Earth:Younger Dryas
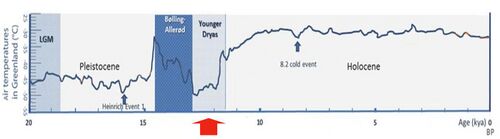
The Younger Dryas (around 12,900 to 11,700 years BP[2]) was a return to glacial conditions after the Late Glacial Interstadial, which temporarily reversed the gradual climatic warming after the Last Glacial Maximum (LGM) started receding around 20,000 BP. It is named after an indicator genus, the alpine-tundra wildflower Dryas octopetala, as its leaves are occasionally abundant in late glacial, often minerogenic-rich sediments, such as the lake sediments of Scandinavia.
Physical evidence of a sharp decline in temperature over most of the Northern Hemisphere has been discovered by geological research. This temperature change occurred at the end of what the earth sciences refer to as the Pleistocene epoch and immediately before the current, warmer Holocene epoch. In archaeology, this time frame coincides with the final stages of the Upper Paleolithic in many areas.
The Younger Dryas was the most recent and longest of several interruptions to the gradual warming of the Earth's climate since the severe LGM, about 27,000 to 24,000 years BP. The change was relatively sudden, taking place in decades, and it resulted in a decline of temperatures in Greenland by 4 to 10°C (7.2 to 18°F)[3] and advances of glaciers and drier conditions, over much of the temperate Northern Hemisphere. It is thought[4] to have been caused by a decline in the strength of the Atlantic meridional overturning circulation, which transports warm water from the Equator towards the North Pole, in turn thought to have been caused by an influx of fresh, cold water from North America to the Atlantic.
The Younger Dryas was a period of climatic change, but the effects were complex and variable. In the Southern Hemisphere and some areas of the Northern Hemisphere, such as southeastern North America, a slight warming occurred.[5]
General description and context
The presence of a distinct cold period at the end of the LGM interval has been known for a long time. Paleobotanical and lithostratigraphic studies of Swedish and Denmark bog and lake sites, as in the Allerød clay pit in Denmark, first recognized and described the Younger Dryas.[6][7][8][9]
The Younger Dryas is the youngest and longest of three stadials, which resulted from typically abrupt climatic changes that took place over the last 16,000 years.[10] Within the Blytt–Sernander classification of north European climatic phases, the prefix "Younger" refers to the recognition that this original "Dryas" period was preceded by a warmer stage, the Allerød oscillation, which, in turn, was preceded by the Older Dryas, around 14,000 calendar years BP. That is not securely dated, and estimates vary by 400 years, but it is generally accepted to have lasted around 200 years. In northern Scotland, the glaciers were thicker and more extensive than during the Younger Dryas.[11] The Older Dryas, in turn, was preceded by another warmer stage, the Bølling oscillation, that separated it from a third and even older stadial, often known as the Oldest Dryas. The Oldest Dryas occurred about 1,770 calendar years before the Younger Dryas and lasted about 400 calendar years. According to the GISP2 ice core from Greenland, the Oldest Dryas occurred between about 15,070 and 14,670 calendar years BP.[12]
In Ireland, the Younger Dryas has also been known as the Nahanagan Stadial, and in Great Britain, it has been called the Loch Lomond Stadial.[13][14] In the Greenland Summit ice core chronology, the Younger Dryas corresponds to Greenland Stadial 1 (GS-1). The preceding Allerød warm period (interstadial) is subdivided into three events: Greenland Interstadial-1c to 1a (GI-1c to GI-1a).[15]
Abrupt climate change
Since 1916 and the onset and then the refinement of pollen analytical techniques and a steadily-growing number of pollen diagrams, palynologists have concluded that the Younger Dryas was a distinct period of vegetational change in large parts of Europe during which vegetation of a warmer climate was replaced by that of a generally cold climate, a glacial plant succession that often contained Dryas octopetala. The drastic change in vegetation is typically interpreted to be an effect of a sudden decrease in (annual) temperature, unfavorable for the forest vegetation that had been spreading northward rapidly. The cooling not only favored the expansion of cold-tolerant, light-demanding plants and associated steppe fauna, but also led to regional glacial advances in Scandinavia and a lowering of the regional snow line.[6]
The change to glacial conditions at the onset of the Younger Dryas in the higher latitudes of the Northern Hemisphere, between 12,900 and 11,500 calendar years BP, has been argued to have been quite abrupt.[16] It is in sharp contrast to the warming of the preceding Older Dryas interstadial. Its end has been inferred to have occurred over a period of a decade or so,[17] but the onset may have even been faster.[18] Thermally fractionated nitrogen and argon isotope data from Greenland ice core GISP2 indicate that its summit was around 15 °C (27 °F) colder during the Younger Dryas[16][19] than today.
In Great Britain, beetle fossil evidence suggests that the mean annual temperature dropped to −5 °C (23 °F),[19] and periglacial conditions prevailed in lowland areas, and icefields and glaciers formed in upland areas.[20] Nothing of the period's size, extent, or rapidity of abrupt climate change has been experienced since its end.[16]
In addition to the Younger, Older, and Oldest Dryases, a century-long period of colder climate, similar to the Younger Dryas in abruptness, has occurred within both the Bølling oscillation and the Allerød oscillation interstadials. The cold period that occurred within the Bølling oscillation is known as the intra-Bølling cold period, and the cold period that occurred within the Allerød oscillation is known as the intra-Allerød cold period. Both cold periods are comparable in duration and intensity with the Older Dryas and began and ended quite abruptly. The cold periods have been recognized in sequence and relative magnitude in paleoclimatic records from Greenland ice cores, European lacustrine sediments, Atlantic Ocean sediments, and the Cariaco Basin, Venezuela.[21]
Examples of older Younger Dryas-like events have been reported from the ends (called terminations)[22] of older glacial periods. Temperature-sensitive lipids, long chain alkenones, found in lake and marine sediments, are well-regarded as a powerful paleothermometer for the quantitative reconstruction of past continental climates.[23] The application of alkenone paleothermometers to high-resolution paleotemperature reconstructions of older glacial terminations have found that very similar, Younger Dryas-like paleoclimatic oscillations occurred during Terminations II and IV. If so, the Younger Dryas is not the unique paleoclimatic event, in terms of size, extent, and rapidity, as it is often regarded to be.[23][24] Furthermore, paleoclimatologists and Quaternary geologists reported finding what they characterized as well-expressed Younger Dryas events in the Chinese δ18O records of Termination III in stalagmites from high-altitude caves in Shennongjia area, Hubei Province, China.[25] Various paleoclimatic records from ice cores, deep-sea sediments, speleothems, continental paleobotanical data, and loesses show similar abrupt climate events, which are consistent with Younger Dryas events, during the terminations of the last four glacial periods (see Dansgaard–Oeschger event). They argue that Younger Dryas events might be an intrinsic feature of deglaciations that occur at the end of glacial periods.[25][26][27]
Timing
Analyses of stable isotopes from Greenland ice cores provide estimates for the start and end of the Younger Dryas. The analysis of Greenland Summit ice cores, as part of the Greenland Ice Sheet Project-2 and Greenland Icecore Project, estimated that the Younger Dryas started about 12,800 ice (calendar) years BP. Depending on the specific ice core analysis consulted, the Younger Dryas is estimated to have lasted 1,150–1,300 years.[6][7] Measurements of oxygen isotopes from the GISP2 ice core suggest the ending of the Younger Dryas took place over just 40 to 50 years in three discrete steps, each lasting five years. Other proxy data, such as dust concentration and snow accumulation, suggest an even more rapid transition, which would require about 7 °C (13 °F) of warming in just a few years.[16][17][28][29] Total warming in Greenland was 10 ± 4 °C (18 ± 7 °F).[30]
The end of the Younger Dryas has been dated to around 11,550 years ago, occurring at 10,000 BP (uncalibrated radiocarbon year), a "radiocarbon plateau" by a variety of methods, mostly with consistent results:
Years ago | Place |
---|---|
11500 ± 50 | GRIP ice core, Greenland[31] |
11530 + 40− 60 | Krakenes Lake, western Norway [32] |
11570 | Cariaco Basin core, Venezuela[33] |
11570 | German oak and pine dendrochronology[34] |
11640 ± 280 | GISP2 ice core, Greenland[28] |
The International Commission on Stratigraphy put the start of the Greenlandian stage, and implicitly the end of the Younger Dryas, at 11,700 years before 2000.[35]
Although the start of the Younger Dryas is regarded to be synchronous across the North Atlantic region, recent research concluded that the start of the Younger Dryas might be time-transgressive even within there. After an examination of laminated varve sequences, Muschitiello and Wohlfarth found that the environmental changes that define the beginning of the Younger Dryas are diachronous in their time of occurrence according to latitude. According to the changes, the Younger Dryas occurred as early as AROUND 12,900–13,100 calendar years ago along latitude 56–54°N. Further north, they found that the changes occurred at roughly 12,600–12,750 calendar years ago.[36]
According to the analyses of varved sediments from Lake Suigetsu, Japan, and other paleoenvironmental records from Asia, a substantial delay occurred in the onset and the end of the Younger Dryas between Asia and the North Atlantic. For example, paleoenvironmental analysis of sediment cores from Lake Suigetsu in Japan found the Younger Dryas temperature decline of 2–4°C between 12,300 and 11,250 varve (calendar) years BP, instead of about 12,900 calendar years BP in the North Atlantic region.
