Biology:Overlapping gene
An overlapping gene (or OLG)[1][2] is a gene whose expressible nucleotide sequence partially overlaps with the expressible nucleotide sequence of another gene.[3] In this way, a nucleotide sequence may make a contribution to the function of one or more gene products. Overlapping genes are present in and a fundamental feature of both cellular and viral genomes.[2] The current definition of an overlapping gene varies significantly between eukaryotes, prokaryotes, and viruses.[2] In prokaryotes and viruses overlap must be between coding sequences but not mRNA transcripts, and is defined when these coding sequences share a nucleotide on either the same or opposite strands. In eukaryotes, gene overlap is almost always defined as mRNA transcript overlap. Specifically, a gene overlap in eukaryotes is defined when at least one nucleotide is shared between the boundaries of the primary mRNA transcripts of two or more genes, such that a DNA base mutation at any point of the overlapping region would affect the transcripts of all genes involved. This definition includes 5′ and 3′ untranslated regions (UTRs) along with introns.
Overprinting refers to a type of overlap in which all or part of the sequence of one gene is read in an alternate reading frame from another gene at the same locus.[4] The alternative open reading frames (ORF) are thought to be created by critical nucleotide substitutions within an expressible pre-existing gene, which can be induced to express a novel protein while still preserving the function of the original gene.[5] Overprinting has been hypothesized as a mechanism for de novo emergence of new genes from existing sequences, either older genes or previously non-coding regions of the genome.[6] It is believed that most overlapping genes, or genes whose expressible nucleotide sequences partially overlap with each other, evolved in part due to this mechanism, suggesting that each overlap is composed of one ancestral gene and one novel gene.[7] Subsequently, overprinting is also believed to be a source of novel proteins, as de novo proteins coded by these novel genes usually lack remote homologs in databases.[8] Overprinted genes are particularly common features of the genomic organization of viruses, likely to greatly increase the number of potential expressible genes from a small set of viral genetic information.[9] It is likely that overprinting is responsible for the generation of numerous novel proteins by viruses over the course of their evolutionary history.
Classification

Genes may overlap in a variety of ways and can be classified by their positions relative to each other.[3][11][12][13][14]
- Unidirectional or tandem overlap: the 3' end of one gene overlaps with the 5' end of another gene on the same strand. This arrangement can be symbolized with the notation → → where arrows indicate the reading frame from start to end.
- Convergent or end-on overlap: the 3' ends of the two genes overlap on opposite strands. This can be written as → ←.
- Divergent or tail-on overlap: the 5' ends of the two genes overlap on opposite strands. This can be written as ← →.
Overlapping genes can also be classified by phases, which describe their relative reading frames:[3][11][12][13][14]
- In-phase overlap occurs when the shared sequences use the same reading frame. This is also known as "phase 0". Unidirectional genes with phase 0 overlap are not considered distinct genes, but rather as alternative start sites of the same gene.
- Out-of-phase overlaps occurs when the shared sequences use different reading frames. This can occur in "phase 1" or "phase 2", depending on whether the reading frames are offset by 1 or 2 nucleotides. Because a codon is three nucleotides long, an offset of three nucleotides is an in-phase, phase 0 frame.
Studies on overlapping genes suggest that their evolution can be summarized in two possible models.[4] In one model, the two proteins encoded by their respective overlapping genes evolve under similar selection pressures. The proteins and the overlap region are highly conserved when strong selection against amino acid change is favored. Overlapping genes are reasoned to evolve under strict constraints as a single nucleotide substitution is able to alter the structure and function of the two proteins simultaneously. A study on the hepatitis B virus (HBV), whose DNA genome contains numerous overlapping genes, showed the mean number of synonymous nucleotide substitutions per site in overlapping coding regions was significantly lower than that of non-overlapping regions.[15] The same study showed that it was possible for some of these overlapping regions and their proteins to diverge significantly from the original when there's weak selection against amino acid change. The spacer domain of the polymerase and the pre-S1 region of a surface protein of HBV, for example, had a percentage of conserved amino acids of 30% and 40%, respectively.[15] However, these overlap regions are known to be less important for replication compared to the overlap regions that were highly conserved among different HBV strains, which are absolutely essential for the process.
The second model suggests that the two proteins and their respective overlap genes evolve under opposite selection pressures: one frame experiences positive selection while the other is under purifying selection. In tombusviruses, the proteins p19 and p22 are encoded by overlapping genes that form a 549 nt coding region, and p19 is shown to be under positive selection while p22 is under purifying selection.[16] Additional examples are mentioned in studies involving overlapping genes of the Sendai virus,[17] potato leafroll virus,[18] and human parvovirus B19.[19] This phenomenon of overlapping genes experiencing different selection pressures is suggested to be a consequence of a high rate of nucleotide substitution with different effects on the two frames; the substitutions may be majorly non-synonymous for one frame while mostly being synonymous for the other frame.[4]
Evolution
Overlapping genes are particularly common in rapidly evolving genomes, such as those of viruses, bacteria, and mitochondria. They may originate in three ways:[20]
- By extension of an existing open reading frame (ORF) downstream into a contiguous gene due to the loss of a stop codon;
- By extension of an existing ORF upstream into a contiguous gene due to loss of an initiation codon;
- By generation of a novel ORF within an existing one due to a point mutation.
