Biology:Jamming avoidance response
The jamming avoidance response is a behavior of some species of weakly electric fish. It occurs when two electric fish with wave discharges meet – if their discharge frequencies are very similar, each fish shifts its discharge frequency to increase the difference between the two. By doing this, both fish prevent jamming of their sense of electroreception.
The behavior has been most intensively studied in the South American species Eigenmannia virescens. It is also present in other Gymnotiformes such as Apteronotus, as well as in the African species Gymnarchus niloticus. The jamming avoidance response was one of the first complex behavioral responses in a vertebrate to have its neural circuitry completely specified. As such, it holds special significance in the field of neuroethology.
Discovery
The jamming avoidance response (JAR) was discovered by Akira Watanabe and Kimihisa Takeda in 1963. The fish they used was an unspecified species of Eigenmannia, which has a quasi-sinusoidal wave discharge of about 300 Hz. They found that when a sinusoidal electrical stimulus is emitted from an electrode near the fish, if the stimulus frequency is within 5 Hz of the fish's electric organ discharge (EOD) frequency, the fish alters its EOD frequency to increase the difference between its own frequency and the stimulus frequency. Stimuli above the fish's EOD frequency push the EOD frequency downwards, while frequencies below that of the fish push the EOD frequency upwards, with a maximum change of about ±6.5 Hz.[1] This behavior was given the name "jamming avoidance response" several years later in 1972, in a paper by Theodore Bullock, Robert Hamstra Jr., and Henning Scheich.[2]
In 1975, Walter Heiligenberg discovered a JAR in the distantly-related Gymnarchus niloticus, the African knifefish, showing that the behavior had convergently evolved in two separate lineages.[3]
Behavior
Eigenmannia and other weakly electric fish use active electrolocation – they can locate objects by generating an electric field and detecting distortions in the field caused by interference from those objects. Electric fish use their electric organ to create electric fields, and they detect small distortions of these fields using special electroreceptive organs in the skin. All fish with the JAR are wave-discharging fish that emit steady quasi-sinusoidal discharges. For the genus Eigenmannia, frequencies range from 240 to 600 Hz.[4] The EOD frequency is very steady, typically with less than 0.3% variation over a 10-minute time span.[2]
If a neighboring sinusoidal electric field is discharging close to the fish's EOD frequency, it causes interference which results in sensory confusion in the fish and sufficient jamming to prevent it from electrolocating effectively.[5] Eigenmannia typically are within the electric field range of three to five other fish of the same species at any time. If many fish are located near each other, it is beneficial for each fish to distinguish between their own signal and those of others; this can be done by increasing the frequency difference between their discharges. Therefore, it seems to be the function of the JAR to avoid sensory confusion among neighboring fish.[6]
To determine how close the stimulus frequency is to the discharge frequency, the fish compares the two frequencies using its electroreceptive organs, rather than comparing the discharge frequency to an internal pacemaker; in other words, the JAR relies only on sensory information. This was determined experimentally by silencing a fish's electric organ with curare, and then stimulating the fish with two external frequencies. The JAR, measured from the electromotor neurons in the spinal cord, depended only on the frequencies of the external stimuli, and not on the frequency of the pacemaker.[7]
Neurobiology
Pathway in Eigenmannia (Gymnotiformes)
Most of the JAR pathway in the South American Gymnotiformes has been worked out using Eigenmannia virescens as a model system.[8][9]
Sensory coding
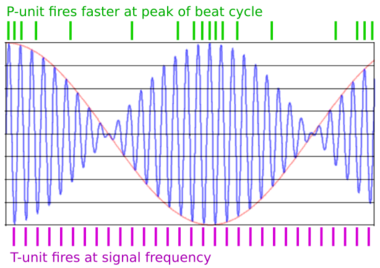
When the stimulus frequency and discharge frequency are close to each other, the two amplitude-time waves undergo interference, and the electroreceptive organs perceive a single wave with an intermediate frequency. In addition, the combined stimulus-EOD wave has a beat pattern, with the beat frequency equal to the frequency difference between the stimulus and EOD.[10]
