Earth:Dolomite (rock)
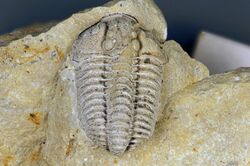
Dolomite (also known as dolomite rock, dolostone or dolomitic rock) is a sedimentary carbonate rock that contains a high percentage of the mineral dolomite, CaMg(CO3)2. In old USGS publications, it was referred to as magnesian limestone, a term now reserved for magnesium-deficient dolomites or magnesium-rich limestones. Dolomite has a stoichiometric ratio of nearly equal amounts of magnesium and calcium. Most dolomite rock formed as a magnesium replacement of limestone or lime mud before lithification.[1] Dolomite rock is resistant to erosion and can either contain bedded layers or be unbedded. It is less soluble than limestone in weakly acidic groundwater, but it can still develop solution features (karst) over time. Dolomite rock can act as an oil and natural gas reservoir.
The term dolostone was introduced in 1948 to avoid confusion with the mineral dolomite. The usage of the term dolostone is controversial because the name dolomite was first applied to the rock during the late 18th century and thus has technical precedence. The use of the term dolostone was not recommended by the Glossary of Geology published by the American Geological Institute.[2]
The geological process of conversion of calcite to dolomite is known as dolomitization and any intermediate product is known as "dolomitic limestone."[3]
The "dolomite problem" refers to the vast worldwide depositions of dolomite in the past geologic record eluding a unified explanation for their formation.
The first geologist to distinguish dolomite rock from limestone was Belsazar Hacquet in 1778.[4]
Caves in dolomite rock
As with limestone caves, natural caves and solution tubes typically form in dolomite rock as a result of the dissolution by weak carbonic acid.[5][6] Caves can also, less commonly, form through dissolution of rock by sulfuric acid.[7] Calcium carbonate speleothems (secondary deposits) in the forms of stalactites, stalagmites, flowstone etc., can also form in caves within dolomite rock. “Dolomite is a common rock type, but a relatively uncommon mineral in speleothems”.[5] Both the 'Union Internationale de Spéléologie' (UIS) and the American 'National Speleological Society' (NSS), extensively use in their publications, the terms "dolomite" or "dolomite rock" when referring to the natural bedrock containing a high percentage of CaMg(CO3)2 in which natural caves or solution tubes have formed.[5][8]
Dolomite speleothems
Both calcium and magnesium go into solution when dolomite rock is dissolved. The speleothem precipitation sequence is: calcite, Mg-calcite, aragonite, huntite and hydromagnesite.[5][8] Hence, the most common speleothem (secondary deposit) in caves within dolomite rock karst, is calcium carbonate in the most stable polymorph form of calcite. Speleothem types known to have a dolomite constituent include: coatings, crusts, moonmilk, flowstone, coralloids, powder, spar and rafts.[5] Although there are reports of dolomite speleothems known to exist in a number of caves around the world, they are usually in relatively small quantities and form in very fine-grained deposits.[5][8]
Physico-chemical importance of Mg2+ ion
Already studied by Bischoff (1968),[9] magnesium ion is a long known inhibitor of calcite nucleation and crystal growth.[10][11][12][13]
The Mg2+ cation is the second-most abundant cation present in seawater after Na+, as SO2–4 is the second anion after Cl−. Although closely resembling Ca2+, the ionic radius of the naked Mg2+ cation is smaller. Some paradoxical consequences arise between its contrasted electrostatic behavior in the mineral solid phase and in aqueous solution. The smaller magnesium ion presents a higher ionic potential than the larger calcium ion.
The ionic potential is the ratio of the electric charge to the ionic radius.[14] This ratio represents the charge density at the surface of a given ion. Denser the charge density, stronger the bond that the ion forms.[15] As Mg2+ ionic potential is greater than this of Ca2+ , it exerts a greater Coulomb interaction with its neighboring anions in the solid phase, or with water molecules in solution.