The use of the same nucleotide sequence to encode multiple genes may provide evolutionary advantage due to reduction in genome size and due to the opportunity for transcriptional and translational co-regulation of the overlapping genes.[12][21][22][23] Gene overlaps introduce novel evolutionary constraints on the sequences of the overlap regions.[14][24]
Origins of new genes
In 1977, Pierre-Paul Grassé proposed that one of the genes in the pair could have originated de novo by mutations to introduce novel ORFs in alternate reading frames; he described the mechanism as overprinting.[25]:231 It was later substantiated by Susumu Ohno, who identified a candidate gene that may have arisen by this mechanism.[26] Some de novo genes originating in this way may not remain overlapping, but subfunctionalize following gene duplication,[6] contributing to the prevalence of orphan genes. Which member of an overlapping gene pair is younger can be identified bioinformatically either by a more restricted phylogenetic distribution, or by less optimized codon usage.[9][27][28] Younger members of the pair tend to have higher intrinsic structural disorder than older members, but the older members are also more disordered than other proteins, presumably as a way of alleviating the increased evolutionary constraints posed by overlap.[27] Overlaps are more likely to originate in proteins that already have high disorder.[27]
Taxonomic distribution
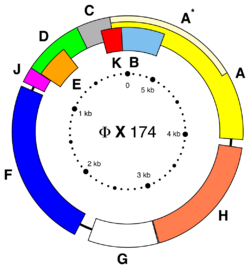
Overlapping genes occur in all domains of life, though with varying frequencies. They are especially common in viral genomes.
Viruses

The existence of overlapping genes was first identified in the virus ΦX174, whose genome was the first DNA genome ever sequenced by Frederick Sanger in 1977.[29] Previous analysis of ΦX174, a small single-stranded DNA bacteriophage that infected the bacteria Escherichia coli, suggested that the proteins produced during infection required coding sequences longer than the measured length of its genome.[31] Analysis of the fully sequenced 5386 nucleotide genome showed that the virus possessed extensive overlap between coding regions, revealing that some genes (like genes D and E) were translated from the same DNA sequences but in different reading frames.[29][31] An alternative start site within the genome replication gene A of ΦX174 was shown to express a truncated protein with an identical coding sequence to the C-terminus of the original A protein but possessing a different function[32][33] It was concluded that other undiscovered sites of polypeptide synthesis could be hidden through the genome due to overlapping genes. An identified de novo gene of another overlapping gene locus was shown to express a novel protein that induces lysis of E. coli by inhibiting biosynthesis of its cell wall[56], suggesting that de novo protein creation through the process of overprinting can be a significant factor in the evolution of pathogenicity of viruses.[4] Another example is the ORF3d gene in the SARS-CoV 2 virus.[1][34] Overlapping genes are particularly common in viral genomes.[9] Some studies attribute this observation to selective pressure toward small genome sizes mediated by the physical constraints of packaging the genome in a viral capsid, particularly one of icosahedral geometry.[35] However, other studies dispute this conclusion and argue that the distribution of overlaps in viral genomes is more likely to reflect overprinting as the evolutionary origin of overlapping viral genes.[36] Overprinting is a common source of de novo genes in viruses.[28]
The proportion of viruses with overlapping coding sequences within their genomes varies.[2] Double-stranded RNA viruses have fewer than a quarter that contains them while almost three-quarters of retroviridae and viruses with single-stranded DNA genomes contain overlapping coding sequences.[37] Segmented viruses in particular, or viruses with their genome split into separate pieces and packaged either all in the same capsid or in separate capsids, are more likely to contain an overlapping sequence than non-segmented viruses.[37] RNA viruses have fewer overlapping genes than DNA viruses which possess lower mutation rates and less restrictive genome sizes.[37][38] The lower mutation rate of DNA viruses facilitates greater genomic novelty and evolutionary exploration within a structurally constrained genome and may be the primary driver of the evolution of overlapping genes.[39][40]
Studies of overprinted viral genes suggest that their protein products tend to be accessory proteins which are not essential to viral proliferation, but contribute to pathogenicity. Overprinted proteins often have unusual amino acid distributions and high levels of intrinsic disorder.[41] In some cases overprinted proteins do have well-defined, but novel, three-dimensional structures;[42] one example is the RNA silencing suppressor p19 found in Tombusviruses, which has both a novel protein fold and a novel binding mode in recognizing siRNAs.[28][30][43]
Prokaryotes
