Biology:Biosequestration
Biosequestration or biological sequestration is the capture and storage of the atmospheric greenhouse gas carbon dioxide by continual or enhanced biological processes.
This form of carbon sequestration occurs through increased rates of photosynthesis via land-use practices such as reforestation, sustainable forest management, and genetic engineering.[1][2] Methods and practices exist to enhance soil carbon sequestration in both sectors of agriculture and forestry. Additionally, in the context of industrial energy production, strategies such as Bio-energy with Carbon Capture and Storage to absorb carbon dioxide emissions from coal, petroleum, or natural gas-fired electricity generation can utilize an alternative of algal bio sequestration (see algae bioreactor).[3]
Biosequestration as a natural process has occurred in the past, and was responsible for the formation of the extensive coal and oil deposits which are now being burned. It is a key policy concept in the climate change mitigation debate.[4] It does not generally refer to the sequestering of carbon dioxide in oceans (see carbon sequestration and ocean acidification) or rock formations (see geological sequestration), depleted oil or gas reservoirs (see oil depletion and peak oil), deep saline aquifers, or deep coal seams (see coal mining) (for all see geosequestration) or through the use of industrial chemical carbon dioxide scrubbing.
Carbon sequestration in plants
Water vapour is the most abundant greenhouse gas in the atmosphere. Carbon dioxide is a significant temperature independent but seasonally dependent green house gas (methane rapidly reacts to form water vapour and carbon dioxide). Average atmospheric carbon dioxide concentrations have increased from about 280 ppm in 1750 to 416 ppm in 2022, now increasing at an average rate of 2 ppm pr year.[5][6] The world's oceans have previously played an important role in sequestering atmospheric carbon dioxide through solubility and the photosynthesis by phytoplankton.[7] Considering the adverse consequences of ocean acidification, global warming, and climate change on humanity, recent research and policy mechanisms have considered bio sequestration through plants.
Reforestation, avoided deforestation and LULUCF
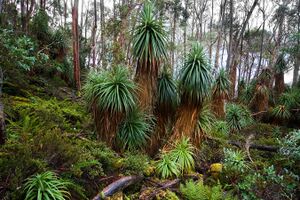
The Intergovernmental Panel on Climate Change (IPCC) estimates that the cutting down of forests is now contributing close to 20 per cent of the overall greenhouse gases entering the atmosphere.[8] Candell and Raupach argue that there are four primary ways in which reforestation and reducing deforestation can increase biosequestration. First, by increasing the volume of existing forest. Second, by increasing the carbon density of existing forests at a stand and landscape scale. Third, by expanding the use of forest products that will sustainably replace fossil-fuel emissions. Fourth, by reducing carbon emissions that are caused from deforestation and degradation.[9] Land clearing reductions, the majority of the time, create biodiversity benefits in a vast expanse of land regions. Concerns, however, arise when the density and area of vegetation increases the grazing pressure could also increase in other areas, causing land degradation.[10] A recent report by the Australian CSIRO found that forestry and forest-related options are the most significant and most easily achieved carbon sink making up 105 Mt per year CO2-e or about 75 per cent of the total figure attainable for the Australian state of Queensland from 2010 to 2050. Among the forestry options, the CSIRO report announced, forestry with the primary aim of carbon storage (called carbon forestry) has the highest attainable carbon storage capacity (77 Mt CO2-e/yr) while strategy balanced with biodiversity plantings can return 7–12 times more native vegetation for a 10%–30% reduction of carbon storage performance.[11] Legal strategies to encourage this form of biosequestration include permanent protection of forests in National Parks or on the World Heritage List, properly funded management and bans on use of rainforest timbers and inefficient uses such as woodchipping old growth forest.[12]
As a result of lobbying by the developing country caucus (or Group of 77) in the United Nations (associated with the United Nations Conference on Environment and Development (UNCED) in Rio de Janeiro, the non-legally binding Forest Principles were established in 1992. These linked the problem of deforestation to third world debt and inadequate technology transfer and stated that the "agreed full incremental cost of achieving benefits associated with forest conservation...should be equitably shared by the international community" (para1(b)).[13] Subsequently, the Group of 77 argued in the 1995 Intergovernmental Panel on Forests (IPF) and then the 2001 Intergovernmental Forum on Forests (IFF), for affordable access to environmentally sound technologies without the stringency of intellectual property rights; while developed states there rejected demands for a forests fund.[14] The expert group created under the United Nations Forum on Forests (UNFF) reported in 2004, but in 2007 developed nations again vetoed language in the principles of the final text which might confirm their legal responsibility under international law to supply finance and environmentally sound technologies to the developing world.[15]
