor & Francis. pp. 213–266. doi:10.1201/ebk1439821169-c4. ISBN 978-1-4398-2116-9. http://www.sb-roscoff.fr/Ecchis/pdf/10-Bright-OMBAR.pdf. Retrieved 2013-10-30.
- ↑ Lutz, R. A.; Shank, T. M.; Fornari, D. J.; Haymon, R. M.; Lilley, M. D.; Von Damm, K. L.; Desbruyeres, D. (1994). "Rapid growth at deep-sea vents". Nature 371 (6499): 663. doi:10.1038/371663a0. Bibcode: 1994Natur.371..663L.
- ↑ MacDonald, Ian R. (2002). "Stability and Change in Gulf of Mexico Chemosynthetic Communities". MMS. http://www.data.boem.gov/PI/PDFImages/ESPIS/2/3071.pdf.
- ↑ Briones-Fourzán, Patricia; Lozano-Alvarez, Enrique (1991). "Aspects of the biology of the giant isopod Bathynomus giganteus A. Milne Edwards, 1879 (Flabellifera: Cirolanidae), off the Yucatan Peninsula". Journal of Crustacean Biology 11 (3): 375–385. doi:10.2307/1548464.
- ↑ Gallagher, Jack (2013-02-26). "Aquarium's deep-sea isopod hasn't eaten for over four years". The Japan Times. http://www.japantimes.co.jp/news/2013/02/26/national/tanks-deep-sea-isopod-hasnt-eaten-for-over-four-years.
- ↑ "I Won't Eat, You Can't Make Me! (And They Couldn't)". NPR. February 22, 2014. https://www.npr.org/blogs/krulwich/2014/02/22/280249001/i-wont-eat-you-cant-make-me-and-they-couldnt.
- ↑ Kleiber, M. (1947). "Body Size and Metabolic Rate". Physiological Reviews 27 (4): 511–541. doi:10.1152/physrev.1947.27.4.511. PMID 20267758.
- ↑ 16.0 16.1 Harper, E. M.; Peck, L. S. (2016). "Latitudinal and depth gradients in marine predation pressure". Global Ecology and Biogeography 25 (6): 670–678. doi:10.1111/geb.12444.
- ↑ 17.0 17.1 Chapelle, Gauthier; Peck, Lloyd S. (1999). "Polar gigantism dictated by oxygen availability" (in en). Nature 399 (6732): 114–115. doi:10.1038/20099. ISSN 0028-0836. Bibcode: 1999Natur.399..114C. http://www.nature.com/articles/20099.
- ↑ 18.0 18.1 Verberk, Wilco C. E. P.; Atkinson, David (2013). "Why polar gigantism and Palaeozoic gigantism are not equivalent: effects of oxygen and temperature on the body size of ectotherms". Functional Ecology 27 (6): 1275–1285. doi:10.1111/1365-2435.12152. ISSN 0269-8463. https://www.jstor.org/stable/24033996.
External links
![]() | Original source: https://en.wikipedia.org/wiki/Deep-sea gigantism.
Read more |