Estimates of gene overlap in bacterial genomes typically find that around one third of bacterial genes are overlapped, though usually only by a few base pairs.[12][44][45] Most studies of overlap in bacterial genomes find evidence that overlap serves a function in gene regulation, permitting the overlapped genes to be transcriptionally and translationally co-regulated.[12][23] In prokaryotic genomes, unidirectional overlaps are most common, possibly due to the tendency of adjacent prokaryotic genes to share orientation.[12][14][11] Among unidirectional overlaps, long overlaps are more commonly read with a one-nucleotide offset in reading frame (i.e., phase 1) and short overlaps are more commonly read in phase 2.[45][46] Long overlaps of greater than 60 base pairs are more common for convergent genes; however, putative long overlaps have very high rates of misannotation.[47] Robustly validated examples of long overlaps in bacterial genomes are rare; in the well-studied model organism Escherichia coli, only four gene pairs are well validated as having long, overprinted overlaps.[48]
Eukaryotes
Compared to prokaryotic genomes, eukaryotic genomes are often poorly annotated and thus identifying genuine overlaps is relatively challenging.[28] However, examples of validated gene overlaps have been documented in a variety of eukaryotic organisms, including mammals such as mice and humans.[49][50][51][52] Eukaryotes differ from prokaryotes in distribution of overlap types: while unidirectional (i.e., same-strand) overlaps are most common in prokaryotes, opposite or antiparallel-strand overlaps are more common in eukaryotes. Among the opposite-strand overlaps, convergent orientation is most common.[50] Most studies of eukaryotic gene overlap have found that overlapping genes are extensively subject to genomic reorganization even in closely related species, and thus the presence of an overlap is not always well-conserved.[51][53] Overlap with older or less taxonomically restricted genes is also a common feature of genes likely to have originated de novo in a given eukaryotic lineage.[51][54][55]
Function
The precise functions of overlapping genes seems to vary across the domains of life but several experiments have shown that they are important for virus lifecycles through proper protein expression and stoichiometry [56] as well as playing a role in proper protein folding.[57] A version of bacteriophage ΦX174 has also been created where all gene overlaps were removed [58] proving they were not necessary for replication.
The retention and evolution of overlapping genes within viruses may also be due to capsid size limitations.[59] Dramatic viability loss was observed in viruses with genomes engineered to be longer than the wild-type genome.[60] Increasing the single-stranded DNA genome length of ΦX174 by >1% results in almost complete loss of infectivity, believed to be the result of the strict physical constraints imposed by the finite capsid volume.[61] Studies on adeno-associated viruses as gene delivery vectors showed that viral packaging is constrained by genetic cargo size limits, requiring the use of multiple vectors to deliver large human genes such as CFTR81.[62][63] Therefore, it is suggested that overlapping genes evolved as a means to overcome these physical constraints, increasing genetic diversity by utilizing only the existing sequence rather than increasing genome length.
Methods in identifying overlapping genes and ORFs
Standardized methods such as genome annotation may be inappropriate for the detection of overlapping genes as they are reliant on already curated genes while overlapping genes are generally overlooked contain atypical sequence composition.[2][64][65][66] Genome annotation standards are also often biased against feature overlaps, such as genes entirely contained within another gene.[67] Furthermore, some bioinformatics pipelines such as the RAST pipeline markedly penalizes overlaps between predicted ORFs.[68] However, rapid advancement of genome-scale protein and RNA measurement tools along with increasingly advanced prediction algorithms have revealed an avalanche of overlapping genes and ORFs within numerous genomes.[2] Proteogenomic methods have been essential in discovering numerous overlapping genes and include a combination of techniques such as bottom-up proteomics, ribosome profiling, DNA sequencing, and perturbation. RNA sequencing is also used to identify genomic regions containing overlapping transcripts. It has been utilized to identify 180,000 alternate ORFs within previously annotated coding regions found in humans.[69] Newly discovered ORFs such as these are verified using a variety of reverse genetics techniques, such as CRISPR-Cas9 and catalytically dead Cas9 (dCas9) disruption.[70][71][72] Attempts at proof-by-synthesis are also performed to show beyond doubt the absence of any undiscovered overlapping genes.[73]
See also
References
- ↑ 1.0 1.1 Nelson, Chase W (1 October 2020). "Dynamically evolving novel overlapping gene as a factor in the SARS-CoV-2 pandemic". eLife 9. doi:10.7554/eLife.59633. PMID 33001029.
- ↑ 2.0 2.1 2.2 2.3 2.4 2.5 Wright, Bradley W.; Molloy, Mark P.; Jaschke, Paul R. (2021-10-05). "Overlapping genes in natural and engineered genomes" (in en). Nature Reviews Genetics 23 (3): 154–168. doi:10.1038/s41576-021-00417-w. ISSN 1471-0064. PMID 34611352.
- ↑ 3.0 3.1 3.2 Y. Fukuda, M. Tomita et T. Washio (1999). "Comparative study of overlapping genes in the genomes of Mycoplasma genitalium and Mycoplasma pneumoniae". Nucleic Acids Res. 27 (8): 1847–1853. doi:10.1093/nar/27.8.1847. PMID 10101192.