Gymnotiforms have two classes of electroreceptive organs, the ampullary receptors and the tuberous receptors. Ampullary receptors respond to low-frequency stimulation less than 40 Hz and their role in the JAR is currently unknown. Tuberous receptors respond to higher frequencies, firing best near the fish's normal EOD frequency. Tuberous receptors themselves have two types, the T-unit and P-unit. The T-unit (T standing for time, meaning phase in the cycle) fires synchronously with the signal frequency by firing a spike on every cycle of the waveform. P-units (P standing for probability) tend to fire when the amplitude increases and fire less when it decreases. Under conditions of jamming, the P-unit fires on the amplitude peaks of the beat cycle where the two waves constructively interfere. So, a combined stimulus-EOD signal causes T-units to fire at the intermediate frequency, and causes P-unit firing to increase and decrease periodically with the beat.[7]
Processing in the brain
The time-coding T-units converge onto neurons called spherical cells in the electrosensory lateral line lobe. By combining information from multiple T-units, the spherical cell is even more precise in its time coding. Amplitude-coding P-units converge onto pyramidal cells, also in the electrosensory lateral line lobe. Two types of pyramidal cells exist: excitatory E-units, which fire more when stimulated by P-units, and inhibitory I-units, which fire less when stimulated by inhibitory interneurons activated by P-units.[8]
Spherical cells and pyramidal cells then project to the torus semicircularis, a structure with many laminae (layers) in the mesencephalon. Phase and amplitude information are integrated here to determine whether the stimulus frequency is greater or less than the EOD frequency. Sign-selective neurons in the deeper layers of the torus semicircularis are selective to whether the frequency difference is positive or negative; any given sign-selective cell fires in one case but not in the other.[9]
Output
Sign-selective cells input into the nucleus electrosensorius in the diencephalon, which then projects onto two different pathways. Neurons selective for a positive difference (stimulus greater than EOD) stimulate the prepacemaker nucleus, while neurons selective for a negative difference (stimulus less than EOD) inhibit the sublemniscal prepacemaker nucleus. Both prepacemaker nuclei send projections to the pacemaker nucleus, which ultimately controls the frequency of the EOD.[8]
Pathway in Gymnarchus (Osteoglossiformes)
The neural pathway of JAR in Gymnarchus is nearly identical to that of the Gymnotiformes, with a few minor differences. S-units in Gymnarchus are time coders, like the T-units in Gymnotiformes. O-units code the signal's intensity, like P-units in Gymnotiformes, but respond over a narrower range of intensities. In Gymnarchus, phase differences between EOD and stimulus are calculated in the electrosensory lateral line lobe rather than in the torus semicircularis.[11]
Phylogeny and evolution of weakly electric fish
There are two main orders of weakly electric fish, Gymnotiformes from South America and Osteoglossiformes from Africa. Electroreception most likely arose independently in the two lineages. Weakly electric fish are mostly pulse-dischargers, which do not perform the JAR, while some are wave-dischargers. Wave-discharge evolved in two taxa: the superfamily Apteronotoidea (order Gymnotiformes), and the species Gymnarchus niloticus (order Osteoglossiformes). Notable genera in Apteronotoidea that perform JAR include Eigenmannia and Apteronotus.[12] Though they evolved the JAR separately, the South American and African taxa (boldface in the tree) have convergently evolved nearly identical neural computational mechanisms and behavioral responses to avoid jamming, with only minor differences.[13] The phylogeny of the weakly electric fish clades, omitting non-electric and strongly-electric fishes, shows major events in their evolution.[12][14] In the tree, "sp" means "a species" and "spp" means "multiple species".
Vertebrates |
| ||||||||||||||||||||||||||||||||||||
See also
References
- ↑ Watanabe, Akira; Takeda, Kimihisa (1963). "The change of discharge frequency by A.C. stimulus in a weak electric fish". The Journal of Experimental Biology 40: 57–66. doi:10.1242/jeb.40.1.57. https://journals.biologists.com/jeb/article-pdf/40/1/57/1338830/57.pdf.
- ↑ 2.0 2.1 Bullock, Theodore H.; Hamstra, R. Jr.; Scheich, H. (1972). "The jamming avoidance response of high frequency electric fish". Journal of Comparative Physiology 77 (1): 1–22. doi:10.1007/BF00696517.
- ↑ Heiligenberg, Walter (1975). "Electrolocation and jamming avoidance in the electric fish Gymnarchus niloticus (Gymnarchidae, Mormyriformes)". Journal of Comparative Physiology A 103 (1): 55–67. doi:10.1007/bf01380044.