In December 2007, after a two-year debate on a proposal from Papua New Guinea and Costa Rica, state parties to the United Nations Framework Convention on Climate Change (FCCC) agreed to explore ways of reducing emissions from deforestation and to enhance forest carbon stocks in developing nations.[16] The underlying idea is that developing nations should be financially compensated if they succeed in reducing their levels of deforestation (through valuing the carbon that is stored in forests); a concept termed 'avoided deforestation (AD) or, REDD if broadened to include reducing forest degradation (see Reducing emissions from deforestation and forest degradation). Under the free market model advocated by the countries who have formed the Coalition of Rainforest Nations, developing nations with rainforests would sell carbon sink credits under a free market system to Kyoto Protocol Annex I states who have exceeded their emissions allowance.[17] Brazil (the state with the largest area of tropical rainforest) however, opposes including avoided deforestation in a carbon trading mechanism and instead favors creation of a multilateral development assistance fund created from donations by developed states.[17] For REDD to be successful science and regulatory infrastructure related to forests will need to increase so nations may inventory all their forest carbon, show that they can control land use at the local level and prove that their emissions are declining.[18]
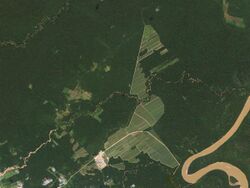
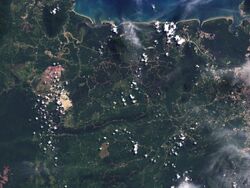
Subsequent to the initial donor nation response, the UN established REDD Plus, or REDD+, expanding the original program's scope to include increasing forest cover through both reforestation and the planting of new forest cover, as well as promoting sustainable forest resource management.[19]
The United Nations Framework Convention on Climate Change (UNFCCC) Article 4(1)(a) requires all Parties to "develop, periodically update, publish and make available to the Conference of the Parties" as well as "national inventories of anthropogenic emissions by sources" "removals by sinks of all greenhouse gases not controlled by the Montreal Protocol." Under the UNFCCC reporting guidelines, human-induced greenhouse emissions must be reported in six sectors: energy (including stationary energy and transport); industrial processes; solvent and other product use; agriculture; waste; and land use, land use change and forestry (LULUCF).[20] The rules governing accounting and reporting of greenhouse gas emissions from LULUCF under the Kyoto Protocol are contained in several decisions of the Conference of Parties under the UNFCCC and LULUCF has been the subject of two major reports by the Intergovernmental Panel on Climate Change (IPCC).[21] The Kyoto Protocol article 3.3 thus requires mandatory LULUCF accounting for afforestation (no forest for last 50 years), reforestation (no forest on 31 December 1989) and deforestation, as well as (in the first commitment period) under article 3.4 voluntary accounting for cropland management, grazing land management, revegetation and forest management (if not already accounted under article 3.3).[22]
thumb|right|Deforestation in Haiti. NASA, 2008.
As an example, the Australian National Greenhouse Gas Inventory (NGGI) prepared in compliance with these requirements indicates that the energy sector accounts for 69 per cent of Australia's emissions, agriculture 16 per cent and LULUCF six per cent. Since 1990, however, emissions from the energy sector have increased 35 per cent (stationary energy up 43% and transport up 23%). By comparison, emissions from LULUCF have fallen by 73%.[23] However, questions have been raised by Andrew Macintosh about the veracity of the estimates of emissions from the LULUCF sector because of discrepancies between the Australian Federal and Queensland Governments’ land clearing data. Data published by the Statewide Landcover and Trees Study (SLATS) in Queensland, for example, show that the total amount of land clearing in Queensland identified under SLATS between 1989/90 and 2000/01 is approximately 50 per cent higher than the amount estimated by the Australian Federal Government’s National Carbon Accounting System (NCAS) between 1990 and 2001.[24]
Satellite imaging has become crucial in obtaining data on levels of deforestation and reforestation. Landsat satellite data, for example, has been used to map tropical deforestation as part of NASA’s Landsat Pathfinder Humid Tropical Deforestation Project, a collaborative effort among scientists from the University of Maryland, the University of New Hampshire, and NASA’s Goddard Space Flight Center. The project yielded deforestation maps for the Amazon Basin, Central Africa, and Southeast Asia for three periods in the 1970s, 1980s, and 1990s.[25]