- ↑ 4.0 4.1 4.2 4.3 Pavesi, Angelo (2021-05-26). "Origin, Evolution and Stability of Overlapping Genes in Viruses: A Systematic Review" (in en). Genes 12 (6): 809. doi:10.3390/genes12060809. ISSN 2073-4425. PMID 34073395.
- ↑ Normark, Staffan; Bergström, Sven; Edlund, Thomas; Grundström, Thomas; Jaurin, Bengtake; Lindberg, Frederik P.; Olsson, Olof (December 1983). "Overlapping Genes". Annual Review of Genetics 17 (1): 499–525. doi:10.1146/annurev.ge.17.120183.002435. ISSN 0066-4197. PMID 6198955. http://dx.doi.org/10.1146/annurev.ge.17.120183.002435.
- ↑ 6.0 6.1 Keese, PK; Gibbs, A (15 October 1992). "Origins of genes: "big bang" or continuous creation?". Proceedings of the National Academy of Sciences of the United States of America 89 (20): 9489–93. doi:10.1073/pnas.89.20.9489. PMID 1329098. Bibcode: 1992PNAS...89.9489K.
- ↑ Keese, P. K.; Gibbs, A. (1992-10-15). "Origins of genes: "big bang" or continuous creation?". Proceedings of the National Academy of Sciences 89 (20): 9489–9493. doi:10.1073/pnas.89.20.9489. ISSN 0027-8424. PMID 1329098. Bibcode: 1992PNAS...89.9489K.
- ↑ Gibbs, Adrian; Keese, Paul K. (1995-10-19), "In search of the origins of viral genes", Molecular Basis of Virus Evolution (Cambridge University Press): pp. 76–90, doi:10.1017/cbo9780511661686.008, ISBN 9780521455336, http://dx.doi.org/10.1017/cbo9780511661686.008, retrieved 2021-12-03
- ↑ 9.0 9.1 9.2 9.3 Pavesi, Angelo; Magiorkinis, Gkikas; Karlin, David G.; Wilke, Claus O. (15 August 2013). "Viral Proteins Originated De Novo by Overprinting Can Be Identified by Codon Usage: Application to the "Gene Nursery" of Deltaretroviruses". PLOS Computational Biology 9 (8): e1003162. doi:10.1371/journal.pcbi.1003162. PMID 23966842. Bibcode: 2013PLSCB...9E3162P.
- ↑ "Sequence and organization of the human mitochondrial genome". Nature 290 (5806): 457–465. April 1981. doi:10.1038/290457a0. PMID 7219534. Bibcode: 1981Natur.290..457A.
- ↑ 11.0 11.1 11.2 Fukuda, Yoko; Nakayama, Yoichi; Tomita, Masaru (December 2003). "On dynamics of overlapping genes in bacterial genomes". Gene 323: 181–187. doi:10.1016/j.gene.2003.09.021. PMID 14659892.
- ↑ 12.0 12.1 12.2 12.3 12.4 12.5 "Properties of overlapping genes are conserved across microbial genomes". Genome Res 14 (11): 2268–72. 2004. doi:10.1101/gr.2433104. PMID 15520290.
- ↑ 13.0 13.1 Normark S.; Bergstrom S.; Edlund T.; Grundstrom T.; Jaurin B.; Lindberg F.P.; Olsson O. (1983). "Overlapping genes". Annual Review of Genetics 17: 499–525. doi:10.1146/annurev.ge.17.120183.002435. PMID 6198955.
- ↑ 14.0 14.1 14.2 14.3 Rogozin, Igor B.; Spiridonov, Alexey N.; Sorokin, Alexander V.; Wolf, Yuri I.; Jordan, I.King; Tatusov, Roman L.; Koonin, Eugene V. (May 2002). "Purifying and directional selection in overlapping prokaryotic genes". Trends in Genetics 18 (5): 228–232. doi:10.1016/S0168-9525(02)02649-5. PMID 12047938.
- ↑ 15.0 15.1 Mizokami, Masashi; Orito, Etsuro; Ohba, Ken-ichi; Ikeo, Kazuho; Lau, Johnson Y. N.; Gojobori, Takashi (January 1997). "Constrained evolution with respect to gene overlap of hepatitis B virus". Journal of Molecular Evolution 44 (S1): S83–S90. doi:10.1007/pl00000061. ISSN 0022-2844. PMID 9071016. Bibcode: 1997JMolE..44S..83M. http://dx.doi.org/10.1007/pl00000061.
- ↑ Allison, Jane R.; Lechner, Marcus; Hoeppner, Marc P.; Poole, Anthony M. (2016-02-12). "Positive Selection or Free to Vary? Assessing the Functional Significance of Sequence Change Using Molecular Dynamics". PLOS ONE 11 (2): e0147619. doi:10.1371/journal.pone.0147619. ISSN 1932-6203. PMID 26871901. Bibcode: 2016PLoSO..1147619A.