In contrast, the abrupt shift in the radiocarbon signal from apparent radiocarbon dates of 11,000 radiocarbon years to radiocarbon dates of 10,700–10,600 radiocarbon years BP in terrestrial macrofossils and tree rings in Europe over a 50-year period occurred at the same time in the varved sediments of Lake Suigetsu. However, this same shift in the radiocarbon signal antedates the start of Younger Dryas at Lake Suigetsu by a few hundred years. Interpretations of data from Chinese also confirm that the Younger Dryas East Asia lags the North Atlantic Younger Dryas cooling by at least 200 to 300 years. Although the interpretation of the data is more murky and ambiguous, the end of the Younger Dryas and the start of Holocene warming likely were similarly delayed in Japan and in other parts of East Asia.[37]
Similarly, an analysis of a stalagmite growing from a cave in Puerto Princesa Subterranean River National Park, Palawan, the Philippines , found that the onset of the Younger Dryas was also delayed there. Proxy data recorded in the stalagmite indicate that more than 550 calendar years were needed for Younger Dryas drought conditions to reach their full extent in the region and about 450 calendar years to return to pre-Younger Dryas levels after it ended.[38]
Global effects
In Western Europe and Greenland, the Younger Dryas is a well-defined synchronous cool period.[39] Cooling in the tropical North Atlantic may, however, have preceded it by a few hundred years; South America shows a less well-defined initiation but a sharp termination. The Antarctic Cold Reversal appears to have started a thousand years before the Younger Dryas and has no clearly defined start or end; Peter Huybers has argued that there is a fair confidence in the absence of the Younger Dryas in Antarctica, New Zealand and parts of Oceania.[40] Timing of the tropical counterpart to the Younger Dryas, the Deglaciation Climate Reversal (DCR), is difficult to establish as low latitude ice core records generally lack independent dating over the interval. An example of this is the Sajama ice core (Bolivia), for which the timing of the DCR has been pinned to that of the GISP2 ice core record (central Greenland). Climatic change in the central Andes during the DCR, however, was significant and was characterized by a shift to much wetter and likely colder conditions.[41] The magnitude and abruptness of the changes would suggest that low latitude climate did not respond passively during the YD/DCR.
Effects of the Younger Dryas were of varying intensity throughout North America.[42] In western North America, its effects were less intense than in Europe or northeast North America;[43] however, evidence of a glacial re-advance[44] indicates that Younger Dryas cooling occurred in the Pacific Northwest. Speleothems from the Oregon Caves National Monument and Preserve in southern Oregon's Klamath Mountains yield evidence of climatic cooling contemporaneous to the Younger Dryas.[45]
Other features include the following:
- Replacement of forest in Scandinavia with glacial tundra (which is the habitat of the plant Dryas octopetala)
- Glaciation or increased snow in mountain ranges around the world
- Formation of solifluction layers and loess deposits in Northern Europe
- More dust in the atmosphere, originating from deserts in Asia
- A decline in evidence for Natufian hunter gatherer permanent settlements in the Levant, suggesting a reversion to a more mobile way of life[46]
- The Huelmo/Mascardi Cold Reversal in the Southern Hemisphere ended at the same time
- Decline of the Clovis culture; the extinction of megafauna game species in North America has been linked to the Younger Dryas but recently, it has been found that megafauna populations collapsed 1000 years earlier[47]
North America
East
The Younger Dryas is a period significant to the study of the response of biota to abrupt climate change and to the study of how humans coped with such rapid changes.[48] The effects of sudden cooling in the North Atlantic had strongly regional effects in North America, with some areas experiencing more abrupt changes than others.[49]
The effects of the Younger Dryas cooling impacted New England and parts of maritime Canada more rapidly than the rest of the United States at the beginning and the end of the Younger Dryas chronozone.[50][51][52][53] Proxy indicators show that summer temperature conditions in Maine decreased by up to 7.5oC. Cool summers, combined with cold winters and low precipitation, resulted in a treeless tundra up to the onset of the Holocene, when the boreal forests shifted north.[54]
Vegetation in the central Appalachian Mountains east towards the Atlantic Ocean was dominated by spruce (Picea spp.) and tamarack (Larix laricina) boreal forests that later changed rapidly to temperate, more broad-leaf tree forest conditions at the end of the Younger Dryas period.[55][56] Conversely, pollen and macrofossil evidence from near Lake Ontario indicates that cool, boreal forests persisted into the early Holocene.[56] West of the Appalachians, in the Ohio River Valley and south to Florida rapid, no-analog vegetation responses seem to have been the result of rapid climate changes, but the area remained generally cool, with hardwood forest dominating.[55] During the Younger Dryas, the Southeastern United States was warmer and wetter than the region had been during the Pleistocene[56][49][57] because of trapped heat from the Caribbean within the North Atlantic Gyre caused by a weakened Atlantic meridional overturning circulation (AMOC).[58]
Central
Also, a gradient of changing effects occurred from the Great Lakes region south to Texas and Louisiana. Climatic forcing moved cold air into the northern portion of the American interior, much as it did the Northeast.[59][60] Although there was not as abrupt a delineation as seen on the Eastern Seaboard, the Midwest was significantly colder in the northern interior than it was south, towards the warmer climatic influence of the Gulf of Mexico.[49][61] In the north, the Laurentide Ice Sheet re-advanced during the Younger Dryas, depositing a moraine from west Lake Superior to southeast Quebec.[62] Along the southern margins of the Great Lakes, spruce dropped rapidly, while pine increased, and herbaceous prairie vegetation decreased in abundance, but increased west of the region.[63][60]
Rocky Mountains
Effects in the Rocky Mountain region were varied.[64][65] In the northern Rockies, a significant increase in pines and firs suggests warmer conditions than before and a shift to subalpine parkland in places.[66][67][68][69] That is hypothesized to be the result of a northward shift in the jet stream, combined with an increase in summer insolation[66][70] as well as a winter snow pack that was higher than today, with prolonged and wetter spring seasons.[71] There were minor re-advancements of glaciers in place, particularly in the northern ranges,[72][73] but several sites in the Rocky Mountain ranges show little to no changes in vegetation during the Younger Dryas.[67] Evidence also indicates an increase in precipitation in New Mexico because of the same Gulf conditions that were influencing Texas.[74]
West
The Pacific Northwest region experienced 2 to 3°C of cooling and an increase in precipitation.[75][57][76][77][78] Glacial re-advancement has been recorded in British Columbia[79][80] as well as in the Cascade Range.[81] An increase of pine pollen indicates cooler winters within the central Cascades.[82] Speleothem records indicate an increase in precipitation in southern Oregon,[78][83] the timing of which coincides with increased sizes of pluvial lakes in the northern Great Basin.[84] A pollen record from the Siskiyou Mountains suggests a lag in timing of the Younger Dryas, indicating a greater influence of warmer Pacific conditions on that range,[85] but the pollen record is less chronologically constrained than the aforementioned speleothem record. The Southwest appears to have seen an increase in precipitation, as well, also with an average 2° of cooling.[86]
Effects on agriculture