Enhanced photosynthesis
Biosequestration may be enhanced by improving photosynthetic efficiency by modifying RuBisCO genes in plants to increase the catalytic and/or oxygenation activity of that enzyme.[26] One such research area involves increasing the Earth's proportion of C4 carbon fixation photosynthetic plants. C4 plants represent about 5% of Earth's plant biomass and 1% of its known plant species,[27] but account for around 30% of terrestrial carbon fixation.[28] In leaves of C3 plants, captured photons of solar energy undergo photosynthesis which assimilates carbon into carbohydrates (triosephosphates) in the chloroplasts of the mesophyll cells. The primary CO2 fixation step is catalysed by ribulose-1,5-bisphosphate carboxylase/oxygenase (Rubisco) which reacts with O2 leading to photorespiration that protects photosynthesis from photoinhibition but wastes 50% of potentially fixed carbon.[29] The C4 photosynthetic pathway, however, concentrates CO2 at the site of the reaction of Rubisco, thereby reducing the biosequestration-inhibiting photorespiration.[30] A new frontier in crop science consists of attempts to genetically engineer C3 staple food crops (such as wheat, barley, soybeans, potatoes and rice) with the "turbo-charged" photosynthetic apparatus of C4 plants.[1]
Biochar
Biochar (charcoal created through the pyrolysis of biomass) is a potent and stable form of biosequestration derived from investigation of the highly fertile Terra preta soils of the Amazon Basin.[31][32] Placing biochar in soils also improves water quality, increases soil fertility, raises agricultural productivity, and reduces pressure on old growth forests.[33] As a method of generating Bio-energy with Carbon Capture and Storage Rob Flanagan and the EPRIDA biochar company have developed low-tech cooking stoves for developing nations that can burn agricultural wastes, producing 15% by weight of biochar. BEST Energies in NSW Australia have spent a decade developing an Agrichar technology that can combust 96 tonnes of dry biomass each day, generating 30-40 tonnes of biochar.[34] A parametric study of biosequestration by Malcolm Fowles at the Open University indicated that to mitigate global warming, policies should encourage displacement of coal with biomass for base load electricity generation if the latter's conversion efficiency rose over 30%. Biosequestering carbon from biomass is a cheaper mitigation option than geosequestration in carbon capture and storage.[35]
Agricultural and farming practices
No-till farming practices occur where, in the presence of mulching, ploughing is intentionally forgone as to maintain the sequestration of carbon-rich organic matter in soil. This practice prevents soil exposure to atmospheric oxygen, leaching, and erosion. Ceasing practice of ploughing has been alleged to encourage ant predation on wood-eating termites, allow weeds to regenerate soil, and help slow water flows over land.[36]
The soil stores more terrestrial carbon than the summation of both the atmospheric and vegetative carbon sinks. The greatest density of this sequestered carbon lies under grassland.[37][38] Studies reveal that Holistic Planned Grazing holds potential in mitigating global warming, while building soil, increasing biodiversity, and reversing desertification.[39][40] Developed by Allan Savory,[41] this practice uses fencing and/or herders to restore grasslands.[42][43][44] Carefully planned movements of large herds of livestock mimic the processes of nature where grazing animals are kept concentrated by pack predators and forced to move on after eating, trampling, and manuring an area, returning only after it has fully recovered. This grazing method seeks to emulate what occurred during the past 40 million years as the expansion of grass-grazer ecosystems built deep, rich grassland soils, sequestering carbon, and consequently cooling the planet.[45]
Dedicated biofuel and biosequestration crops, such as switchgrass (panicum virgatum), are also being developed.[46] This requires from 0.97 to 1.34 GJ fossil energy to produce 1 tonne of switchgrass, compared with 1.99 to 2.66 GJ to produce 1 tonne of corn.[47] Given that switchgrass contains approximately 18.8 GJ/ODT of biomass, the energy output-to-input ratio for the crop can be up to 20:1.[48]
Biosequestration can also be enhanced by species selection to produce large numbers of phytoliths. Phytoliths are microscopic spherical shells of silicon that can store carbon for thousands of years.[49]
Biosequestration and climate change policy