- ↑ Fujii, Yutaka; Kiyotani, Katsuhiro; Yoshida, Tetsuya; Sakaguchi, Takemasa (2001). "Conserved and non-conserved regions in the Sendai virus genome: Evolution of a gene possessing overlapping reading frames". Virus Genes 22 (1): 47–52. doi:10.1023/a:1008130318633. ISSN 0920-8569. PMID 11210938. http://dx.doi.org/10.1023/a:1008130318633.
- ↑ Guyader, Sébastien; Ducray, Danièle Giblot (2002-07-01). "Sequence analysis of Potato leafroll virus isolates reveals genetic stability, major evolutionary events and differential selection pressure between overlapping reading frame products". Journal of General Virology 83 (7): 1799–1807. doi:10.1099/0022-1317-83-7-1799. ISSN 0022-1317. PMID 12075102.
- ↑ Stamenković, Gorana G.; Ćirković, Valentina S.; Šiljić, Marina M.; Blagojević, Jelena V.; Knežević, Aleksandra M.; Joksić, Ivana D.; Stanojević, Maja P. (2016-10-24). "Substitution rate and natural selection in parvovirus B19". Scientific Reports 6 (1): 35759. doi:10.1038/srep35759. ISSN 2045-2322. PMID 27775080. PMC 5075947. Bibcode: 2016NatSR...635759S. http://dx.doi.org/10.1038/srep35759.
- ↑ Krakauer, David C. (June 2000). "Stability and Evolution of Overlapping Genes". Evolution 54 (3): 731–739. doi:10.1111/j.0014-3820.2000.tb00075.x. PMID 10937248.
- ↑ Delaye, Luis; DeLuna, Alexander; Lazcano, Antonio; Becerra, Arturo (2008). "The origin of a novel gene through overprinting in Escherichia coli". BMC Evolutionary Biology 8 (1): 31. doi:10.1186/1471-2148-8-31. PMID 18226237.
- ↑ Saha, Deeya; Podder, Soumita; Panda, Arup; Ghosh, Tapash Chandra (May 2016). "Overlapping genes: A significant genomic correlate of prokaryotic growth rates". Gene 582 (2): 143–147. doi:10.1016/j.gene.2016.02.002. PMID 26853049.
- ↑ 23.0 23.1 Luo, Yingqin; Battistuzzi, Fabia; Lin, Kui; Gibas, Cynthia (29 November 2013). "Evolutionary Dynamics of Overlapped Genes in Salmonella". PLOS ONE 8 (11): e81016. doi:10.1371/journal.pone.0081016. PMID 24312259. Bibcode: 2013PLoSO...881016L.
- ↑ Wei, X.; Zhang, J. (31 December 2014). "A Simple Method for Estimating the Strength of Natural Selection on Overlapping Genes". Genome Biology and Evolution 7 (1): 381–390. doi:10.1093/gbe/evu294. PMID 25552532.
- ↑ Grassé, Pierre-Paul (1977). Evolution of Living Organisms: Evidence for a New Theory of Transformation. Academic Press. ISBN 9781483274096.
- ↑ Ohno, S (April 1984). "Birth of a unique enzyme from an alternative reading frame of the preexisted, internally repetitious coding sequence.". Proceedings of the National Academy of Sciences of the United States of America 81 (8): 2421–5. doi:10.1073/pnas.81.8.2421. PMID 6585807. Bibcode: 1984PNAS...81.2421O.
- ↑ 27.0 27.1 27.2 Willis, Sara; Masel, Joanna (19 July 2018). "Gene Birth Contributes to Structural Disorder Encoded by Overlapping Genes". Genetics 210 (1): 303–313. doi:10.1534/genetics.118.301249. PMID 30026186.
- ↑ 28.0 28.1 28.2 28.3 Sabath, N.; Wagner, A.; Karlin, D. (19 July 2012). "Evolution of Viral Proteins Originated De Novo by Overprinting". Molecular Biology and Evolution 29 (12): 3767–3780. doi:10.1093/molbev/mss179. PMID 22821011.
- ↑ 29.0 29.1 29.2 Sanger, F.; Air, G. M.; Barrell, B. G.; Brown, N. L.; Coulson, A. R.; Fiddes, J. C.; Hutchison, C. A.; Slocombe, P. M. et al. (1977). "Nucleotide sequence of bacteriophage ΦX174 DNA". Nature 265 (5596): 687–95. doi:10.1038/265687a0. PMID 870828. Bibcode: 1977Natur.265..687S.
- ↑ 30.0 30.1 Ye, Keqiong; Malinina, Lucy; Patel, Dinshaw J. (3 December 2003). "Recognition of small interfering RNA by a viral suppressor of RNA silencing". Nature 426 (6968): 874–878. doi:10.1038/nature02213. PMID 14661029. Bibcode: 2003Natur.426..874Y.
- ↑ 31.0 31.1 Barrell, B. G.; Air, G. M.; Hutchison, C. A. (November 1976). "Overlapping genes in bacteriophage φX174" (in en). Nature 264 (5581): 34–41. doi:10.1038/264034a0. ISSN 1476-4687. PMID 1004533. Bibcode: 1976Natur.264...34B. https://www.nature.com/articles/264034a0.
