Physics:Scanning Kelvin probe
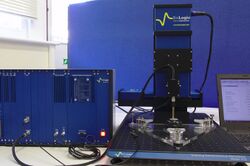
In microscopy, a scanning Kelvin probe (SKP) is a non-contact, non-destructive scanning probe microscopy (SPM) technique used to measure the work function of the sample under study. By raster scanning in the x,y plane the work function of the sample can be locally mapped for correlation with sample features. It is predominantly used to measure corrosion and coatings. It is closely related to the Kelvin probe force microscope (KPFM) technique.
History
The SKP technique is based on parallel plate capacitor experiments performed by Lord Kelvin in 1898.[1] In the 1930s William Zisman built upon Lord Kelvin's experiments to develop a technique to measure contact potential differences of dissimilar metals.[2]
Principle of Operation
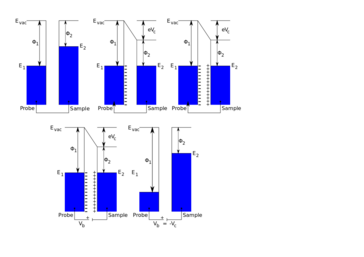
In SKP the probe and sample are held parallel to each other and electrically connected to form a parallel plate capacitor. The probe is selected to be of a different material to the sample, therefore each component initially has a distinct Fermi level. When electrical connection is made between the probe and the sample electron flow can occur between the probe and the sample in the direction of the lower to the higher Fermi level. This electron flow causes the equilibration of the probe and sample Fermi levels. Furthermore, a surface charge develops on the probe and the sample, with a related potential difference known as the contact potential (Vc).[3] In SKP the probe is vibrated in the plane perpendicular to the plane of the sample.[4] This vibration causes a change in probe to sample distance, which in turn results in the flow of current, taking the form of an ac sine wave. The resulting ac sine wave is demodulated to a dc signal through the use of a lock-in amplifier.[5] Typically the user must select the correct reference phase value used by the lock-in amplifier. Once the dc potential has been determined, an external potential, known as the backing potential (Vb) can be applied to null the charge between the probe and the sample. When the charge is nulled the Fermi level of the sample returns to its original position. This means that Vb is equal to -Vc, which is the work function difference between the SKP probe and the sample measured.[6]
Factors affecting SKP measurements
The quality of an SKP measurement is affected by a number of factors. This includes the diameter of the SKP probe, the probe to sample distance, and the material of the SKP probe. The probe diameter is important in the SKP measurement because it affects the overall resolution of the measurement, with smaller probes leading to improved resolution.[7][8] On the other hand, reducing the size of the probe causes an increase in fringing effects which reduces the sensitivity of the measurement by increasing the measurement of stray capacitances.[9] The material used in the construction of the SKP probe is important to the quality of the SKP measurement.[10] This occurs for a number of reasons. Different materials have different work function values which will affect the contact potential measured. Different materials have a different sensitivity to humidity changes. The material can also affect the resulting lateral resolution of the SKP measurement. In commercial probes tungsten is used,[11] though probes of platinum,[12] copper,[13] gold,[14] and NiCr have been used.[15] The probe to sample distance affects the final SKP measurement, with smaller probe to sample distances improving the lateral resolution [8] and the signal-to-noise ratio of the measurement.[16] Furthermore, reducing the SKP probe to sample distance increases the intensity of the measurement, where the intensity of the measurement is proportional to 1/d2, where d is the probe to sample distance.[17] The effects of changing probe to sample distance on the measurement can be counteracted by using SKP in constant distance mode.
Applications
The Volta potential measured by SKP is directly proportional to the corrosion potential of a material,[18] as such SKP has found widespread use in the study of the fields of corrosion and coatings. In the field of coatings for example, a scratched region of a self-healing shape memory polymer coating containing a heat generating agent on aluminium alloys was measured by SKP.[19] Initially after the scratch was made the Volta potential was noticeably higher and wider over the scratch than over the rest of the sample, implying this region is more likely to corrode. The Volta potential decreased over subsequent measurements, and eventually the peak over the scratch completely disappeared implying the coating has healed. Because SKP can be used to investigate coatings in a non-destructive way it has also been used to determine coating failure. In a study of polyurethane coatings, it was seen that the work function increases with increasing exposure to high temperature and humidity.[20] This increase in work function is related to decomposition of the coating likely from hydrolysis of bonds within the coating.