The Younger Dryas is often linked to the Neolithic Revolution, the adoption of agriculture in the Levant.[87][88] The cold and dry Younger Dryas arguably lowered the carrying capacity of the area and forced the sedentary early Natufian population into a more mobile subsistence pattern. Further climatic deterioration is thought to have brought about cereal cultivation. While relative consensus exists regarding the role of the Younger Dryas in the changing subsistence patterns during the Natufian, its connection to the beginning of agriculture at the end of the period is still being debated.[89][90]
Sea level
Based upon solid geological evidence, consisting largely of the analysis of numerous deep cores from coral reefs, variations in the rates of sea level rise have been reconstructed for the postglacial period. For the early part of the sea level rise that is associated with deglaciation, three major periods of accelerated sea level rise, called meltwater pulses, occurred. They are commonly called meltwater pulse 1A0 for the pulse between 19,000 and 19,500 calendar years ago; meltwater pulse 1A for the pulse between 14,600 and 14,300 calendar years ago and meltwater pulse 1B for the pulse between 11,400 and 11,100 calendar years ago. The Younger Dryas occurred after meltwater pulse 1A, a 13.5 m rise over about 290 years, centered at about 14,200 calendar years ago, and before meltwater pulse 1B, a 7.5 m rise over about 160 years, centered at about 11,000 calendar years ago.[91][92][93] Finally, not only did the Younger Dryas postdate both all of meltwater pulse 1A and predate all of meltwater pulse 1B, it was a period of significantly-reduced rate of sea level rise relative to the periods of time immediately before and after it.[91][94]
Possible evidence of short-term sea level changes has been reported for the beginning of the Younger Dryas. First, the plotting of data by Bard and others suggests a small drop, less than 6 m, in sea level near the onset of the Younger Dryas. There is a possible corresponding change in the rate of change of sea level rise seen in the data from both Barbados and Tahiti. Given that this change is "within the overall uncertainty of the approach," it was concluded that a relatively smooth sea-level rise, with no significant accelerations, occurred then.[94] Finally, research by Lohe and others in western Norway has reported a sea-level low-stand at 13,640 calendar years ago and a subsequent Younger Dryas transgression starting at 13,080 calendar years ago. They concluded that the timing of the Allerød low-stand and the subsequent transgression were the result of increased regional loading of the crust, and geoid changes were caused by an expanding ice sheet, which started growing and advancing in the early Allerød about 13,600 calendar years ago, well before the start of the Younger Dryas.[95]
Causes
The current theory is that the Younger Dryas was caused by significant reduction or shutdown of the North Atlantic "Conveyor", which circulates warm tropical waters northward, in response to a sudden influx of fresh water from Lake Agassiz and deglaciation in North America. Geological evidence for such an event is not fully secure,[96] but recent work has identified a pathway along the Mackenzie River that would have spilled fresh water into the Arctic and thence into the Atlantic.[97][98] The global climate would then have become locked into the new state until freezing removed the fresh water "lid" from the North Atlantic. However, simulations indicated that a one-time-flood could not likely cause the new state to be locked for 1000 years. Once the flood ceased, the AMOC would recover and the Younger Dryas would stop in less than 100 years. Therefore, continuous freshwater input was necessary to maintain a weak AMOC for more than 1000 years. Recent study proposed that the snowfall could be a source of continuous freshwater resulting in a prolonged weakened state of the AMOC.[99] An alternative theory suggests instead that the jet stream shifted northward in response to the changing topographic forcing of the melting North American ice sheet, which brought more rain to the North Atlantic, which freshened the ocean surface enough to slow the thermohaline circulation.[100]There is also some evidence that a solar flare may have been responsible for the megafaunal extinction, but that cannot explain the apparent variability in the extinction across all continents.[101]
Impact hypothesis
A hypothesized Younger Dryas impact event, presumed to have occurred in North America about 12,900 years ago, has been proposed as the mechanism that initiated the Younger Dryas cooling.[102]
Among other things, findings of melt-glass material in sediments in Pennsylvania, South Carolina and Syria have been reported. The researchers argue that the material, which dates back nearly 13,000 years, was formed at temperatures of 1,700 to 2,200 °C (3,100 to 4,000 °F) as the result of a bolide impact. They argue that these findings support the controversial Younger Dryas Boundary (YDB) hypothesis that the bolide impact occurred at the onset of the Younger Dryas.[103] The hypothesis has been questioned in research that concluded that most of the results cannot be confirmed by other scientists and that the authors misinterpreted the data.[104][105][106]
After a review of the sediments found at the sites, new research has found that the sediments claimed by hypothesis proponents to be deposits resulting from a bolide impact date from much later or much earlier times than the proposed date of the cosmic impact. The researchers examined 29 sites commonly referenced to support the impact theory to determine if they can be geologically dated to around 13,000 years ago. Crucially, only three of those sites actually date from then.[107]
Charles R. Kinzie, et al. looked at the distribution of nanodiamonds produced during extraterrestrial collisions: 50 million km2 of the Northern Hemisphere at the YDB were found to have the nanodiamonds.[108] Only two layers exist showing these nanodiamonds: the YDB 12,800 calendar years ago and the Cretaceous-Tertiary boundary, 65 million years ago, which, in addition, is marked by mass extinctions.[109]
New support for the cosmic-impact hypothesis of the origin of the YDB was published in 2018. It postulates Earth's collision with one or more fragments from a larger (over 100-km diameter) disintegrating comet (some remnants of which have persisted within the inner solar system to the present day). Evidence is presented consistent with large-scale biomass burning (wildfires) following the putative collision. The evidence is derived from analyses of ice cores, glaciers, lake- and marine-sediment cores, and terrestrial sequences.[110][111]
Evidence that adds further to the credibility of this hypothesis includes extraterrestrial platinum, which has been found in meteorites. There are multiple sites around the world with spikes in levels of platinum that can be associated with the Impact Hypothesis, of which at least 25 are major.[112] Although most of these sites are found in the Northern Hemisphere, a study conducted in October 2019 has found and confirmed another site with high platinum levels located in the Wonderkrater area north of Pretoria in South Africa .[113] This coincides with the Pilauco site in southern Chile which also happens to contain high levels of platinum as well as rare metallic spherules, gold and high-temperature iron that is rarely found in nature and suspected of originating from airbursts or impacts.[114][115][116] These Southern Hemisphere high platinum zones further add to the credibility of the Younger Dryas impact hypothesis.
Laacher See eruption hypothesis
The Laacher See volcano erupted at approximately the same time as the beginning of the Younger Dryas, and has historically been suggested as a possible cause. Laacher See is a maar lake, a lake within a broad low-relief volcanic crater about 2 km (1.2 mi) diameter. It is in Rhineland-Palatinate, Germany , about 24 km (15 mi) northwest of Koblenz and 37 km (23 mi) south of Bonn. The maar lake is within the Eifel mountain range, and is part of the East Eifel volcanic field within the larger Vulkaneifel.[117][118] This eruption was of sufficient size, VEI 6, with over 20 km3 (2.4 cu mi) tephra ejected,[119] to have caused significant temperature change in the Northern Hemisphere.
