Engineering:Cryogenic electron microscopy
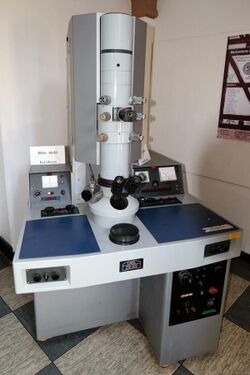
Cryogenic electron microscopy (cryo-EM) is an electron microscopy (EM) technique applied on samples cooled to cryogenic temperatures and embedded in an environment of vitreous water. An aqueous sample solution is applied to a grid-mesh and plunge-frozen in liquid ethane or a mixture of liquid ethane and propane.[2] While development of the technique began in the 1970s, recent advances in detector technology and software algorithms have allowed for the determination of biomolecular structures at near-atomic resolution.[3] This has attracted wide attention to the approach as an alternative to X-ray crystallography or NMR spectroscopy for macromolecular structure determination without the need for crystallization.
In 2017, the Nobel Prize in Chemistry was awarded to Jacques Dubochet, Joachim Frank, and Richard Henderson "for developing cryo-electron microscopy for the high-resolution structure determination of biomolecules in solution."[4] Nature Methods also named cryo-EM as the "Method of the Year" in 2016.[5]
Transmission electron cryomicroscopy
Cryogenic transmission electron microscopy (cryo-TEM) is a transmission electron microscopy technique that is used in structural biology and materials science.
- Cryogenic electron tomography (Cryo-ET), a specialized application where samples are imaged as they are tilted
- Electron crystallography, method to determine the arrangement of atoms in solids using a TEM
- MicroED,[6] method to determine the structure of proteins, peptides, organic molecules, and inorganic compounds using electron diffraction from 3D crystals[7][8][9]
- Single particle analysis cryo-EM, an averaging method to determine protein structure from monodisperse samples[10]
Cryo-TEM image of GroEL suspended in amorphous ice at 50000× magnification
Structure of alcohol oxidase from Pichia pastoris by Cryo-TEM
History
Early development
In the 1960s, the use of transmission electron microscopy for structure determination methods was limited because of the radiation damage due to high energy electron beams. Scientists hypothesized that examining specimens at low temperatures would reduce beam-induced radiation damage.[11] Both liquid helium (−269 °C or 4 K or −452.2 °F) and liquid nitrogen (−195.79 °C or 77 K or −320 °F) were considered as cryogens. In 1980, Erwin Knapek and Jacques Dubochet published commenting on beam damage at cryogenic temperatures sharing observations that:
Thin crystals mounted on carbon film were found to be from 30 to 300 times more beam-resistant at 4 K than at room temperature... Most of our results can be explained by assuming that cryoprotection in the region of 4 K is strongly dependent on the temperature.[12]
However, these results were not reproducible and amendments were published in Nature just two years later informing that the beam resistance was less significant than initially anticipated. The protection gained at 4 K was closer to "tenfold for standard samples of L-valine",[13] than what was previously stated.
In 1981, Alasdair McDowall and Jacques Dubochet, scientists at the European Molecular Biology Laboratory, reported the first successful implementation of cryo-EM.[14] McDowall and Dubochet vitrified pure water in a thin film by spraying it onto a hydrophilic carbon film that was rapidly plunged into cryogen (liquid propane or liquid ethane cooled to 77 K). The thin layer of amorphous ice was less than 1 µm thick and an electron diffraction pattern confirmed the presence of amorphous/vitreous ice. In 1984, Dubochet's group demonstrated the power of cryo-EM in structural biology with analysis of vitrified adenovirus type 2, T4 bacteriophage, Semliki Forest virus, Bacteriophage CbK, and Vesicular-Stomatitis-Virus.[15]
2017 Nobel Prize in Chemistry
In 2017, three scientists, Jacques Dubochet, Joachim Frank and Richard Henderson, were awarded the Nobel Prize in Chemistry for developing a technique that would image biomolecules.[4]
Potential rival to X-ray crystallography
As of October 27, 2020 X-ray crystallography has been used to image 150,494 biological samples and is the dominant technique in biological microscopy, with Cryo-EM far behind at just 6016.[16]
However, according to Nature, advancements in direct electron detectors (often referred to as a direct detection devices or DDDs) at the University of Cambridge[17] and automation of sample production by SPT labtech[18] has led to an increase in use in biological fields,[19] making Cryo-EM a potential rival.
The resolution of X-ray crystallography is limited by crystal purity,[20] and creating these samples is very time consuming, taking up to months or even years.[19] Also, some proteins are hard to crystalize.[19][21] Although sample preparation for Cryo-EM is still laborious,[22] it does not have these issues as it observes the sample in its “native state”.[21]
According to Proteopedia, the median resolution achieved by X-ray crystallography (as of May 19, 2019) on the Protein Data Bank is 2.05 Å,[20] and the highest resolution achieved on record (as of October 27, 2020) is 0.48 Å.[23] As of 2020, the majority of the protein structures determined by Cryo-EM are at a lower resolution of 3–4 Å.[24] However, the best Cryo-EM resolutions are approaching 1.5 Å,[25] making it a fair competitor in resolution in some cases.