- ↑ LINNEY, ELWOOD; HAYASHI, MASAKI (May 1974). "Intragenic regulation of the synthesis of ΦX174 gene A proteins". Nature 249 (5455): 345–348. doi:10.1038/249345a0. ISSN 0028-0836. PMID 4601823. Bibcode: 1974Natur.249..345L. http://dx.doi.org/10.1038/249345a0.
- ↑ Roznowski, Aaron P.; Doore, Sarah M.; Kemp, Sundance Z.; Fane, Bentley A. (2020-01-06). "Finally, a Role Befitting A star : Strongly Conserved, Unessential Microvirus A* Proteins Ensure the Product Fidelity of Packaging Reactions". Journal of Virology 94 (2). doi:10.1128/jvi.01593-19. ISSN 0022-538X. PMID 31666371. PMC 6955274. http://dx.doi.org/10.1128/jvi.01593-19.
- ↑ Dockrill, Peter (11 November 2020). "Scientists Just Found a Mysteriously Hidden 'Gene Within a Gene' in SARS-CoV-2". ScienceAlert. https://www.sciencealert.com/scientists-find-mysterious-gene-within-gene-hidden-in-the-coronavirus-genome.
- ↑ Chirico, N.; Vianelli, A.; Belshaw, R. (7 July 2010). "Why genes overlap in viruses". Proceedings of the Royal Society B: Biological Sciences 277 (1701): 3809–3817. doi:10.1098/rspb.2010.1052. PMID 20610432.
- ↑ Brandes, Nadav; Linial, Michal (21 May 2016). "Gene overlapping and size constraints in the viral world". Biology Direct 11 (1): 26. doi:10.1186/s13062-016-0128-3. PMID 27209091.
- ↑ 37.0 37.1 37.2 Schlub, Timothy E; Holmes, Edward C (2020-01-01). "Properties and abundance of overlapping genes in viruses". Virus Evolution 6 (1): veaa009. doi:10.1093/ve/veaa009. ISSN 2057-1577. PMID 32071766. PMC 7017920. http://dx.doi.org/10.1093/ve/veaa009.
- ↑ Chirico, Nicola; Vianelli, Alberto; Belshaw, Robert (2010-07-07). "Why genes overlap in viruses". Proceedings of the Royal Society B: Biological Sciences 277 (1701): 3809–3817. doi:10.1098/rspb.2010.1052. ISSN 0962-8452. PMID 20610432. PMC 2992710. http://dx.doi.org/10.1098/rspb.2010.1052.
- ↑ Brandes, Nadav; Linial, Michal (2016-05-21). "Gene overlapping and size constraints in the viral world". Biology Direct 11 (1): 26. doi:10.1186/s13062-016-0128-3. ISSN 1745-6150. PMID 27209091.
- ↑ Pavesi, Angelo (July 2020). "New insights into the evolutionary features of viral overlapping genes by discriminant analysis". Virology 546: 51–66. doi:10.1016/j.virol.2020.03.007. ISSN 0042-6822. PMID 32452417. PMC 7157939. http://dx.doi.org/10.1016/j.virol.2020.03.007.
- ↑ Rancurel, C.; Khosravi, M.; Dunker, A. K.; Romero, P. R.; Karlin, D. (29 July 2009). "Overlapping Genes Produce Proteins with Unusual Sequence Properties and Offer Insight into De Novo Protein Creation". Journal of Virology 83 (20): 10719–10736. doi:10.1128/JVI.00595-09. PMID 19640978.
- ↑ Abroi, Aare (2015-12-01). "A protein domain-based view of the virosphere–host relationship". Biochimie 119: 231–243. doi:10.1016/j.biochi.2015.08.008. PMID 26296474.
- ↑ Vargason, Jeffrey M; Szittya, György; Burgyán, József; Hall, Traci M.Tanaka (December 2003). "Size Selective Recognition of siRNA by an RNA Silencing Suppressor". Cell 115 (7): 799–811. doi:10.1016/S0092-8674(03)00984-X. PMID 14697199. https://digitalcommons.georgefox.edu/bio_fac/10.
- ↑ Huvet, Maxime; Stumpf, Michael PH (2014-01-01). "Overlapping genes: a window on gene evolvability". BMC Genomics 15 (1): 721. doi:10.1186/1471-2164-15-721. ISSN 1471-2164. PMID 25159814.
- ↑ 45.0 45.1 Cock, Peter J. A.; Whitworth, David E. (19 March 2007). "Evolution of Gene Overlaps: Relative Reading Frame Bias in Prokaryotic Two-Component System Genes". Journal of Molecular Evolution 64 (4): 457–462. doi:10.1007/s00239-006-0180-1. PMID 17479344. Bibcode: 2007JMolE..64..457C.
