Physics:Solid-state laser
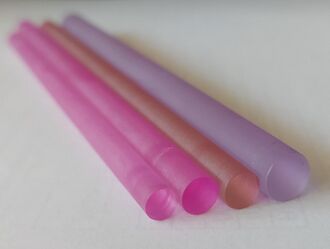
A solid-state laser is a laser that uses a gain medium that is a solid, rather than a liquid as in dye lasers or a gas as in gas lasers.[1] Semiconductor-based lasers are also in the solid state, but are generally considered as a separate class from solid-state lasers, called laser diodes.
Solid-state media
Generally, the active medium of a solid-state laser consists of a glass or crystalline "host" material, to which is added a "dopant" such as neodymium, chromium, erbium,[2] thulium[3] or ytterbium.[4] Many of the common dopants are rare-earth elements, because the excited states of such ions are not strongly coupled with the thermal vibrations of their crystal lattices (phonons), and their operational thresholds can be reached at relatively low intensities of laser pumping.
There are many hundreds of solid-state media in which laser action has been achieved, but relatively few types are in widespread use. Of these, probably the most common is neodymium-doped yttrium aluminum garnet (Nd:YAG). Neodymium-doped glass (Nd:glass) and ytterbium-doped glasses or ceramics are used at very high power levels (terawatts) and high energies (megajoules), for multiple-beam inertial confinement fusion.
The first material used for lasers was synthetic ruby crystals. Ruby lasers are still used for a few applications, but they are not common because of their low power efficiencies. At room temperature, ruby lasers emit only short pulses of light, but at cryogenic temperatures they can be made to emit a continuous train of pulses.[5]
Some solid-state lasers can also be tunable using several intracavity techniques, which employ etalons, prisms, and gratings, or a combination of these.[6] Titanium-doped sapphire is widely used for its broad tuning range, 660 to 1080 nanometers. Alexandrite lasers are tunable from 700 to 820 nm and yield higher-energy pulses than titanium-sapphire lasers because of the gain medium's longer energy storage time and higher damage threshold.
Pumping
Solid state lasing media are typically optically pumped, using either a flashlamp or arc lamp, or by laser diodes.[1] Diode-pumped solid-state lasers tend to be much more efficient and have become much more common as the cost of high-power semiconductor lasers has decreased.
Mode locking
Mode locking of solid-state lasers and fiber lasers has wide applications, as large-energy ultra-short pulses can be obtained.[1] There are two types of saturable absorbers that are widely used as mode lockers: SESAM,[7][8][9] and SWCNT. Graphene has also been used.[10][11][12] These materials use a nonlinear optical behavior called saturable absorption to make a laser create short pulses.
Current applications and developments
Solid-state lasers are being developed as optional weapons for the F-35 Lightning II, and are reaching near-operational status,[13][14][15] as well as the introduction of Northrop Grumman's FIRESTRIKE laser weapon system.[16][17] In April 2011 the United States Navy tested a high energy solid state laser. The exact range is classified, but they said it fired "miles not yards".[18][19]
Uranium-doped calcium fluoride was the second type of solid state laser invented, in the 1960s. Peter Sorokin and Mirek Stevenson at IBM's laboratories in Yorktown Heights (US) achieved lasing at 2.5 µm shortly after Maiman's ruby laser.
The U.S. Army is preparing to test a truck-mounted laser system using a 58 kW fiber laser.[20] The scalability of the laser opens up use on everything from drones to massive ships at different levels of power. The new laser puts 40 percent of available energy into its beam, which is considered very high for solid-state lasers. Since more and more military vehicles and trucks are using advanced hybrid engine and propulsion systems that produce electricity for applications like lasers the applications are likely to proliferate in trucks, drones, ships, helicopters and planes.[20]
See also
References
- ↑ Jump up to: 1.0 1.1 1.2 Heller, Jörg (1 March 2022). "A Primer on Solid-State Lasers" (in en). SAE Media Group. https://www.techbriefs.com/component/content/article/tb/supplements/pit/features/articles/45327.
- ↑ Singh, G.; Purnawirman; Bradley, J. D. B.; Li, N.; Magden, E. S.; Moresco, M.; Adam, T. N.; Leake, G. et al. (2016). "Resonant pumped erbium-doped waveguide lasers using distributed Bragg reflector cavities". Optics Letters 41 (6): 1189–1192. doi:10.1364/OL.41.001189. PMID 26977666. Bibcode: 2016OptL...41.1189S.