Correlative light Cryo-TEM and Cryo-ET
In 2019, correlative light Cryo-TEM and Cryo-ET were used to observe tunnelling nanotubes (TNTs) in neuronal cells.[26]
Scanning electron cryomicroscopy
Scanning electron cryomicroscopy (cryoSEM) is a scanning electron microscopy technique with a scanning electron microscope's cold stage in a cryogenic chamber.
See also
References
- ↑ Xiao, C., Fischer, M.G., Bolotaulo, D.M., Ulloa-Rondeau, N., Avila, G.A., and Suttle, C.A. (2017) "Cryo-EM reconstruction of the Cafeteria roenbergensis virus capsid suggests novel assembly pathway for giant viruses". Scientific Reports, 7: 5484. doi:10.1038/s41598-017-05824-w.
- ↑ Tivol, William F.; Briegel, Ariane; Jensen, Grant J. (October 2008). "An Improved Cryogen for Plunge Freezing" (in en). Microscopy and Microanalysis 14 (5): 375–379. doi:10.1017/S1431927608080781. ISSN 1431-9276. PMID 18793481. Bibcode: 2008MiMic..14..375T.
- ↑ "A primer to single-particle cryo-electron microscopy". Cell 161 (3): 438–449. April 2015. doi:10.1016/j.cell.2015.03.050. PMID 25910204.
- ↑ 4.0 4.1 "Cryo-electron microscopy wins chemistry Nobel". Nature 550 (7675): 167. October 2017. doi:10.1038/nature.2017.22738. PMID 29022937. Bibcode: 2017Natur.550..167C.
- ↑ Doerr, Allison (January 2017). "Cryo-electron tomography". Nature Methods 14 (1): 34. doi:10.1038/nmeth.4115. ISSN 1548-7091.
- ↑ Nannenga, Brent L; Shi, Dan; Leslie, Andrew G W; Gonen, Tamir (2014-08-03). "High-resolution structure determination by continuous-rotation data collection in MicroED". Nature Methods 11 (9): 927–930. doi:10.1038/nmeth.3043. PMID 25086503.
- ↑ Jones, Christopher G.; Martynowycz, Michael W.; Hattne, Johan; Fulton, Tyler J.; Stoltz, Brian M.; Rodriguez, Jose A.; Nelson, Hosea M.; Gonen, Tamir (2018-11-02). "The CryoEM Method MicroED as a Powerful Tool for Small Molecule Structure Determination". ACS Central Science 4 (11): 1587–1592. doi:10.1021/acscentsci.8b00760. PMID 30555912.
- ↑ de la Cruz, M Jason; Hattne, Johan; Shi, Dan; Seidler, Paul; Rodriguez, Jose; Reyes, Francis E; Sawaya, Michael R; Cascio, Duilio et al. (2017). "Atomic-resolution structures from fragmented protein crystals with the cryoEM method MicroED". Nature Methods 14 (4): 399–402. doi:10.1038/nmeth.4178. PMID 28192420.
- ↑ "Rapid structure determination of microcrystalline molecular compounds using electron diffraction". Angewandte Chemie 57 (50): 16313–16317. October 2018. doi:10.1002/anie.201811318. PMID 30325568.
- ↑ Cheng, Yifan (2018-08-31). "Single-particle cryo-EM—How did it get here and where will it go" (in en). Science 361 (6405): 876–880. doi:10.1126/science.aat4346. ISSN 0036-8075. PMID 30166484. Bibcode: 2018Sci...361..876C.
- ↑ "Ups and downs in early electron cryo-microscopy". PLOS Biology 16 (4): e2005550. April 2018. doi:10.1371/journal.pbio.2005550. PMID 29672565.
- ↑ "Beam damage to organic material is considerably reduced in cryo-electron microscopy". Journal of Molecular Biology 141 (2): 147–61. August 1980. doi:10.1016/0022-2836(80)90382-4. PMID 7441748.
- ↑ Newmark, Peter (30 September 1982). "Cryo-transmission microscopy Fading hopes". Nature 299 (5882): 386–387. doi:10.1038/299386c0. Bibcode: 1982Natur.299..386N.
- ↑ Dubochet, J.; McDowall, A.W. (December 1981). "Vitrification of Pure Water for Electron Microscopy" (in en). Journal of Microscopy 124 (3): 3–4. doi:10.1111/j.1365-2818.1981.tb02483.x.