- ↑ Fonseca, M. M.; Harris, D. J.; Posada, D. (5 November 2013). "Origin and Length Distribution of Unidirectional Prokaryotic Overlapping Genes". G3: Genes, Genomes, Genetics 4 (1): 19–27. doi:10.1534/g3.113.005652. PMID 24192837.
- ↑ Pallejà, Albert; Harrington, Eoghan D; Bork, Peer (2008). "Large gene overlaps in prokaryotic genomes: result of functional constraints or mispredictions?". BMC Genomics 9 (1): 335. doi:10.1186/1471-2164-9-335. PMID 18627618.
- ↑ Fellner, Lea; Simon, Svenja; Scherling, Christian; Witting, Michael; Schober, Steffen; Polte, Christine; Schmitt-Kopplin, Philippe; Keim, Daniel A. et al. (18 December 2015). "Evidence for the recent origin of a bacterial protein-coding, overlapping orphan gene by evolutionary overprinting". BMC Evolutionary Biology 15 (1): 283. doi:10.1186/s12862-015-0558-z. PMID 26677845.
- ↑ McLysaght, Aoife; Guerzoni, Daniele (31 August 2015). "New genes from non-coding sequence: the role of de novo protein-coding genes in eukaryotic evolutionary innovation". Philosophical Transactions of the Royal Society B: Biological Sciences 370 (1678): 20140332. doi:10.1098/rstb.2014.0332. PMID 26323763.
- ↑ 50.0 50.1 C. Sanna, W. Li et L. Zhang (2008). "Overlapping genes in the human and mouse genomes". BMC Genomics 9 (169): 169. doi:10.1186/1471-2164-9-169. PMID 18410680.
- ↑ 51.0 51.1 51.2 Makałowska, Izabela; Lin, Chiao-Feng; Hernandez, Krisitina (2007). "Birth and death of gene overlaps in vertebrates". BMC Evolutionary Biology 7 (1): 193. doi:10.1186/1471-2148-7-193. PMID 17939861.
- ↑ Veeramachaneni, V. (1 February 2004). "Mammalian Overlapping Genes: The Comparative Perspective". Genome Research 14 (2): 280–286. doi:10.1101/gr.1590904. PMID 14762064.
- ↑ Behura, Susanta K; Severson, David W (2013). "Overlapping genes of Aedes aegypti: evolutionary implications from comparison with orthologs of Anopheles gambiae and other insects". BMC Evolutionary Biology 13 (1): 124. doi:10.1186/1471-2148-13-124. PMID 23777277.
- ↑ Murphy, Daniel N.; McLysaght, Aoife; Carmel, Liran (21 November 2012). "De Novo Origin of Protein-Coding Genes in Murine Rodents". PLOS ONE 7 (11): e48650. doi:10.1371/journal.pone.0048650. PMID 23185269. Bibcode: 2012PLoSO...748650M.
- ↑ Knowles, D. G.; McLysaght, A. (2 September 2009). "Recent de novo origin of human protein-coding genes". Genome Research 19 (10): 1752–1759. doi:10.1101/gr.095026.109. PMID 19726446.
- ↑ Wright, Bradley W.; Ruan, Juanfang; Molloy, Mark P.; Jaschke, Paul R. (2020-11-20). "Genome Modularization Reveals Overlapped Gene Topology Is Necessary for Efficient Viral Reproduction" (in en). ACS Synthetic Biology 9 (11): 3079–3090. doi:10.1021/acssynbio.0c00323. ISSN 2161-5063. PMID 33044064. https://pubs.acs.org/doi/10.1021/acssynbio.0c00323.
- ↑ Pradhan, Prajakta; Li, Wen; Kaur, Parjit (January 2009). "Translational Coupling Controls Expression and Function of the DrrAB Drug Efflux Pump" (in en). Journal of Molecular Biology 385 (3): 831–842. doi:10.1016/j.jmb.2008.11.027. PMID 19063901. https://linkinghub.elsevier.com/retrieve/pii/S0022283608014642.
- ↑ Jaschke, Paul R.; Lieberman, Erica K.; Rodriguez, Jon; Sierra, Adrian; Endy, Drew (December 2012). "A fully decompressed synthetic bacteriophage øX174 genome assembled and archived in yeast". Virology 434 (2): 278–284. doi:10.1016/j.virol.2012.09.020. ISSN 0042-6822. PMID 23079106.
- ↑ Krakauer, D. C.; Plotkin, J. B. (2002-01-29). "Redundancy, antiredundancy, and the robustness of genomes". Proceedings of the National Academy of Sciences 99 (3): 1405–1409. doi:10.1073/pnas.032668599. ISSN 0027-8424. PMID 11818563. Bibcode: 2002PNAS...99.1405K.
- ↑ Feiss, Michael; Fisher, R.A.; Crayton, M.A.; Egner, Carol (March 1977). "Packaging of the bacteriophage λ chromosome: Effect of chromosome length". Virology 77 (1): 281–293. doi:10.1016/0042-6822(77)90425-1. ISSN 0042-6822. PMID 841861. http://dx.doi.org/10.1016/0042-6822(77)90425-1.