Currently available evidence suggests that the hypothesis that the Laacher See eruption triggered the Younger Dryas has considerable merit. Earlier, the hypothesis was dismissed based on the timing of the Laacher See Tephra relative to the clearest signs of climate change associated with the Younger Dryas Event within various Central European varved lake deposits.[119][120] This set the scene for the development of the Younger Dryas Impact Hypothesis and the meltwater pulse hypothesis. However, more recent research places the very large eruption of the Laacher See volcano at 12,880 years BP, coinciding with the initiation of North Atlantic cooling into the Younger Dryas.[121][122] Although the eruption was about twice size as the 1991 eruption of Mount Pinatubo, it contained considerably more sulfur, potentially rivalling the climatologically very significant 1815 eruption of Mount Tambora in terms of amount of sulfur introduced into the atmosphere.[122] Evidence exists that an eruption of this magnitude and sulfur content occurring during deglaciation could trigger a long-term positive feedback involving sea ice and oceanic circulation, resulting in a cascade of climate shifts across the North Atlantic and the globe.[122] Further support for this hypothesis appears as a large volcanogenic sulfur spike within Greenland ice, coincident with both the date of the Laacher See eruption and the beginning of cooling into the Younger Dryas as recorded in Greenland.[122] The mid-latitude westerly winds may have tracked sea ice growth southward across the North Atlantic as the cooling became more pronounced, resulting in time transgressive climate shifts across northern Europe and explaining the lag between the Laacher See Tephra and the clearest (wind-derived) evidence for the Younger Dryas in central European lake sediments.[123][124]
Although the timing of the eruption appears to coincide with the beginning of the Younger Dryas, and the amount of sulfur contained would have been enough to result in substantial Northern Hemisphere cooling, the hypothesis has not yet been tested thoroughly, and no climate model simulations are currently available. The exact nature of the positive feedback is also unknown, and questions remain regarding the sensitivity to the deglacial climate to a volcanic forcing of the size and sulfur content of the Laacher See eruption. However, evidence exists that a similar feedback following other volcanic eruptions could also have triggered similar long-term cooling events during the last glacial period,[125] the Little Ice Age,[126][127] and the Holocene in general,[128] suggesting that the proposed feedback is poorly constrained but potentially common.
It is possible that the Laacher See eruption was triggered by lithospheric unloading related to the removal of ice during the last deglaciation,[129][130] a concept that is supported by the observation that three of the largest eruptions within the East Eifel Volcanic Field occurred during deglaciation.[131] Because of this potential relationship to lithospheric unloading, the Laacher See eruption hypothesis suggests that eruptions such as the 12,880 year BP Laacher See eruption are not isolated in time and space, but instead are a fundamental part of deglaciation, thereby also explaining the presence of Younger Dryas-type events during other glacial terminations.[122][132]
Vela supernova hypothesis
Studies suggest correlations between the Younger Dryas and a supernova that exploded within the constellation of Vela approximately around the same time, leaving behind what is now known as the Vela supernova remnant. Researchers who studied effects of nearby supernovae on earth uncovered suggestive correlating evidence during the Younger Dryas, including depletion of the ozone layer, increased UV exposure, nitrogen changes on the Earth's surface and in the troposphere, evidence of global cooling, changes in 14C and 10Be in ice cores, a thin layer (about 30 cm) of “black mats”, and many extinctions that may have been caused by the explosion of the Vela supernova.[133]
While no cause is determined for the extinction of many species, a combination of some climatic change or being hunted by humans is suggested as a cause. Most of the species that became extinct were cold-blooded species and Rancholabrean megafauna, including Columbian mammoth, Dire wolf, and Camelops. Survivors of the Younger Dryas included nocturnal, low-birthrate species residing in the mountainous or forest-like terrains of the Americas, Eurasia, Australia, and Madagascar. The largest, slow-breeding, diurnal species that lived in more open spaces survived in the lower parts of Africa.[133]
Across faunal and paleoindian hunting sites, evidence existed of carbon-rich “black mats” that were around 30 cm in thickness, suggesting an abrupt change that occurred in a small time window and a rise in aquatic conditions. Brakenridge also discusses pollen-core research that suggests global cooling conditions not to have just occurred in the northern latitudes, but also latitudes as far as 41°S. Tree-ring evidence shows a rise in 14C cosmogenic isotope. The increase may have also occurred around the same time as the increase of another cosmogenic isotope, 10Be.[133]
Another hypothesis discussed is that effects of a supernova could have been a factor in the Younger Dryas. Effects of a supernova have been suggested before, but without confirming evidence. Potential evidence that these effects could have been caused by a celestial event, a supernova, are observations of gamma-ray bursts and X-ray flashes have been compared to nebular records to test this, as well as supernovae flash models, comparable to the records of in-galaxy supernovae, to study the effects of such an event on Earth. These effects include depletion of the ozone layer, increased UV exposure, global cooling, and nitrogen changes in the Earth's surface and troposphere. As Brakenridge[133] states, the only supernova possible at that time was the Vela supernova, now classified as the Vela supernova remnant. Most geologists regard this hypothesis as an academic exercise by astronomers with little knowledge of earth system science.[134]
See also
- 8.2 kiloyear climate event – Sudden decrease in global temperatures c. 6200 BCE
- Earth:Heinrich event – Large groups of icebergs traverse the North Atlantic.
- Huelmo–Mascardi Cold Reversal
- Earth:Little Ice Age – A period of cooling after the Medieval Warm Period that lasted from the 16th to the 19th century
- Earth:Medieval Warm Period – Time of warm climate in the North Atlantic region lasting from c. 950 to c. 1250
- Earth:Neoglaciation
- Earth:Older Dryas – Geological period of the Earth
- Earth:Oldest Dryas – Abrupt climatic cooling event during the last glacial retreat
- Earth:Shutdown of thermohaline circulation – Effect of global warming on a major ocean circulation.
- Earth:Timeline of glaciation – Chronology of the major ice ages of the Earth
References
- ↑ Zalloua, Pierre A.; Matisoo-Smith, Elizabeth (6 January 2017). "Mapping Post-Glacial expansions: The Peopling of Southwest Asia" (in en). Scientific Reports 7: 40338. doi:10.1038/srep40338. ISSN 2045-2322. PMID 28059138. Bibcode: 2017NatSR...740338P.
- ↑ Rasmussen, S. O.; Andersen, K. K.; Svensson, A. M.; Steffensen, J. P.; Vinther, B. M.; Clausen, H. B.; Siggaard-Andersen, M.-L.; Johnsen, S. J. et al. (2006). "A new Greenland ice core chronology for the last glacial termination" (in en). Journal of Geophysical Research 111 (D6): D06102. doi:10.1029/2005JD006079. ISSN 0148-0227. Bibcode: 2006JGRD..111.6102R. https://epic.awi.de/id/eprint/12532/1/Ras2005a.pdf.
- ↑ Buizert, C.; Gkinis, V.; Severinghaus, J. P.; He, F.; Lecavalier, B. S.; Kindler, P.; Leuenberger, M.; Carlson, A. E. et al. (2014-09-05). "Greenland temperature response to climate forcing during the last deglaciation" (in en). Science 345 (6201): 1177–1180. doi:10.1126/science.1254961. ISSN 0036-8075. PMID 25190795. Bibcode: 2014Sci...345.1177B.
- ↑ Meissner, K. J. (2007). "Younger Dryas: A data to model comparison to constrain the strength of the overturning circulation.". Geophys. Res. Lett. 34 (21): L21705. doi:10.1029/2007GL031304. Bibcode: 2007GeoRL..3421705M.