- ↑ Hopkins, C. (1974). "Electric communication: functions in the social behavior of Eigenmannia virescens". Behaviour 50 (3/4): 270–305. doi:10.1163/156853974X00499.
- ↑ Shifman, Aaron R.; Lewis, John E. (2018-01-04). "The complexity of high-frequency electric fields degrades electrosensory inputs: implications for the jamming avoidance response in weakly electric fish". Journal of the Royal Society Interface 15 (138): 20170633. doi:10.1098/rsif.2017.0633. PMID 29367237.
- ↑ Tan, E.; Nizar, J.; Carrera-G, E.; Fortune, E. (2005). "Electrosensory interference in naturally occurring aggregates of a species of weakly electric fish, Eigenmannia virescens". Behavioural Brain Research 164 (164): 83–92. doi:10.1016/j.bbr.2005.06.014. PMID 16099058.
- ↑ 7.0 7.1 Scheich, H.; Bullock, Theodore H.; Hamstra, R. Jr. (1973). "Coding properties of two classes of afferent nerve fibers: high-frequency electroreceptors in the electric fish, Eigenmannia". Journal of Neurophysiology 36 (1): 39–60. doi:10.1152/jn.1973.36.1.39. PMID 4705666.
- ↑ 8.0 8.1 8.2 Bastian, J.; Heiligenberg, Walter (1980). "Neural correlates of the jamming avoidance response of Eigenmannia". Journal of Comparative Physiology A 136 (2): 135–152. doi:10.1007/BF00656908.
- ↑ 9.0 9.1 Heiligenberg, Walter; Rose, G. (1985). "Phase and amplitude computations in the midbrain of an electric fish: Intracellular studies of neurons participating in the jamming avoidance response of Eigenmannia". The Journal of Neuroscience 5 (2): 515–531. doi:10.1523/JNEUROSCI.05-02-00515.1985. PMID 3973680.
- ↑ Kramer, Berndt (15 May 1999). "Waveform discrimination, phase sensitivity and jamming avoidance in a wave-type electric fish". Journal of Experimental Biology 202 (10): 1387–1398. doi:10.1242/jeb.202.10.1387. PMID 10210679.
- ↑ Bullock, T.; Behrend, K.; Heiligenberg, Walter (1975). "Comparison of the jamming avoidance responses in Gymnotid and Gymnarchid electric fish: A case of convergent evolution of behavior and its sensory basis". Journal of Comparative Physiology 103 (103): 97–121. doi:10.1007/BF01380047.
- ↑ 12.0 12.1 Bullock, T. H.; Bodznick, D. A.; Northcutt, R. G. (1983). "The phylogenetic distribution of electroreception: Evidence for convergent evolution of a primitive vertebrate sense modality". Brain Research Reviews 6 (1): 25–46. doi:10.1016/0165-0173(83)90003-6. PMID 6616267. https://deepblue.lib.umich.edu/bitstream/2027.42/25137/1/0000573.pdf.
- ↑ Kawasaki, M. (1975). "Independently evolved jamming avoidance responses in Gymnotid and Gymnarchid electric fish: a case of convergent evolution of behavior and its sensory basis". Journal of Comparative Physiology 103 (1): 97–121. doi:10.1007/BF00209614. PMID 8366474.
- ↑ Lavoué, Sébastien; Miya, Masaki; Arnegard, Matthew E.; Sullivan, John P.; Hopkins, Carl D.; Nishida, Mutsumi (2012-05-14). Murphy, William J.. ed. "Comparable Ages for the Independent Origins of Electrogenesis in African and South American Weakly Electric Fishes". PLOS ONE 7 (5): e36287. doi:10.1371/journal.pone.0036287. PMID 22606250. Bibcode: 2012PLoSO...736287L.
Further reading
- Heiligenberg, W. (1977) Principles of Electrolocation and Jamming Avoidance in Electric Fish: A Neuroethological Approach. Studies of Brain Function, Vol. 1. Berlin-New York: Springer Verlag.
- Heiligenberg, W. (1990) Electric Systems in Fish. Synapse 6:196-206.
- Heiligenberg, W. (1991) Neural Nets in Electric fish. MIT Press: Cambridge, Massachusetts.
- Kawasaki, M. (2009) Evolution of time-coding systems in weakly electric fishes. Zoological Science 26: 587-599.
![]() | Original source: https://en.wikipedia.org/wiki/Jamming avoidance response.
Read more |