- ↑ Aoyama, A; Hayashi, M (September 1985). "Effects of genome size on bacteriophage phi X174 DNA packaging in vitro.". Journal of Biological Chemistry 260 (20): 11033–11038. doi:10.1016/s0021-9258(17)39144-5. ISSN 0021-9258. PMID 3161888.
- ↑ Wu, Zhijian; Yang, Hongyan; Colosi, Peter (January 2010). "Effect of Genome Size on AAV Vector Packaging". Molecular Therapy 18 (1): 80–86. doi:10.1038/mt.2009.255. ISSN 1525-0016. PMID 19904234. PMC 2839202. http://dx.doi.org/10.1038/mt.2009.255.
- ↑ Vaidyanathan, Sriram; Baik, Ron; Chen, Lu; Bravo, Dawn T.; Suarez, Carlos J.; Abazari, Shayda M.; Salahudeen, Ameen A.; Dudek, Amanda M. et al. (March 2021). "Targeted replacement of full-length CFTR in human airway stem cells by CRISPR-Cas9 for pan-mutation correction in the endogenous locus" (in en). Molecular Therapy 30 (1): 223–237. doi:10.1016/j.ymthe.2021.03.023. PMID 33794364.
- ↑ Willis, Sara; Masel, Joanna (2018-07-19). "Gene Birth Contributes to Structural Disorder Encoded by Overlapping Genes". Genetics 210 (1): 303–313. doi:10.1534/genetics.118.301249. ISSN 1943-2631. PMID 30026186. PMC 6116962. http://dx.doi.org/10.1534/genetics.118.301249.
- ↑ Pavesi, Angelo; Vianelli, Alberto; Chirico, Nicola; Bao, Yiming; Blinkova, Olga; Belshaw, Robert; Firth, Andrew; Karlin, David (2018-10-19). "Overlapping genes and the proteins they encode differ significantly in their sequence composition from non-overlapping genes". PLOS ONE 13 (10): e0202513. doi:10.1371/journal.pone.0202513. ISSN 1932-6203. PMID 30339683. Bibcode: 2018PLoSO..1302513P.
- ↑ Pavesi, Angelo; Magiorkinis, Gkikas; Karlin, David G. (2013-08-15). "Viral Proteins Originated De Novo by Overprinting Can Be Identified by Codon Usage: Application to the "Gene Nursery" of Deltaretroviruses". PLOS Computational Biology 9 (8): e1003162. doi:10.1371/journal.pcbi.1003162. ISSN 1553-7358. PMID 23966842. Bibcode: 2013PLSCB...9E3162P.
- ↑ Supplemental Information 2: NCBI genome database accession information (PDF file).. doi:10.7717/peerj.6447/supp-2.
- ↑ Ahmed, Niyaz (2009-03-27). Faculty Opinions recommendation of The RAST Server: rapid annotations using subsystems technology.. doi:10.3410/f.1157743.618965.
- ↑ Ben-Tal, Nir, ed (2017-06-23). Decision letter: Deep transcriptome annotation enables the discovery and functional characterization of cryptic small proteins. doi:10.7554/elife.27860.082.
- ↑ Bazzini, Ariel; Wu, Qiushuang (2020-03-06). Faculty Opinions recommendation of Pervasive functional translation of noncanonical human open reading frames.. doi:10.3410/f.737484924.793572056.
- ↑ Prensner, John R.; Enache, Oana M.; Luria, Victor; Krug, Karsten; Clauser, Karl R.; Dempster, Joshua M.; Karger, Amir; Wang, Li et al. (2021-01-28). "Noncanonical open reading frames encode functional proteins essential for cancer cell survival". Nature Biotechnology 39 (6): 697–704. doi:10.1038/s41587-020-00806-2. ISSN 1087-0156. PMID 33510483. PMC 8195866. http://dx.doi.org/10.1038/s41587-020-00806-2.
- ↑ Cao, Xiongwen; Khitun, Alexandra; Luo, Yang; Na, Zhenkun; Phoodokmai, Thitima; Sappakhaw, Khomkrit; Olatunji, Elizabeth; Uttamapinant, Chayasith et al. (2020-03-05). "Alt-RPL36 downregulates the PI3K-AKT-mTOR signaling pathway by interacting with TMEM24". Nature Communications 12 (1): 508. doi:10.1038/s41467-020-20841-6. PMID 33479206.
- ↑ Jaschke, Paul R.; Dotson, Gabrielle A.; Hung, Kay S.; Liu, Diane; Endy, Drew (2019-11-12). "Definitive demonstration by synthesis of genome annotation completeness". Proceedings of the National Academy of Sciences 116 (48): 24206–24213. doi:10.1073/pnas.1905990116. ISSN 0027-8424. PMID 31719208. Bibcode: 2019PNAS..11624206J.
![]() | Original source: https://en.wikipedia.org/wiki/Overlapping gene.
Read more |