thumb|right|Windturbines D4 (nearest) to D1 on the Thornton BankIndustries with large amounts of CO2 emissions (such as the coal industry) are interested in biosequestration as a means of offsetting their greenhouse gas production.[50] In Australia, university researchers are engineering algae to produce biofuels (hydrogen and biodiesel oils) and investigating whether this process can be used to biosequester carbon. Algae naturally capture sunlight and use its energy to split water into hydrogen, oxygen and oil which can be extracted. Such clean energy production also can be coupled with desalination using salt-tolerant marine algae to generate fresh water and electricity.[51]
Many new bioenergy (biofuel) technologies, including cellulosic ethanol biorefineries (using stems and branches of most plants including crop residues such as corn stalks, wheat straw and rice straw) are being promoted because they have the added advantage of biosequestration of CO2.[52] The Garnaut Climate Change Review recommends that a carbon price in a carbon emission trading scheme could include a financial incentive for biosequestration processes.[53] Garnaut recommends the use of algal biosequestration (see algae bioreactor) to absorb the constant stream of carbon dioxide emissions from coal-fired electricity generation and metal smelting until renewable forms of energy, such as solar and wind power, become more established contributors to the grid.[54] Garnaut, for example, states: "Some algal biosequestration processes could absorb emissions from coal-fired electricity generation and metals smelting."[55] The United Nations Collaborative Programme on Reducing Emissions from Deforestation and Forest Degradation in Developing Countries (UN-REDD Programme) is a collaboration between FAO, UNDP and UNEP under which a trust fund established in July 2008 allows donors to pool resources to generate the requisite transfer flow of resources to significantly reduce global emissions from deforestation and forest degradation.[56] The UK government's Stern Review on the economics of climate change argued that curbing deforestation was a "highly cost-effective way of reducing greenhouse gas emissions".[57]
James E. Hansen argues that, "An effective way to achieve drawdown [of carbon dioxide] would be to burn biofuels in power plants and capture the CO2, with the biofuels derived from agricultural or urban wastes or grown on degraded lands using little or no fossil fuel inputs."[58] Such carbon dioxide drawdown systems are referred to as Bio-energy with carbon capture and storage, or BECCS. According to a study by Biorecro and the Global CCS Institute, there is currently (as of January 2012) 550 000 tonnes CO
2/year in total BECCS capacity operating, divided between three different facilities.[59]
Under a 2009 agreement, Loy Yang Power and MBD Energy Ltd will build a pilot Fossil fuel power plant at the Latrobe Valley power station in Australia using biosequestration technology in the form of an algal synthesiser system. Captured CO2 from the waste exhaust flue gases will be injected into circulating waste water to grow oil-rich algae where sunlight and nutrients will produce heavy oil-laden slurry that can make high grade oil for energy, or stock feed.[60] Other commercial demonstration projects involving biosequestration of CO2 at point of emission have begun in Australia.[61]
Philosophical basis
The arguments for biosequestration are often shaped in terms of economic theory, yet there is a well-recognised quality of life dimension to this debate.[62] Biosequestration assists human beings to increase their collective and individual contributions to the essential resources of the biosphere.[63] The policy case for biosequestration overlaps with principles of ecology, sustainability and sustainable development, as well as biosphere, biodiversity and ecosystem protection, environmental ethics, climate ethics, and natural conservation.
Barriers to increased global biosequestration
The Garnaut Climate Change Review notes many barriers to increased global biosequestration. "There must be changes in the accounting regimes for greenhouse gases. Investments are required in research, development and commercialisation of superior approaches to biosequestration. Adjustments are required in the regulation of land use. New institutions will need to be developed to coordinate the interests in utilisation of biosequestration opportunities across small business in rural communities. Special efforts will be required to unlock potential in rural communities in developing countries."[64] Saddler and King have argued that biosequestration and agricultural greenhouse gas emissions should not be handled within a global emissions trading scheme because of difficulties with measuring such emissions, problems in controlling them and the burden that would be placed on numerous small-scale farming operations.[65] Collett likewise maintains that REDD credits (post-facto payments to developing countries for reducing their deforestation rates below an historical or projected reference rate), simply create a complex market approach to this global public health problem that reduces transparency and accountability when targets are not met and will not be as effective as developed nations voluntarily funding countries to keep their rainforests.[66]