- ↑ Carlson, A. E. (2013). "The Younger Dryas Climate Event". Encyclopedia of Quaternary Science. 3. Elsevier. pp. 126–34. http://people.oregonstate.edu/~carlsand/carlson_encyclopedia_Quat_2013_YD.pdf.
- ↑ 6.0 6.1 6.2 Björck, S. (2007) Younger Dryas oscillation, global evidence. In S. A. Elias, (Ed.): Encyclopedia of Quaternary Science, Volume 3, pp. 1987–1994. Elsevier B.V., Oxford.
- ↑ 7.0 7.1 Bjorck, S.; Kromer, B.; Johnsen, S.; Bennike, O.; Hammarlund, D.; Lemdahl, G.; Possnert, G.; Rasmussen, T. L. et al. (15 November 1996). "Synchronized terrestrial-atmospheric deglacial records around the North Atlantic". Science 274 (5290): 1155–1160. doi:10.1126/science.274.5290.1155. PMID 8895457. Bibcode: 1996Sci...274.1155B. https://semanticscholar.org/paper/6c8a7c21ab9f810d9e094e1a1a1f848403ea013d.
- ↑ Andersson, Gunnar (1896) (in Swedish). Svenska växtvärldens historia. Stockholm: P. A. Norstedt & Söner.
- ↑ Hartz, N.; Milthers, V. (1901). "Det senglacie ler i Allerød tegelværksgrav" (in Danish). Meddelelser Dansk Geologisk Foreningen (Bulletin of the Geological Society of Denmark) 2 (8): 31–60. https://babel.hathitrust.org/cgi/pt?id=inu.32000004344760&view=1up&seq=247.
- ↑ Mangerud, Jan; Andersen, Svend T.; Berglund, Björn E.; Donner, Joakim J. (16 January 2008). "Quaternary stratigraphy of Norden, a proposal for terminology and classification". Boreas 3 (3): 109–126. doi:10.1111/j.1502-3885.1974.tb00669.x.
- ↑ Pettit, Paul; White, Mark (2012). The British Palaeolithic: Human Societies at the Edge of the Pleistocene World. Abingdon, UK: Routledge. p. 477. ISBN 978-0-415-67455-3.
- ↑ Stuiver, Minze; Grootes, Pieter M.; Braziunas, Thomas F. (November 1995). "The GISP2 δ18O Climate Record of the Past 16,500 Years and the Role of the Sun, Ocean, and Volcanoes". Quaternary Research 44 (3): 341–354. doi:10.1006/qres.1995.1079. Bibcode: 1995QuRes..44..341S.
- ↑ Seppä, H.; Birks, H. H.; Birks, H. J. B. (2002). "Rapid climatic changes during the Greenland stadial 1 (Younger Dryas) to early Holocene transition on the Norwegian Barents Sea coast". Boreas 31 (3): 215–225. doi:10.1111/j.1502-3885.2002.tb01068.x.
- ↑ Walker, M. J. C. (2004). "A Lateglacial pollen record from Hallsenna Moor, near Seascale, Cumbria, NW England, with evidence for arid conditions during the Loch Lomond (Younger Dryas) Stadial and early Holocene". Proceedings of the Yorkshire Geological Society 55: 33–42. doi:10.1144/pygs.55.1.33.
- ↑ Björck, Svante; Walker, Michael J. C.; Cwynar, Les C.; Johnsen, Sigfus; Knudsen, Karen-Luise; Lowe, J. John; Wohlfarth, Barbara (July 1998). "An event stratigraphy for the Last Termination in the North Atlantic region based on the Greenland ice-core record: a proposal by the INTIMATE group". Journal of Quaternary Science 13 (4): 283–292. doi:10.1002/(SICI)1099-1417(199807/08)13:4<283::AID-JQS386>3.0.CO;2-A. Bibcode: 1998JQS....13..283B.
- ↑ 16.0 16.1 16.2 16.3 Alley, Richard B. (2000). "The Younger Dryas cold interval as viewed from central Greenland". Quaternary Science Reviews 19 (1): 213–226. doi:10.1016/S0277-3791(99)00062-1. Bibcode: 2000QSRv...19..213A.
- ↑ 17.0 17.1 Alley, Richard B. et al. (1993). "Abrupt increase in Greenland snow accumulation at the end of the Younger Dryas event". Nature 362 (6420): 527–529. doi:10.1038/362527a0. Bibcode: 1993Natur.362..527A.
- ↑ Choi, Charles Q. (2 December 2009). "Big Freeze: Earth Could Plunge into Sudden Ice Age". http://www.livescience.com/environment/091202-fast-ice-age.html. Retrieved 2 December 2009.
- ↑ 19.0 19.1 Severinghaus, Jeffrey P. et al. (1998). "Timing of abrupt climate change at the end of the Younger Dryas interval from thermally fractionated gases in polar ice". Nature 391 (6663): 141–146. doi:10.1038/34346. Bibcode: 1998Natur.391..141S.
- ↑ Atkinson, T. C. et al. (1987). "Seasonal temperatures in Britain during the past 22,000 years, reconstructed using beetle remains". Nature 325 (6105): 587–592. doi:10.1038/325587a0. Bibcode: 1987Natur.325..587A.
- ↑ Yu, Z.; Eicher, U. (2001). "Three amphi-Atlantic century-scale cold events during the Bølling-Allerød warm period". Géographie Physique et Quaternaire 55 (2): 171–179. doi:10.7202/008301ar.
- ↑ The relatively rapid changes from cold conditions to warm interglacials are called terminations. They are numbered from the most recent termination as I and with increasing value (II, III, and so forth) into the past. Termination I is the end Marine Isotope Stage 2 (MIS2); Termination II is the end of Marine Isotope Stage 6 (MIS6); Termination III is the end of Marine Isotope Stage 8 (MIS8); Termination III is the end of Marine Isotope Stage 10 (MIS10), and so forth. For an example, see Pleistocene glacial terminations triggered by synchronous changes in Southern and Northern Hemisphere insolation: The insolation canon hypothesis. by K.G. Schulz and R.E. Zeebe.
- ↑ 23.0 23.1 Bradley, R. (2015) Paleoclimatology: Reconstructing Climates of the Quaternary, 3rd ed.Academic Press: Kidlington, Oxford ISBN:978-0-12-386913-5
- ↑ Eglinton, G., A.B. Stuart, A. Rosell, M. Sarnthein, U. Pflaumann, and R. Tiedeman (1992) Molecular record of secular sea surface temperature changes on 100-year timescales for glacial terminations I, II and IV. Nature. 356:423–426.
- ↑ 25.0 25.1 Chen, S., Y. Wang, X. Kong, D. Liu, H. Cheng, and R.L. Edwards. (2006) A possible Younger Dryas-type event during Asian monsoonal Termination 3. Science China Earth Sciences. 49(9):982–990.
- ↑ Sima, A., A. Paul, and M. Schulz (2004) The Younger Dryas—an intrinsic feature of late Pleistocene climate change at millennial timescales. Earth Planetary Science Letters. 222:741–750.
- ↑ Xiaodong, D.; Liwei, Z.; Shuji, K. (2014). "A Review on the Younger Dryas Event". Advances in Earth Science 29 (10): 1095–1109.
- ↑ 28.0 28.1 Sissons, J. B. (1979). "The Loch Lomond stadial in the British Isles". Nature 280 (5719): 199–203. doi:10.1038/280199a0. Bibcode: 1979Natur.280..199S.
- ↑ Dansgaard, W. et al. (1989). "The abrupt termination of the Younger Dryas climate event". Nature 339 (6225): 532–534. doi:10.1038/339532a0. Bibcode: 1989Natur.339..532D.