As a consequence, the lattice energy of magnesite (MgCO3) is higher than that of calcite (CaCO3) and therefore the solubility of MgCO3 is lower than that of CaCO3 because it requires more energy to separate the ions of opposite charge present in the crystal lattice.
Similarly, in aqueous solution, the intermolecular forces (IMF) due to the ion-dipole interactions between Mg2+ and the immediately surrounding H2O molecules are also stronger than for Ca2+. The Mg2+ ion is hexahydrated in aqueous solution and presents an octahedral configuration. The [Mg(H2O)6]2+ species has a much larger hydrated ionic radius than the naked Mg2+ ions and sorbs onto the negatively charged surface of calcite. Because of the larger ion-ion distance imposed by this larger hydrated ionic radius, the Coulomb interactions with CO2−3 anions present both in the crystal lattice of the solid calcite and in aqueous solution are weaker. Further calcite crystal growth first requires the dehydration of this [Mg(H2O)6]2+ species, which is an energetically unfavorable process. This explains why the Mg2+ cation can act as an inhibitor of calcite crystal growth if present at sufficiently high concentration in aqueous solution. The same effect of crystal growth inhibition is also observed with the Zn2+ cation whose ionic radius is similar to this of Mg2+.[16]
When the Mg2+ cation is incorporated at low concentration in the crystal lattice of calcite in chemical equilibrium with seawater, it also stabilizes the electron-hole centers observable in electron spin resonance (ESR) spectrometry (characteristic ESR line at g = 2.0006 – 2.0007) as evidenced by Barabas et al. (1988, 1992) and others when attempting to unravel the nature of the paramagnetic centers used to date corals and foraminifera in deep sea sediments.[17] [18] [19] [20]
See also
References
- ↑ Zenger & Mazzullo (1982). Dolomitization.
- ↑ Neuendorf, K.K.E.; Mehl, Jr., J.P.; Jackson, J.A. (editors) (2005). Glossary of Geology (5th edition). Alexandria, Virginia: American Geological Institute. p. 189. ISBN 978-0922152896.
- ↑ "Dolomite. A sedimentary rock known as dolostone or dolomite rock". Geology.com. http://geology.com/rocks/dolomite.shtml. Retrieved 20 June 2014.
- ↑ Kranjc, Andrej (2006). "Balthasar Hacquet (1739/40-1815), the Pioneer of Karst Geomorphologists". Acta Carsologica (Institute for the Karst Research, Scientific Research Centre, Slovenian Academy of Sciences and Arts) 35 (2). ISSN 0583-6050. Archived from the original on 31 December 2016. https://web.archive.org/web/20161231234811/http://www.dlib.si/details/URN:NBN:SI:DOC-XBSU59HS/?&language=eng.
- ↑ 5.0 5.1 5.2 5.3 5.4 5.5 Hill, C A and Forti, P, (1997). Cave Minerals of the World, Second editions. [Huntsville, Alabama: National Speleological Society Inc.] pp 14, 142, 143, 144 & 150, ISBN:1-879961-07-5
- ↑ White W.B and Culver D.C., (2005) Chapter "Caves, Definitions of", Encyclopedia of Caves, edited by Culver D.C and White W.B., ISBN:0-12-406061-7
- ↑ Polyak, Victor J.; Provencio, Paula (2000). "By-product materials relatied to H2S-H2SO4-influenced speleogenesis of Carlsbad, Lechuguilla, and other caves of the Guadalupe Mountains, New Mexico". Journal of Cave and Karst Studies 63 (1): 23–32. https://www.researchgate.net/publication/242359913_By-product_materials_related_to_H2S-H2SO4_influenced_speleogenesis_of_Carlsbad_Lechuguilla_and_other_caves_of_the_Guadalupe_mountains_New_Mexico. Retrieved 4 April 2020.