The World Rainforest Movement has argued that poor developing countries could be pressured to accept reforestation projects under the Kyoto Protocol's Clean Development Mechanism in order to earn foreign exchange simply to pay off the interest on debt to the World Bank.[67] Tensions also exist over forest management between the sovereignty claims of nations states, arguments about common heritage of mankind and the rights of indigenous peoples and local communities; the Forest Peoples Programme (FPP) arguing the anti-deforestation programs could merely allow financial benefits to flow to national treasuries, privilege would-be corporate forest degraders who manipulate the system by periodically threatening forests, rather than local communities who conserve them.[68] The success of such projects will also depend on the accuracy of the baseline data and the number of countries involved. Further, it has been argued that if biosequestration is to play a significant role in mitigating anthropogenic climate change then coordinated policies should set a goal of achieving global forest cover to its extent prior to the industrial revolution in the 1800s.[69]
It has also been argued that the United Nations mechanism for Reducing Emissions from Deforestation and Forest Degradation (REDD) may increase pressure to convert or modify other ecosystems, especially savannahs and wetlands, for food or biofuel, even though those ecosystems also have high carbon sequestration potential. Globally, for example, peatlands cover only 3% of the land surface but store twice the amount of carbon as all the world's forests, whilst mangrove forests and saltmarshes are examples of relatively low-biomass ecosystems with high levels of productivity and carbon sequestration.[70] Other researchers have argued that REDD is a critical component of an effective global biosequestration strategy that could provide significant benefits, such as the conservation of biodiversity, particularly if it moves away from focusing on protecting forests that are most cost-effective for reducing carbon emissions (such as those in Brazil where agricultural opportunity costs are relatively low, unlike Asia, which has sizeable revenues from oil palm, rubber, rice, and maize). They argue REDD could be varied to allow funding of programs to slow peat degradation in Indonesia and target protection of biodiversity in "hot spot"—areas with high species richness and relatively little remaining forest. Some purchasers, they maintain, of REDD carbon credits, such as multinational corporations or nations, might pay a premium to save imperiled eco-systems or areas with high-profile species.[71]
See also
- Azolla event
- Bio-energy with carbon capture and storage
- Blue carbon
- Carbon cycle
- Carbon dioxide removal
- Carbon negative
- Fossil-fuel power station
- SALK Harnessing Plants Initiative
- Greenhouse gas remediation
- Miscanthus giganteus
- Negative emissions
- Soil carbon
References
- ↑ 1.0 1.1 Beerling, David (2008). The Emerald Planet: How Plants Changed Earth's History. Oxford University Press. pp. 194–5. ISBN 978-0-19-954814-9.
- ↑ National Academies Of Sciences, Engineering (2019) (in en). Negative Emissions Technologies and Reliable Sequestration: A Research Agenda. Washington, D.C.: National Academies of Sciences, Engineering, and Medicine. pp. 45–136. doi:10.17226/25259. ISBN 978-0-309-48452-7.
- ↑ Jansson, Christer; Wullschleger, Stan D.; Kalluri, Udaya C.; Tuskan, Gerald A. (2010). "Phytosequestration: Carbon Biosequestration by Plants and the Prospects of Genetic Engineering". BioScience 60 (9): 685–696. doi:10.1525/bio.2010.60.9.6. ISSN 1525-3244.
- ↑ Garnaut 2008, p. 558 p. 609 defines biosequestration as involving greenhouse gases in general.
- ↑ Garnaut 2008, p. 33
- ↑ Earth CO2, 2022
- ↑ "Oceanic sinks for atmospheric CO2". Plant, Cell & Environment 22 (6): 741–55. 1999. doi:10.1046/j.1365-3040.1999.00419.x.
- ↑ Intergovernmental Panel on Climate Change * The IPCC web site
- ↑ "Managing Forests for Climate Change". Science 320 (5882): 1456–7. 2008. doi:10.1126/science.1155458. PMID 18556550. Bibcode: 2008Sci...320.1456C.
- ↑ "An analysis of greenhouse gas mitigation and carbon biosequestration opportunities from rural land use". 2009. https://www.fcrn.org.uk/sites/default/files/prdz.pdf.
- ↑ "Australian National Outlook 2015. Technical Report. Economic activity, resource use, environmental performance and living standards, 1970–2050". 2015-11-05. https://www.csiro.au/~/media/Major-initiatives/Australian-National-Outlook/CSIRO-TECHNICAL-REPORT-National_Outlook_2015.pdf?la=en&hash=DA85B33E39002CADDA5D05C94CA8E36E1210BEF5.
- ↑ Diesendorf, Mark (2009). Climate action: a campaign manual for greenhouse solutions. Sydney: University of New South Wales Press. p. 116. ISBN 978-1-74223-018-4.
- ↑ United Nations. Non-Legally Binding Authoritative Statement of Principles for a Global Consensus on the Management, Conservation and Sustainable Development of all Types of Forests. A/CONF.151/6/Rev1. United Nations, Rio de Janeiro. 1992.