- ↑ Kobashia, Takuro et al. (2008). "4 ± 1.5 °C abrupt warming 11,270 years ago identified from trapped air in Greenland ice". Earth and Planetary Science Letters 268 (3–4): 397–407. doi:10.1016/j.epsl.2008.01.032. Bibcode: 2008E&PSL.268..397K.
- ↑ Taylor, K. C. (1997). "The Holocene-Younger Dryas transition recorded at Summit, Greenland". Science 278 (5339): 825–827. doi:10.1126/science.278.5339.825. Bibcode: 1997Sci...278..825T. https://cloudfront.escholarship.org/dist/prd/content/qt9c8680t0/qt9c8680t0.pdf.
- ↑ Spurk, M. (1998). "Revisions and extension of the Hohenheim oak and pine chronologies: New evidence about the timing of the Younger Dryas/Preboreal transition". Radiocarbon 40 (3): 1107–1116. doi:10.1017/S0033822200019159.
- ↑ Gulliksen, Steinar et al. (1998). "A calendar age estimate of the Younger Dryas-Holocene boundary at Krakenes, western Norway". Holocene 8 (3): 249–259. doi:10.1191/095968398672301347. Bibcode: 1998Holoc...8..249G.
- ↑ Hughen, Konrad A. et al. (2000). "Synchronous Radiocarbon and Climate Shifts During the Last Deglaciation". Science 290 (5498): 1951–1954. doi:10.1126/science.290.5498.1951. PMID 11110659. Bibcode: 2000Sci...290.1951H.
- ↑ Mike Walker & others (3 October 2008). "Formal definition and dating of the GSSP, etc.". Journal of Quaternary Science (John Wiley & Sons Ltd) 24 (1): 3–17. doi:10.1002/jqs.1227. Bibcode: 2009JQS....24....3W. http://www.stratigraphy.org/GSSP/Holocene.pdf. Retrieved 11 November 2019.
- ↑ Muschitiello, F., and B. Wohlfarth (2015) Time-transgressive environmental shifts across Northern Europe at the onset of the Younger Dryas. Quaternary Science Reviews. 109:49–56.
- ↑ Nakagawa, T; Kitagawa, H.; Yasuda, Y.; Tarasov, P.E.; Nishida, K.; Gotanda, K.; Sawai, Y.; Yangtze River, Civilization Program Members (2003). "Asynchronous climate changes in the North Atlantic and Japan during the last termination". Science 299 (5607): 688–691. doi:10.1126/science.1078235. PMID 12560547. Bibcode: 2003Sci...299..688N.
- ↑ Partin, J.W., T.M. Quinn, C.-C. Shen, Y. Okumura, M.B. Cardenas, F.P. Siringan, J.L. Banner, K. Lin, H.-M. Hu and F.W Taylor (2014) Gradual onset and recovery of the Younger Dryas abrupt climate event in the tropics. Nature Communications. Received 10 October 2014 | Accepted 13 July 2015 | Published 2 September 2015
- ↑ "Climate Change 2001: The Scientific Basis". Grida.no. Archived from the original on 24 September 2015. https://web.archive.org/web/20150924023910/http://www.grida.no/climate/ipcc_tar/wg1/073.htm. Retrieved 2015-11-24.
- ↑ "New clue to how last ice age ended". Archived from the original on 11 September 2010. https://web.archive.org/web/20100911052653/https://www.sciencedaily.com/releases/2010/09/100908132214.htm.
- ↑ Thompson, L. G. et al. (2000). "Ice-core palaeoclimate records in tropical South America since the Last Glacial Maximum". Journal of Quaternary Science 15 (4): 377–394. doi:10.1002/1099-1417(200005)15:4<377::AID-JQS542>3.0.CO;2-L. Bibcode: 2000JQS....15..377T.
- ↑ A., Elias, Scott; J., Mock, Cary (2013-01-01). Encyclopedia of quaternary science. Elsevier. pp. 126–127. ISBN 9780444536426. OCLC 846470730.
- ↑ Denniston, R. F; Gonzalez, L. A; Asmerom, Y; Polyak, V; Reagan, M. K; Saltzman, M. R (2001-12-25). "A high-resolution speleothem record of climatic variability at the Allerød–Younger Dryas transition in Missouri, central United States". Palaeogeography, Palaeoclimatology, Palaeoecology 176 (1–4): 147–155. doi:10.1016/S0031-0182(01)00334-0. Bibcode: 2001PPP...176..147D.
- ↑ Friele, P. A.; Clague, J. J. (2002). "Younger Dryas readvance in Squamish river valley, southern Coast mountains, British Columbia". Quaternary Science Reviews 21 (18–19): 1925–1933. doi:10.1016/S0277-3791(02)00081-1. Bibcode: 2002QSRv...21.1925F.
- ↑ Vacco, David A.; Clark, Peter U.; Mix, Alan C.; Cheng, Hai; Edwards, R. Lawrence (2005-09-01). "A Speleothem Record of Younger Dryas Cooling, Klamath Mountains, Oregon, USA". Quaternary Research 64 (2): 249–256. doi:10.1016/j.yqres.2005.06.008. ISSN 0033-5894. Bibcode: 2005QuRes..64..249V.
- ↑ Hassett, Brenna (2017). Built on Bones: 15,000 Years of Urban Life and Death. London: Bloomsbury Sigma. pp. 20–21. ISBN 978-1-4729-2294-6.
- ↑ Gill, J. L.; Williams, J. W.; Jackson, S. T.; Lininger, K. B.; Robinson, G. S. (19 November 2009). "Pleistocene Megafaunal Collapse, Novel Plant Communities, and Enhanced Fire Regimes in North America". Science 326 (5956): 1100–1103. doi:10.1126/science.1179504. PMID 19965426. Bibcode: 2009Sci...326.1100G. http://doc.rero.ch/record/210391/files/PAL_E4398.pdf.
- ↑ Miller, D. Shane; Gingerich, Joseph A. M. (March 2013). "Regional variation in the terminal Pleistocene and early Holocene radiocarbon record of eastern North America". Quaternary Research 79 (2): 175–188. doi:10.1016/j.yqres.2012.12.003. ISSN 0033-5894. Bibcode: 2013QuRes..79..175M.
- ↑ 49.0 49.1 49.2 Meltzer, David J.; Holliday, Vance T. (2010-03-01). "Would North American Paleoindians have Noticed Younger Dryas Age Climate Changes?". Journal of World Prehistory 23 (1): 1–41. doi:10.1007/s10963-009-9032-4. ISSN 0892-7537.
- ↑ Peteet, D. (1995-01-01). "Global Younger Dryas?". Quaternary International 28: 93–104. doi:10.1016/1040-6182(95)00049-o. Bibcode: 1995QuInt..28...93P.
- ↑ Shuman, Bryan; Bartlein, Patrick; Logar, Nathaniel; Newby, Paige; Webb III, Thompson (September 2002). "Parallel climate and vegetation responses to the early Holocene collapse of the Laurentide Ice Sheet". Quaternary Science Reviews 21 (16–17): 1793–1805. doi:10.1016/s0277-3791(02)00025-2. Bibcode: 2002QSRv...21.1793S.
- ↑ Dorale, J. A.; Wozniak, L. A.; Bettis, E. A.; Carpenter, S. J.; Mandel, R. D.; Hajic, E. R.; Lopinot, N. H.; Ray, J. H. (2010). "Isotopic evidence for Younger Dryas aridity in the North American midcontinent". Geology 38 (6): 519–522. doi:10.1130/g30781.1. Bibcode: 2010Geo....38..519D.
- ↑ Williams, John W.; Post*, David M.; Cwynar, Les C.; Lotter, André F.; Levesque, André J. (2002-11-01). "Rapid and widespread vegetation responses to past climate change in the North Atlantic region". Geology 30 (11): 971–974. doi:10.1130/0091-7613(2002)030<0971:rawvrt>2.0.co;2. ISSN 0091-7613. Bibcode: 2002Geo....30..971W.