- ↑ Adrian, Marc; Dubochet, Jacques; Lepault, Jean; McDowall, Alasdair W. (March 1984). "Cryo-electron microscopy of viruses" (in en). Nature 308 (5954): 32–36. doi:10.1038/308032a0. ISSN 0028-0836. PMID 6322001. Bibcode: 1984Natur.308...32A. http://www.nature.com/articles/308032a0.
- ↑ "RCSB PDB - Holdings Report". https://www.rcsb.org/pdb/statistics/holdings.do.
- ↑ Callaway, Ewen (2015-09-10). "The revolution will not be crystallized: a new method sweeps through structural biology" (in en). Nature News 525 (7568): 172–174. doi:10.1038/525172a. PMID 26354465. Bibcode: 2015Natur.525..172C. http://www.nature.com/news/the-revolution-will-not-be-crystallized-a-new-method-sweeps-through-structural-biology-1.18335.
- ↑ Baker, Monya (2018-09-25). "Cryo-electron microscopy shapes up" (in en). Nature 561 (7724): 565–567. doi:10.1038/d41586-018-06791-6. PMID 30254359. Bibcode: 2018Natur.561..565B. https://www.nature.com/articles/d41586-018-06791-6.
- ↑ 19.0 19.1 19.2 Callaway, Ewen (2020-02-10). "Revolutionary cryo-EM is taking over structural biology" (in en). Nature 578 (7794): 201. doi:10.1038/d41586-020-00341-9. PMID 32047310. Bibcode: 2020Natur.578..201C. https://www.nature.com/articles/d41586-020-00341-9.
- ↑ 20.0 20.1 "Resolution - Proteopedia, life in 3D". https://proteopedia.org/wiki/index.php/Resolution#Confusion_of_high_vs._low_resolution.
- ↑ 21.0 21.1 "Cryo-EM Services - Creative Biostructure". https://www.creative-biostructure.com/cryo-em-services_4.htm.
- ↑ Bhella, David (2019-08-01). "Cryo-electron microscopy: an introduction to the technique, and considerations when working to establish a national facility" (in en). Biophysical Reviews 11 (4): 515–519. doi:10.1007/s12551-019-00571-w. ISSN 1867-2469. PMID 31359340.
- ↑ Bank, RCSB Protein Data. "RCSB PDB" (in en-US). https://www.rcsb.org/search?request=%7B%22query%22:%7B%22type%22:%22group%22,%22logical_operator%22:%22and%22,%22nodes%22:%5B%7B%22type%22:%22terminal%22,%22service%22:%22text%22,%22node_id%22:0%7D,%7B%22type%22:%22group%22,%22logical_operator%22:%22and%22,%22nodes%22:%5B%7B%22type%22:%22group%22,%22logical_operator%22:%22or%22,%22nodes%22:%5B%7B%22type%22:%22terminal%22,%22service%22:%22text%22,%22parameters%22:%7B%22attribute%22:%22rcsb_entry_info.resolution_combined%22,%22operator%22:%22less%22,%22value%22:0.5%7D,%22node_id%22:1%7D%5D%7D%5D,%22label%22:%22refinements%22%7D%5D%7D,%22return_type%22:%22entry%22,%22request_options%22:%7B%22scoring_strategy%22:%22combined%22,%22sort%22:%5B%7B%22sort_by%22:%22rcsb_accession_info.initial_release_date%22,%22direction%22:%22desc%22%7D%5D,%22pager%22:%7B%22start%22:0,%22rows%22:100%7D%7D,%22request_info%22:%7B%22src%22:%22ui%22,%22query_id%22:%22a4626603b23b34e7bdb697b74da938db%22%7D%7D.
- ↑ Yip, Ka Man; Fischer, Niels; Paknia, Elham; Chari, Ashwin; Stark, Holger (2020). "Atomic-resolution protein structure determination by cryo-EM". Nature 587 (7832): 157–161. doi:10.1038/s41586-020-2833-4. PMID 33087927. Bibcode: 2020Natur.587..157Y.
- ↑ Bhella, David (2019-08-01). "Cryo-electron microscopy: an introduction to the technique, and considerations when working to establish a national facility" (in en). Biophysical Reviews 11 (4): 515–519. doi:10.1007/s12551-019-00571-w. ISSN 1867-2469. PMID 31359340.
- ↑ Sartori-Rupp, Anna; Cordero Cervantes, Diégo; Pepe, Anna; Gousset, Karine; Delage, Elise; Corroyer-Dulmont, Simon; Schmitt, Christine; Krijnse-Locker, Jacomina et al. (December 2019). "Correlative cryo-electron microscopy reveals the structure of TNTs in neuronal cells" (in en). Nature Communications 10 (1): 342. doi:10.1038/s41467-018-08178-7. ISSN 2041-1723. PMID 30664666. Bibcode: 2019NatCo..10..342S.