- ↑ Humphreys, David (2006). Logjam: Deforestation and the Crisis of Global Governance. London: Earthscan. p. 280. ISBN 978-1-84407-301-6. https://archive.org/details/logjamdeforestat00hump.
- ↑ United Nations. Non-Legally Binding Instrument on All Types of Forests. United Nations 22 Oct. 2007. A/C.2/62/L.5.
- ↑ United Nations. 2007. Reducing emissions from deforestation in developing countries: approaches to stimulate action. accessed 10 November 2009.
- ↑ 17.0 17.1 Humphreys 2008, p. 434
- ↑ "On the road to REDD". Nature 462 (7269): 11. November 2009. doi:10.1038/462011a. PMID 19890280. Bibcode: 2009Natur.462Q..11..
- ↑ "UD Redd: Can the program save our tropical forests?". Thomaswhite.com. 11 May 2011. http://www.thomaswhite.com/global-perspectives/un-redd-can-the-program-save-our-tropical-forests/.
- ↑ Department of the Environment and Heritage (DEH) 2006, National Greenhouse Gas Inventory 2004: Accounting for the 108% Target, Commonwealth of Australia, Canberra.
- ↑ IPCC. Good Practice Guidance for Land Use, Land Use Change and Forestry. IPCC. Hayama, Japan 2003.
- ↑ "The rules for land use, land use change and forestry under the Kyoto Protocol—lessons learned for the future climate negotiations". Environmental Science and Policy 10 (4): 353–69. 2007. doi:10.1016/j.envsci.2007.02.001. at p. 354
- ↑ Department of the Environment and Heritage (DEH) 2006, National Greenhouse Gas Inventory: Analysis of Recent Trends and Greenhouse Indicators 1990 to 2004, Commonwealth of Australia, Canberra.
- ↑ Macintosh, Andrew (January 2007). The National Greenhouse Accounts and Land Clearing: Do the numbers stack up?. Australia Institute. pp. 19–20. Research Paper No. 38.
- ↑ Earth Observatory. NASA Tropical Deforestation Research. accessed 12 November 2009.
- ↑ "Rubisco: structure, regulatory interactions, and possibilities for a better enzyme". Annu Rev Plant Biol 53: 449–75. 2002. doi:10.1146/annurev.arplant.53.100301.135233. PMID 12221984.
- ↑ "The global distribution of ecosystems in a world without fire". New Phytologist 165 (2): 525–38. 2005. doi:10.1111/j.1469-8137.2004.01252.x. PMID 15720663.
- ↑ Osborne, C. P.; Beerling, D. J. (2006). "Nature's green revolution: the remarkable evolutionary rise of C4 plants". Philosophical Transactions of the Royal Society B: Biological Sciences 361 (1465): 173–94. doi:10.1098/rstb.2005.1737. PMID 16553316.
- ↑ Leegood RC. (2002). "C4 photosynthesis: principles of CO2 concentration and prospects for its introduction into C3 plants". J. Exp. Bot. 53 (369): 581–90. doi:10.1093/jexbot/53.369.581. PMID 11886878.
- ↑ Mitsue Miyao (2003). "Molecular evolution and genetic engineering of C4 photosynthetic enzymes". J. Exp. Bot. 54 (381): 179–89. doi:10.1093/jxb/54.381.179. PMID 12493846.
- ↑ Yousaf, Balal; Liu, Guijian; Wang, Ruwei; Abbas, Qumber; Imtiaz, Muhammad; Liu, Ruijia (2016). "Investigating the biochar effects on C-mineralization and sequestration of carbon in soil compared with conventional amendments using stable isotope (δ13C) approach". GCB Bioenergy 9 (6): 1085–1099. doi:10.1111/gcbb.12401.
- ↑ Laird, David A. (2008). "The Charcoal Vision: A Win–Win–Win Scenario for Simultaneously Producing Bioenergy, Permanently Sequestering Carbon, while Improving Soil and Water Quality". Agronomy Journal 100: 178–81. doi:10.2134/agrojnl2007.0161.
- ↑ "Ameliorating physical and chemical properties of highly weathered soils in the tropics with charcoal – a review". Biology and Fertility Soils 35 (4): 219–230. 2002. doi:10.1007/s00374-002-0466-4.
- ↑ Goodall 2008, pp. 210–31
- ↑ Fowles M (2007). "Black carbon sequestration as an alternative to bio-energy". Biomass and Bioenergy 31 (6): 426–32. doi:10.1016/j.biombioe.2007.01.012.