Using SKP the corrosion of industrially important alloys has been measured.[21] In particular with SKP it is possible to investigate the effects of environmental stimulus on corrosion. For example, the microbially induced corrosion of stainless steel and titanium has been examined.[22] SKP is useful to study this sort of corrosion because it usually occurs locally, therefore global techniques are poorly suited. Surface potential changes related to increased localized corrosion were shown by SKP measurements. Furthermore, it was possible to compare the resulting corrosion from different microbial species. In another example SKP was used to investigate biomedical alloy materials, which can be corroded within the human body. In studies on Ti-15Mo under inflammatory conditions,[23] SKP measurements showed a lower corrosion resistance at the bottom of a corrosion pit than at the oxide protected surface of the alloy. SKP has also been used to investigate the effects of atmospheric corrosion, for example to investigate copper alloys in marine environment.[24] In this study Kelvin potentials became more positive, indicating a more positive corrosion potential, with increased exposure time, due to an increase in thickness of corrosion products. As a final example SKP was used to investigate stainless steel under simulated conditions of gas pipeline.[25] These measurements showed an increase in difference in corrosion potential of cathodic and anodic regions with increased corrosion time, indicating a higher likelihood of corrosion. Furthermore, these SKP measurements provided information about local corrosion, not possible with other techniques.
SKP has been used to investigate the surface potential of materials used in solar cells, with the advantage that it is a non-contact, and therefore a non-destructive technique.[26] It can be used to determine the electron affinity of different materials in turn allowing the energy level overlap of conduction bands of differing materials to be determined. The energy level overlap of these bands is related to the surface photovoltage response of a system.[27]
As a non-contact, non-destructive technique SKP has been used to investigate latent fingerprints on materials of interest for forensic studies.[28] When fingerprints are left on a metallic surface they leave behind salts which can cause the localized corrosion of the material of interest. This leads to a change in Volta potential of the sample, which is detectable by SKP. SKP is particularly useful for these analyses because it can detect this change in Volta potential even after heating, or coating by, for example, oils.
SKP has been used to analyze the corrosion mechanisms of schreibersite-containing meteorites.[29][30] The aim of these studies has been to investigate the role in such meteorites in releasing species utilized in prebiotic chemistry.
In the field of biology SKP has been used to investigate the electric fields associated with wounding,[31] and acupuncture points.[32]
References
- ↑ Kelvin, Lord (1898). "V. Contact electricity of metals" (in en). The London, Edinburgh, and Dublin Philosophical Magazine and Journal of Science 46 (278): 82–120. doi:10.1080/14786449808621172. ISSN 1941-5982. https://zenodo.org/record/1653410.
- ↑ Zisman, W. A. (1932). "A NEW METHOD OF MEASURING CONTACT POTENTIAL DIFFERENCES IN METALS" (in en). Review of Scientific Instruments 3 (7): 367–370. doi:10.1063/1.1748947. ISSN 0034-6748.
- ↑ Stein, John (1970). "An Improved Method for Constructing Electrostatic Prisms". Review of Scientific Instruments 41 (3): 477. doi:10.1063/1.1684557. ISSN 0034-6748.
- ↑ Rohwerder, Michael; Turcu, Florin (2007). "High-resolution Kelvin probe microscopy in corrosion science: Scanning Kelvin probe force microscopy (SKPFM) versus classical scanning Kelvin probe (SKP)" (in en). Electrochimica Acta 53 (2): 290–299. doi:10.1016/j.electacta.2007.03.016.
- ↑ Cheran, Larisa-Emilia; Johnstone, Sherri; Sadeghi, Saman; Thompson, Michael (2007-01-19). "Work-function measurement by high-resolution scanning Kelvin nanoprobe". Measurement Science and Technology 18 (3): 567–578. doi:10.1088/0957-0233/18/3/005. ISSN 0957-0233.
- ↑ Surplice, N A; D'Arcy, R J (1970). "A critique of the Kelvin method of measuring work functions". Journal of Physics E: Scientific Instruments 3 (7): 477–482. doi:10.1088/0022-3735/3/7/201. ISSN 0022-3735.
- ↑ Wicinski, Mariusz; Burgstaller, Wolfgang; Hassel, Achim Walter (2016). "Lateral resolution in scanning Kelvin probe microscopy" (in en). Corrosion Science 104: 1–8. doi:10.1016/j.corsci.2015.09.008.