- ↑ 8.0 8.1 8.2 Encyclopedia of Caves, (2005). Edited by Culver D.C and White W.B., ISBN:0-12-406061-7
- ↑ Bischoff, James L. (1968). "Kinetics of calcite nucleation: Magnesium ion inhibition and ionic strength catalysis". Journal of Geophysical Research 73 (10): 3315–3322. doi:10.1029/JB073i010p03315. ISSN 01480227.
- ↑ Katz, Amitai (1973). "The interaction of magnesium with calcite during crystal growth at 25–90°C and one atmosphere". Geochimica et Cosmochimica Acta 37 (6): 1563–1586. doi:10.1016/0016-7037(73)90091-4. ISSN 00167037.
- ↑ Berner, R.A. (1975). "The role of magnesium in the crystal growth of calcite and aragonite from sea water". Geochimica et Cosmochimica Acta 39 (4): 489–504. doi:10.1016/0016-7037(75)90102-7. ISSN 00167037.
- ↑ Nielsen, M. R.; Sand, K. K.; Rodriguez-Blanco, J. D.; Bovet, N.; Generosi, J.; Dalby, K. N.; Stipp, S. L. S. (2016). "Inhibition of calcite growth: Combined effects of Mg2+ and SO2–4". Crystal Growth & Design 16 (11): 6199–6207. doi:10.1021/acs.cgd.6b00536. ISSN 1528-7483.
- ↑ Dobberschütz, S.; Nielsen, M. R.; Sand, K. K.; Civioc, R.; Bovet, N.; Stipp, S. L. S.; Andersson, M. P. (2018). "The mechanisms of crystal growth inhibition by organic and inorganic inhibitors". Nature Communications 9 (1). doi:10.1038/s41467-018-04022-0. ISSN 2041-1723.
- ↑ "Ionic potential". http://www.encyclopedia.com/science/dictionaries-thesauruses-pictures-and-press-releases/ionic-potential.
- ↑ "Ionic potential". http://www.gly.uga.edu/railsback/Fundamentals/IonicPotential04P.pdf.
- ↑ Ghizellaoui, S.; Euvrard, M. (2008). "Assessing the effect of zinc on the crystallization of calcium carbonate". Desalination 220 (1-3): 394–402. doi:10.1016/j.desal.2007.02.044. ISSN 00119164.
- ↑ Barabas, M.; Bach, A.; Mangini, A. (1988). "An analytical model for the growth of ESR signals". International Journal of Radiation Applications and Instrumentation. Part D. Nuclear Tracks and Radiation Measurements 14 (1-2): 231–235. doi:10.1016/1359-0189(88)90070-2. ISSN 13590189.
- ↑ Barabas, Michael; Bach, Andreas; Mudelsee, Manfred; Mangini, Augusto (1992). "General properties of the paramagnetic centre at g = 2.0006 in carbonates". Quaternary Science Reviews 11 (1-2): 165–171. doi:10.1016/0277-3791(92)90059-H. ISSN 02773791.
- ↑ Radtke, Ulrich; Grün, Rainer (1988). "ESR dating of corals". Quaternary Science Reviews 7 (3-4): 465–470. doi:10.1016/0277-3791(88)90047-9. ISSN 02773791.
- ↑ Barabas, Michael; Mudelsee, Manfred; Walther, Ralf; Mangini, Augusto (1992). "Dose-response and thermal behaviour of the ESR signal at g = 2.0006 in carbonates". Quaternary Science Reviews 11 (1-2): 173–179. doi:10.1016/0277-3791(92)90060-L. ISSN 02773791.
Further reading
- Blatt, Harvey; Tracy, Robert J. (1996). Petrology; Igneous, Sedimentary, and Metamorphic (2nd ed.). W. H. Freeman. pp. 317–323. ISBN 0-7167-2438-3.
- Tucker, M. E.; V. P., Wright (1990). Carbonate Sedimentology. Blackwell Scientific Publications. ISBN 0-632-01472-5.
- Zenger, D. H.; Mazzullo, S. J. (1982). Dolomitization. Hutchinson Ross. ISBN 0-87933-416-9.
External links