- ↑ Andrews, Peter (2008). Beyond the brink: Peter Andrews' radical vision for a sustainable Australian landscape. Sydney: ABC Books for the Australian Broadcasting Corporation. p. 40. ISBN 978-0-7333-2410-9.
- ↑ Fynn, A.J., P. Alvarez, J.R. Brown, M.R. George, C. Kustin, E.A. Laca, J.T. Oldfield, T. Schohr, C.L. Neely, and C.P. Wong. 2009. "Soil carbon sequestration in U.S. rangelands" Issues paper for protocol development. Environmental Defense Fund, New York, NY, USA.
- ↑ Follett, R.F., Kimble, J.M., Lal, R., 2001. "The Potential of U.S. Grazing Lands to Sequester Carbon and Mitigate the Greenhouse Effect" CRC Press LLC. 1-457.
- ↑ "Allan Savory: How to green the desert and reverse climate change." TED Talk, February 2013.
- ↑ Thackara, John (June 2010). "Greener Pastures". Seed Magazine. http://seedmagazine.com/content/article/Greener_Pastures.
- ↑ Savory, Allan; Jody Butterfield (1998-12-01) [1988]. Holistic Management: A New Framework for Decision Making (2nd ed. ed.). Washington, D.C.: Island Press. ISBN:1-55963-487-1.
- ↑ Teague, W.R.; Dowhower, S.L.; Baker, S.A.; Haile, N.; DeLaune, P.B.; Conover, D.M. (2011). "Grazing Management Impacts on Vegetation, Soil Biota and Soil Chemical, Physical and Hydrological Properties in Tall Grass Prairie". Agriculture, Ecosystems and Environment 141 (3–4): 310–322. doi:10.1016/j.agee.2011.03.009. http://www.sciencedirect.com/science/article/pii/S0167880911000934. Retrieved 2013-04-02.
- ↑ Weber, K.T.; Gokhale, B.S. (2011). "Effect of grazing on soil-water content in semiarid rangelands of southeast Idaho". Journal of Arid Environments 75 (5): 464–470. doi:10.1016/j.jaridenv.2010.12.009. Bibcode: 2011JArEn..75..464W. http://www.sciencedirect.com/science/article/pii/S0140196310003460. Retrieved 2013-04-02.
- ↑ Sanjari, G; Ghadiri, H; Ciesiolka, CAA; Yu, B (2008). "Comparing the effects of continuous and time-controlled grazing systems on soil characteristics in Southeast Queensland". Soil Research 46 (4): 348–358. doi:10.1071/sr07220. http://www.publish.csiro.au/paper/SR07220.htm. Retrieved 2013-04-02.
- ↑ Retallack, Gregory (2001). "Cenozoic Expansion of Grasslands and Climatic Cooling". The Journal of Geology 109 (4): 407–426. doi:10.1086/320791. Bibcode: 2001JG....109..407R. http://pages.uoregon.edu/dogsci/_media/directory/faculty/greg/grasslandscooling.pdf?id=directory%3Afaculty%3Agreg%3Aabout&cache=cache.
- ↑ Biotechnology Industry Organization (2007). Industrial Biotechnology Is Revolutionizing the Production of Ethanol Transportation Fuel pp. 3-4.
- ↑ "Cumulative Energy and Global Warming Impact from the Production of Biomass for Biobased Products". Journal of Industrial Ecology 7 (3–4): 147–62. 2004. doi:10.1162/108819803323059442.
- ↑ Samson, R. (2008). "Developing Energy Crops for Thermal Applications: Optimizing Fuel Quality, Energy Security and GHG Mitigation". in Pimentel, David. Biofuels, Solar and Wind as Renewable Energy Systems: Benefits and Risks. Berlin: Springer. pp. 395–423. ISBN 978-1-4020-8653-3. https://archive.org/details/biofuelssolarwin00pime_385.
- ↑ "Soil carbon sequestration in phytoliths". Soil Biology and Biochemistry 37: 117–24. 2005. doi:10.1016/j.soilbio.2004.06.013.
- ↑ Tom Fearon. Australia’s ‘massive advantage’ in bio-sequestration. Environmental Management News. Monday, 3 August 2009
- ↑ Guy Healey. Pond life fuels bio research The Australian. July 23, 2008
- ↑ International Energy Agency (2006). World Energy Outlook 2006 p. 8.