- ↑ 8.0 8.1 McMurray, H. N.; Williams, G. (2002). "Probe diameter and probe–specimen distance dependence in the lateral resolution of a scanning Kelvin probe" (in en). Journal of Applied Physics 91 (3): 1673–1679. doi:10.1063/1.1430546. ISSN 0021-8979.
- ↑ Wicinski, Mariusz; Burgstaller, Wolfgang; Hassel, Achim Walter (2016). "Lateral resolution in scanning Kelvin probe microscopy" (in en). Corrosion Science 104: 1–8. doi:10.1016/j.corsci.2015.09.008.
- ↑ Huber, Silvia; Wicinski, Mariusz; Hassel, Achim Walter (2018). "Suitability of Various Materials for Probes in Scanning Kelvin Probe Measurements" (in en). Physica Status Solidi A 215 (15): 1700952. doi:10.1002/pssa.201700952.
- ↑ "High Resolution Scanning Kelvin Probe" (in en-GB). https://www.bio-logic.net/accessories/probes/hr-skp/.
- ↑ Hansen, Douglas C.; Hansen, Karolyn M.; Ferrell, Thomas L.; Thundat, Thomas (2003). "Discerning Biomolecular Interactions Using Kelvin Probe Technology". Langmuir 19 (18): 7514–7520. doi:10.1021/la034333w. ISSN 0743-7463.
- ↑ Dirscherl, Konrad; Baikie, Iain; Forsyth, Gregor; Heide, Arvid van der (2003). "Utilisation of a micro-tip scanning Kelvin probe for non-invasive surface potential mapping of mc-Si solar cells" (in en). Solar Energy Materials and Solar Cells 79 (4): 485–494. doi:10.1016/S0927-0248(03)00064-3.
- ↑ Stratmann, M. (1987). "The investigation of the corrosion properties of metals, covered with adsorbed electrolyte layers—A new experimental technique" (in en). Corrosion Science 27 (8): 869–872. doi:10.1016/0010-938X(87)90043-6.
- ↑ Nazarov, A. P.; Thierry, D. (2001). "Study of the Carbon Steel/Alkyd Coating Interface with a Scanning Vibrating Capacitor Technique". Protection of Metals 37 (2): 108–119. doi:10.1023/a:1010361702449. ISSN 0033-1732.
- ↑ "Height tracking with the SKP370 or SKP470 module". https://www.bio-logic.net/wp-content/uploads/SCANLAB-AN-1.pdf.
- ↑ Wapner, K.; Schoenberger, B.; Stratmann, M.; Grundmeier, G. (2005). "Height-Regulating Scanning Kelvin Probe for Simultaneous Measurement of Surface Topology and Electrode Potentials at Buried Polymer/Metal Interfaces" (in en). Journal of the Electrochemical Society 152 (3): E114. doi:10.1149/1.1856914.
- ↑ "SKP imaging example of a corroded Zn-plated Fe sample.". https://www.bio-logic.net/wp-content/uploads/SCANLAB-AN-9-SKP.pdf.
- ↑ Fan, Weijie; Zhang, Yong; Li, Weihua; Wang, Wei; Zhao, Xiaodong; Song, Liying (2019). "Multi-level self-healing ability of shape memory polyurethane coating with microcapsules by induction heating" (in en). Chemical Engineering Journal 368: 1033–1044. doi:10.1016/j.cej.2019.03.027.
- ↑ Borth, David J.; Iezzi, Erick B.; Dudis, Douglas S.; Hansen, Douglas C. (2019). "Nondestructive Evaluation of Urethane-Ester Coating Systems Using the Scanning Kelvin Probe Technique" (in en). Corrosion 75 (5): 457–464. doi:10.5006/3020. ISSN 0010-9312. http://rave.ohiolink.edu/etdc/view?acc_num=dayton1524749968517527.
- ↑ Zhou, Herong (2017). "Corrosion Behavior of the Al2Cu Intermetallic Compound and Coupled Al2Cu/Al". International Journal of Electrochemical Science: 9542–9554. doi:10.20964/2017.10.32. http://www.electrochemsci.org/abstracts/vol12/121009542.pdf.