- ↑ Dieffenbacher-Krall, Ann C.; Borns, Harold W.; Nurse, Andrea M.; Langley, Geneva E.C.; Birkel, Sean; Cwynar, Les C.; Doner, Lisa A.; Dorion, Christopher C. et al. (2016-03-01). "Younger Dryas Paleoenvironments and Ice Dynamics in Northern Maine: A Multi-Proxy, Case History". Northeastern Naturalist 23 (1): 67–87. doi:10.1656/045.023.0105. ISSN 1092-6194.
- ↑ 55.0 55.1 Liu, Yao; Andersen, Jennifer J.; Williams, John W.; Jackson, Stephen T. (March 2012). "Vegetation history in central Kentucky and Tennessee (USA) during the last glacial and deglacial periods". Quaternary Research 79 (2): 189–198. doi:10.1016/j.yqres.2012.12.005. ISSN 0033-5894. Bibcode: 2013QuRes..79..189L.
- ↑ 56.0 56.1 56.2 Griggs, Carol; Peteet, Dorothy; Kromer, Bernd; Grote, Todd; Southon, John (2017-04-01). "A tree-ring chronology and paleoclimate record for the Younger Dryas–Early Holocene transition from northeastern North America". Journal of Quaternary Science 32 (3): 341–346. doi:10.1002/jqs.2940. ISSN 1099-1417. Bibcode: 2017JQS....32..341G.
- ↑ 57.0 57.1 A., Elias, Scott; J., Mock, Cary (2013). Encyclopedia of quaternary science. Elsevier. pp. 126–132. ISBN 9780444536426. OCLC 846470730.
- ↑ Grimm, Eric C.; Watts, William A.; Jacobson Jr., George L.; Hansen, Barbara C. S.; Almquist, Heather R.; Dieffenbacher-Krall, Ann C. (September 2006). "Evidence for warm wet Heinrich events in Florida". Quaternary Science Reviews 25 (17–18): 2197–2211. doi:10.1016/j.quascirev.2006.04.008. Bibcode: 2006QSRv...25.2197G.
- ↑ Yu, Zicheng; Eicher, Ulrich (1998). "Abrupt Climate Oscillations During the Last Deglaciation in Central North America". Science 282 (5397): 2235–2238. doi:10.1126/science.282.5397.2235. PMID 9856941. Bibcode: 1998Sci...282.2235Y.
- ↑ 60.0 60.1 Ofer., Bar-Yosef; J., Shea, John; 1964–, Lieberman, Daniel; Research., American School of Prehistoric (2009). Transitions in prehistory : essays in honor of Ofer Bar-Yosef. Oxbow Books. ISBN 9781842173404. OCLC 276334680.
- ↑ Nordt, Lee C.; Boutton, Thomas W.; Jacob, John S.; Mandel, Rolfe D. (2002-09-01). "C4 Plant Productivity and Climate-CO2 Variations in South-Central Texas during the Late Quaternary". Quaternary Research 58 (2): 182–188. doi:10.1006/qres.2002.2344. Bibcode: 2002QuRes..58..182N.
- ↑ Lowell, Thomas V; Larson, Graham J; Hughes, John D; Denton, George H (1999-03-25). "Age verification of the Lake Gribben forest bed and the Younger Dryas Advance of the Laurentide Ice Sheet". Canadian Journal of Earth Sciences 36 (3): 383–393. doi:10.1139/e98-095. ISSN 0008-4077. Bibcode: 1999CaJES..36..383L.
- ↑ Williams, John W.; Shuman, Bryan N.; Webb, Thompson (2001-12-01). "Dissimilarity Analyses of Late-Quaternary Vegetation and Climate in Eastern North America". Ecology 82 (12): 3346–3362. doi:10.1890/0012-9658(2001)082[3346:daolqv2.0.co;2]. ISSN 1939-9170.
- ↑ 1982–, Eren, Metin I.. Hunter-gatherer behavior : human response during the Younger Dryas. ISBN 9781598746037. OCLC 907959421.
- ↑ MacLeod, David Matthew; Osborn, Gerald; Spooner, Ian (2006-04-01). "A record of post-glacial moraine deposition and tephra stratigraphy from Otokomi Lake, Rose Basin, Glacier National Park, Montana". Canadian Journal of Earth Sciences 43 (4): 447–460. doi:10.1139/e06-001. ISSN 0008-4077. Bibcode: 2006CaJES..43..447M. https://semanticscholar.org/paper/8a787a2d416e39a5c0dab628776d38883a438f64.
- ↑ 66.0 66.1 Mumma, Stephanie Ann; Whitlock, Cathy; Pierce, Kenneth (2012-04-01). "A 28,000 year history of vegetation and climate from Lower Red Rock Lake, Centennial Valley, Southwestern Montana, USA". Palaeogeography, Palaeoclimatology, Palaeoecology 326: 30–41. doi:10.1016/j.palaeo.2012.01.036. Bibcode: 2012PPP...326...30M.
- ↑ 67.0 67.1 Brunelle, Andrea; Whitlock, Cathy (July 2003). "Postglacial fire, vegetation, and climate history in the Clearwater Range, Northern Idaho, USA". Quaternary Research 60 (3): 307–318. doi:10.1016/j.yqres.2003.07.009. ISSN 0033-5894. Bibcode: 2003QuRes..60..307B.
- ↑ "Precise Cosmogenic 10Be Measurements in Western North America: Support for a Global Younger Dryas Cooling Event". https://www.researchgate.net/publication/230891096.
- ↑ Reasoner, Mel A.; Osborn, Gerald; Rutter, N. W. (1994-05-01). "Age of the Crowfoot advance in the Canadian Rocky Mountains: A glacial event coeval with the Younger Dryas oscillation". Geology 22 (5): 439–442. doi:10.1130/0091-7613(1994)022<0439:AOTCAI>2.3.CO;2. ISSN 0091-7613. Bibcode: 1994Geo....22..439R.
- ↑ Reasoner, Mel A.; Jodry, Margret A. (2000-01-01). "Rapid response of alpine timberline vegetation to the Younger Dryas climate oscillation in the Colorado Rocky Mountains, USA". Geology 28 (1): 51–54. doi:10.1130/0091-7613(2000)28<51:RROATV>2.0.CO;2. ISSN 0091-7613. Bibcode: 2000Geo....28...51R.
- ↑ Briles, Christy E.; Whitlock, Cathy; Meltzer, David J. (January 2012). "Last glacial–interglacial environments in the southern Rocky Mountains, USA and implications for Younger Dryas-age human occupation". Quaternary Research 77 (1): 96–103. doi:10.1016/j.yqres.2011.10.002. ISSN 0033-5894. Bibcode: 2012QuRes..77...96B.
- ↑ Davis, P. Thompson; Menounos, Brian; Osborn, Gerald (2009-10-01). "Holocene and latest Pleistocene alpine glacier fluctuations: a global perspective". Quaternary Science Reviews. Holocene and Latest Pleistocene Alpine Glacier Fluctuations: A Global Perspective 28 (21): 2021–2033. doi:10.1016/j.quascirev.2009.05.020. Bibcode: 2009QSRv...28.2021D.
- ↑ Osborn, Gerald; Gerloff, Lisa (1997-01-01). "Latest pleistocene and early Holocene fluctuations of glaciers in the Canadian and northern American Rockies". Quaternary International 38: 7–19. doi:10.1016/s1040-6182(96)00026-2. Bibcode: 1997QuInt..38....7O.
- ↑ Feng, Weimin; Hardt, Benjamin F.; Banner, Jay L.; Meyer, Kevin J.; James, Eric W.; Musgrove, MaryLynn; Edwards, R. Lawrence; Cheng, Hai et al. (2014-09-01). "Changing amounts and sources of moisture in the U.S. southwest since the Last Glacial Maximum in response to global climate change". Earth and Planetary Science Letters 401: 47–56. doi:10.1016/j.epsl.2014.05.046. Bibcode: 2014E&PSL.401...47F.