- ↑ Garnaut 2008, p. 558
- ↑ Garnaut 2008, p. 432
- ↑ Ross Garnaut. The Garnaut Climate Change Review. Cambridge University Press , Cambridge and Melbourne 2008 ISBN:978-0-521-74444-7. p432
- ↑ United Nations Collaborative Programme on Reducing Emissions from Deforestation and Forest Degradation in Developing Countries *Official UN-REDD Programme Website .
- ↑ Stern, Nicholas Herbert (2007). The economics of climate change: the Stern review. Cambridge, UK: Cambridge University Press. p. xxv. ISBN 978-0-521-70080-1. http://www.hm-treasury.gov.uk/independent_reviews/stern_review_economics_climate_change/stern_review_report.cfm. Retrieved 2009-12-28.
- ↑ James Hansen. Tell Barack Obama the Truth. accessed 1o Dec 2009.
- ↑ "Global Status of BECCS Projects 2010". Biorecro AB, Global CCS Institute. 2010. http://www.globalccsinstitute.com/publications/global-status-beccs-projects-2010/online/27026.
- ↑ MBD Energy Ltd. MBD captures Loy Yang Carbon Emissions. Eco Investor June 2009 "MBD Captures Loy Yang Carbon Emissions - Eco Investor June 2009 - MBD Energy". http://www.mbdenergy.com/catalogue/c17/c33/p129. accessed 28 Jan 2010.
- ↑ "Commercial scale demonstration of bio sequestration of carbon dioxide Wednesday, . Archived 2016-03-03 at the Wayback Machine accessed 28 Jan 2010". 25 November 2009. http://www.bairdmaritime.com/index.php?option=com_content&view=article&id=4389:commercial-scale-demonstration-of-bio-sequestration-of-carbon-dioxide&catid=116:environment&Itemid=211.
- ↑ Schumacher, E. F. (1974). Small Is Beautiful: A Study of Economics As If People Mattered. London: Abacus. p. 112. ISBN 978-0-349-13139-9.
- ↑ Davies, Geoffrey F. (2004). Economia: new economic systems to empower people and support the living world. Sydney: ABC Books for the Australian Broadcasting Corporation. pp. 202–3. ISBN 978-0-7333-1298-4.
- ↑ Garnaut 2008, p. 582
- ↑ Saddler H and King H. Agriculture and Emissions Trading: The Impossible Dream. Australia Institute Discussion Paper 102. Australia Institute, Canberra. 2008.
- ↑ Collett M (2009). "In the REDD: A conservative approach to reducing emissions from deforestation and forest degradation". CCLR 3: 324–39.
- ↑ Lohmann L. The Carbon shop: Planting New Problems. Briefing paper, Plantations Campaign, World Rainforest Movement, Moreton-in-March (UK) and Montevideo (Uruguay). 1999. p3.
- ↑ Humphreys 2008, p. 439
- ↑ Humphreys 2008, p. 440
- ↑ William, J. Sutherland WJ (2010). "A horizon scan of global conservation issues for 2010". Trends in Ecology & Evolution 25 (1): 1–7. doi:10.1016/j.tree.2009.10.003. PMID 19939492. https://www.repository.cam.ac.uk/handle/1810/252454. Retrieved 2020-09-12.
- ↑ Venter, Oscar; Laurance, William F.; Iwamura, Takuya; Wilson, Kerrie A.; Fuller, Richard A.; Possingham, Hugh P. (2009). "Harnessing Carbon Payments to Protect Biodiversity". Science 326 (5958): 1368. doi:10.1126/science.1180289. PMID 19965752. Bibcode: 2009Sci...326.1368V.
- Garnaut, Ross (2008). The Garnaut Climate Change Review. Cambridge, UK: Cambridge University Press. ISBN 978-0-521-74444-7. http://www.garnautreview.org.au/index.htm.
- Goodall, Chris (2008). Ten Technologies to Save the Planet. London: Green Profile. ISBN 978-1-84668-868-3.
- Humphreys, D (2008). "The politics of 'Avoided Deforestation': historical context and contemporary issues". International Forestry Review 10 (3): 433–42. doi:10.1505/ifor.10.3.433.
External links
- Greenfleet (not-for-profit company assisting with biosequestration options) http://www.greenfleet.com.au
- Negative Emissions Technologies and Reliable Sequestration. 2018. doi:10.17226/25259. ISBN 978-0-309-48452-7.
- Pew Center on Global Climate Change. Biosequestration fact sheet. http://www.c2es.org/technology/factsheet/Biosequestration
- Fungi pull carbon into northern forest soils; Organisms living on tree roots do lion’s share of sequestering carbon March 28, 2013 Vol.183 #9 Science News