- ↑ Su, Z.; Li, N.; Magden, E. S.; Byrd, M.; Purnawirman; Adam, T. N.; Leake, G.; Coolbaugh, D. et al. (2016). "Ultra-compact and low-threshold thulium microcavity laser monolithically integrated on silicon". Optics Letters 41 (24): 5708–5711. doi:10.1364/OL.41.005708. PMID 27973495. Bibcode: 2016OptL...41.5708S.
- ↑ Z. Su, J. D. Bradley, N. Li, E. S. Magden, Purnawirman, D. Coleman, N. Fahrenkopf, C. Baiocco, T. Adam, G. Leake, D. Coolbaugh, D. Vermeulen, and M. R. Watts (2016) "Ultra-Compact CMOS-Compatible Ytterbium Microlaser", Integrated Photonics Research, Silicon and Nanophotonics 2016, IW1A.3.
- ↑ "Continuous solid-state laser operation revealed by BTL". Astronautics: 74. March 1962. http://www.gravityassist.com/IAF3-1/Ref.%203-49.pdf.
- ↑ N. P. Barnes, Transition metal solid-state lasers, in Tunable Lasers Handbook, F. J. Duarte (Ed.) (Academic, New York, 1995).
- ↑ H. Zhang et al., "Induced solitons formed by cross polarization coupling in a birefringent cavity fiber laser" , Opt. Lett., 33, 2317–2319.(2008).
- ↑ D. Y. Tang et al., "Observation of high-order polarization-locked vector solitons in a fiber laser" , Physical Review Letters, 101, 153904 (2008).
- ↑ L. M. Zhao et al., "Polarization rotation locking of vector solitons in a fiber ring laser" , Optics Express, 16,10053–10058 (2008).
- ↑ H. Zhang; D. Y. Tang; L. M. Zhao; Q. L. Bao; K. P. Loh (2009). "Large energy mode locking of an erbium-doped fiber laser with atomic layer graphene". Optics Express 17 (20): 17630–5. doi:10.1364/OE.17.017630. PMID 19907547. Bibcode: 2009OExpr..1717630Z. http://www3.ntu.edu.sg/home2006/zhan0174/OE_graphene.pdf.
- ↑ Han Zhang; Qiaoliang Bao; Dingyuan Tang; Luming Zhao; Kianping Loh (2009). "Large energy soliton erbium-doped fiber laser with a graphene-polymer composite mode locker". Applied Physics Letters 95 (14): P141103. doi:10.1063/1.3244206. Bibcode: 2009ApPhL..95n1103Z. http://www3.ntu.edu.sg/home2006/zhan0174/apl.pdf.
- ↑ "Graphene: Mode-locked lasers". NPG Asia Materials. 21 December 2009. doi:10.1038/asiamat.2009.52.
- ↑ Fulghum, David A. "Lasers being developed for F-35 and AC-130." Aviation Week and Space Technology, (8 July 2002). Access date: 8 February 2006.
- ↑ Morris, Jefferson. "Keeping cool a big challenge for JSF laser, Lockheed Martin says." Aerospace Daily, 26 September 2002. Access date: 3 June 2007.
- ↑ Fulghum, David A. "Lasers, HPM weapons near operational status." Aviation Week and Space Technology, 22 July 2002. Access date: 8 February 2006.
- ↑ "Northrop Grumman Press Release". http://www.irconnect.com/noc/press/pages/news_releases.html?d=154600.
- ↑ "The Register Press Release". https://www.theregister.co.uk/2008/11/14/weaponised_rayguns_hit_shelves_in_time_for_xmas/?d=154600.
- ↑ "US Navy's laser test could put heat on pirates". 13 April 2011. http://www.foxnews.com/world/2011/04/13/navys-laser-test-heat-pirates/.
- ↑ Kaplan, Jeremy A. (8 April 2011). "Navy shows off powerful new laser weapon". http://www.foxnews.com/scitech/2011/04/08/navy-showboats-destructive-new-laser-gun/.
- ↑ Jump up to: 20.0 20.1 Tucker, Patrick (16 March 2017). "US Army to Test Powerful New Truck-Mounted Laser 'Within Months'". http://www.defenseone.com/technology/2017/03/us-army-test-powerful-new-truck-mounted-laser-within-months/136239/?oref=search_navy.
- Koechner, Walter (1999). Solid-State Laser Engineering (5th ed.). Springer. ISBN 978-3-540-65064-5.
![]() | Original source: https://en.wikipedia.org/wiki/Solid-state laser.
Read more |