- ↑ Zhang, Dawei; Zhou, Feichi; Xiao, Kui; Cui, Tianyu; Qian, Hongchong; Li, Xiaogang (2015). "Microbially Influenced Corrosion of 304 Stainless Steel and Titanium by P. variotii and A. niger in Humid Atmosphere" (in en). Journal of Materials Engineering and Performance 24 (7): 2688–2698. doi:10.1007/s11665-015-1558-2. ISSN 1059-9495.
- ↑ Szklarska, M.; Dercz, G.; Kubisztal, J.; Balin, K.; Łosiewicz, B. (2016). "Semi-Conducting Properties of Titanium Dioxide Layer on Surface of Ti-15Mo Implant Alloy in Biological Milieu" (in en). Acta Physica Polonica A 130 (4): 1085–1087. doi:10.12693/APhysPolA.130.1085. ISSN 0587-4246.
- ↑ Kong, Decheng; Dong, Chaofang; Ni, Xiaoqing; Man, Cheng; Xiao, Kui; Li, Xiaogang (2018). "Insight into the mechanism of alloying elements (Sn, Be) effect on copper corrosion during long-term degradation in harsh marine environment" (in en). Applied Surface Science 455: 543–553. doi:10.1016/j.apsusc.2018.06.029.
- ↑ Jin, Z.H.; Ge, H.H.; Lin, W.W.; Zong, Y.W.; Liu, S.J.; Shi, J.M. (2014). "Corrosion behaviour of 316L stainless steel and anti-corrosion materials in a high acidified chloride solution" (in en). Applied Surface Science 322: 47–56. doi:10.1016/j.apsusc.2014.09.205.
- ↑ Dirscherl, Konrad; Baikie, Iain; Forsyth, Gregor; Heide, Arvid van der (2003). "Utilisation of a micro-tip scanning Kelvin probe for non-invasive surface potential mapping of mc-Si solar cells". Solar Energy Materials and Solar Cells 79 (4): 485–494. doi:10.1016/s0927-0248(03)00064-3. ISSN 0927-0248.
- ↑ Liu, Xiangyang; Zheng, Haiwu; Zhang, Jiwei; Xiao, Yin; Wang, Zhiyong (2013). "Photoelectric properties and charge dynamics for a set of solid state solar cells with Cu4Bi4S9 as the absorber layer" (in en). Journal of Materials Chemistry A 1 (36): 10703. doi:10.1039/c3ta11830d. ISSN 2050-7488.
- ↑ Williams, Geraint; McMurray, H. N. (2008). "Human Fingerprint - Metal Interactions Studied Using a Scanning Kelvin Probe" (in en). ECS Transactions (Washington, DC: ECS) 11: 81–89. doi:10.1149/1.2925265.
- ↑ Bryant, David E.; Greenfield, David; Walshaw, Richard D.; Evans, Suzanne M.; Nimmo, Alexander E.; Smith, Caroline L.; Wang, Liming; Pasek, Matthew A. et al. (2009). "Electrochemical studies of iron meteorites: phosphorus redox chemistry on the early Earth" (in en). International Journal of Astrobiology 8 (1): 27–36. doi:10.1017/S1473550408004345. ISSN 1473-5504.
- ↑ Bryant, David E.; Greenfield, David; Walshaw, Richard D.; Johnson, Benjamin R.G.; Herschy, Barry; Smith, Caroline; Pasek, Matthew A.; Telford, Richard et al. (2013). "Hydrothermal modification of the Sikhote-Alin iron meteorite under low pH geothermal environments. A plausibly prebiotic route to activated phosphorus on the early Earth" (in en). Geochimica et Cosmochimica Acta 109: 90–112. doi:10.1016/j.gca.2012.12.043.
- ↑ Nuccitelli, Richard; Nuccitelli, Pamela; Ramlatchan, Samdeo; Sanger, Richard; Smith, Peter J.S. (2008). "Imaging the electric field associated with mouse and human skin wounds" (in en). Wound Repair and Regeneration 16 (3): 432–441. doi:10.1111/j.1524-475X.2008.00389.x. ISSN 1067-1927. PMID 18471262.
- ↑ Gow, Brian J.; Cheng, Justine L.; Baikie, Iain D.; Martinsen, Ørjan G.; Zhao, Min; Smith, Stephanie; Ahn, Andrew C. (2012). "Electrical Potential of Acupuncture Points: Use of a Noncontact Scanning Kelvin Probe" (in en). Evidence-Based Complementary and Alternative Medicine 2012: 632838. doi:10.1155/2012/632838. ISSN 1741-427X. PMID 23320033.