- ↑ Barron, John A.; Heusser, Linda; Herbert, Timothy; Lyle, Mitch (2003-03-01). "High-resolution climatic evolution of coastal northern California during the past 16,000 years". Paleoceanography 18 (1): 1020. doi:10.1029/2002pa000768. ISSN 1944-9186. Bibcode: 2003PalOc..18.1020B.
- ↑ Kienast, Stephanie S.; McKay, Jennifer L. (2001-04-15). "Sea surface temperatures in the subarctic northeast Pacific reflect millennial-scale climate oscillations during the last 16 kyrs". Geophysical Research Letters 28 (8): 1563–1566. doi:10.1029/2000gl012543. ISSN 1944-8007. Bibcode: 2001GeoRL..28.1563K.
- ↑ Mathewes, Rolf W. (1993-01-01). "Evidence for Younger Dryas-age cooling on the North Pacific coast of America". Quaternary Science Reviews 12 (5): 321–331. doi:10.1016/0277-3791(93)90040-s. Bibcode: 1993QSRv...12..321M.
- ↑ 78.0 78.1 Vacco, David A.; Clark, Peter U.; Mix, Alan C.; Cheng, Hai; Edwards, R. Lawrence (September 2005). "A Speleothem Record of Younger Dryas Cooling, Klamath Mountains, Oregon, USA". Quaternary Research 64 (2): 249–256. doi:10.1016/j.yqres.2005.06.008. ISSN 0033-5894. Bibcode: 2005QuRes..64..249V.
- ↑ Friele, Pierre A.; Clague, John J. (2002-10-01). "Younger Dryas readvance in Squamish river valley, southern Coast mountains, British Columbia". Quaternary Science Reviews 21 (18): 1925–1933. doi:10.1016/s0277-3791(02)00081-1. Bibcode: 2002QSRv...21.1925F.
- ↑ Kovanen, Dori J. (2002-06-01). "Morphologic and stratigraphic evidence for Allerød and Younger Dryas age glacier fluctuations of the Cordilleran Ice Sheet, British Columbia, Canada and Northwest Washington, U.S.A". Boreas 31 (2): 163–184. doi:10.1111/j.1502-3885.2002.tb01064.x. ISSN 1502-3885.
- ↑ HEINE, JAN T. (1998-12-01). "Extent, Timing, and Climatic Implications of Glacier Advances Mount Rainier, Washington, U.s.a., at the Pleistocene/Holocene Transition". Quaternary Science Reviews 17 (12): 1139–1148. doi:10.1016/s0277-3791(97)00077-2. Bibcode: 1998QSRv...17.1139H.
- ↑ Grigg, Laurie D.; Whitlock, Cathy (May 1998). "Late-Glacial Vegetation and Climate Change in Western Oregon". Quaternary Research 49 (3): 287–298. doi:10.1006/qres.1998.1966. ISSN 0033-5894. Bibcode: 1998QuRes..49..287G.
- ↑ Grigg, Laurie D.; Whitlock, Cathy; Dean, Walter E. (July 2001). "Evidence for Millennial-Scale Climate Change During Marine Isotope Stages 2 and 3 at Little Lake, Western Oregon, U.S.A". Quaternary Research 56 (1): 10–22. doi:10.1006/qres.2001.2246. ISSN 0033-5894. Bibcode: 2001QuRes..56...10G. https://digitalcommons.unl.edu/usgsstaffpub/248.
- ↑ Hershler, Robert; Madsen, D. B.; Currey, D. R. (2002-12-11). "Great Basin Aquatic Systems History" (in English). Smithsonian Contributions to the Earth Sciences 33 (33): 1–405. doi:10.5479/si.00810274.33.1. ISSN 0081-0274. Bibcode: 2002SCoES..33.....H. https://semanticscholar.org/paper/d3edba883664df995663589d6b98120f944015b1.
- ↑ Briles, Christy E.; Whitlock, Cathy; Bartlein, Patrick J. (July 2005). "Postglacial vegetation, fire, and climate history of the Siskiyou Mountains, Oregon, USA". Quaternary Research 64 (1): 44–56. doi:10.1016/j.yqres.2005.03.001. ISSN 0033-5894. Bibcode: 2005QuRes..64...44B.
- ↑ Cole, Kenneth L.; Arundel, Samantha T. (2005). "Carbon isotopes from fossil packrat pellets and elevational movements of Utah agave plants reveal the Younger Dryas cold period in Grand Canyon, Arizona". Geology 33 (9): 713. doi:10.1130/g21769.1. Bibcode: 2005Geo....33..713C. https://semanticscholar.org/paper/a1cba720312e405d8415478c6dce3915aa50a8fc.
- ↑ Bar-Yosef, O. and A. Belfer-Cohen: "Facing environmental crisis. Societal and cultural changes at the transition from the Younger Dryas to the Holocene in the Levant." In: The Dawn of Farming in the Near East. Edited by R.T.J. Cappers and S. Bottema, pp. 55–66. Studies in Early Near Eastern Production, Subsistence and Environment 6. Berlin: Ex oriente.
- ↑ Mithen, Steven J.: After The Ice: A Global Human History, 20,000–5000 BC, pages 46–55. Harvard University Press paperback edition, 2003.
- ↑ Munro, N. D. (2003). "Small game, the younger dryas, and the transition to agriculture in the southern levant". Mitteilungen der Gesellschaft für Urgeschichte 12: 47–64. http://www.anth.uconn.edu/faculty/munro/assets/Mitteilungen.pdf.
- ↑ Balter, Michael (2010). "Archaeology: The Tangled Roots of Agriculture". Science 327 (5964): 404–406. doi:10.1126/science.327.5964.404. PMID 20093449.
- ↑ 91.0 91.1 Blanchon, P. (2011a) Meltwater Pulses. In: Hopley, D. (Ed), Encyclopedia of Modern Coral Reefs: Structure, form and process. Springer-Verlag Earth Science Series, p. 683-690. ISBN:978-90-481-2638-5
- ↑ Blanchon, P. (2011b) Backstepping. In: Hopley, D. (Ed), Encyclopedia of Modern Coral Reefs: Structure, form and process. Springer-Verlag Earth Science Series, p. 77-84. ISBN:978-90-481-2638-5
- ↑ Blanchon, P., and Shaw, J. (1995) Reef drowning during the last deglaciation: evidence for catastrophic sea-level rise and icesheet collapse. Geology, 23:4–8.
- ↑ 94.0 94.1 Bard E., Hamelin B., and Delanghe-Sabatier D. (2010) Deglacial meltwater Pulse 1B and Younger Dryas Sea Levels Revisited with Boreholes at Tahiti Science. 327:1235–1237.
- ↑ Lohne, Ø. S.; Bondevik, S.; Mangeruda, J.; Svendsena, J. I. (2007). "Sea-level fluctuations imply that the Younger Dryas ice-sheet expansion in western Norway commenced during the Allerød". Quaternary Science Reviews 26 (17–18): 2128–2151. doi:10.1016/j.quascirev.2007.04.008. Bibcode: 2007QSRv...26.2128L.
- ↑ Broecker, Wallace S. (2006). "Was the Younger Dryas Triggered by a Flood?". Science 312 (5777): 1146–1148. doi:10.1126/science.1123253. PMID 16728622. https://semanticscholar.org/paper/42288c83cd71bc7051793716f2e8f3c58650ceac.
- ↑ Murton, Julian B.; Bateman, Mark D.; Dallimore, Scott R.; Teller, James T.; Yang, Zhirong (2010). "Identification of Younger Dryas outburst flood path from Lake Agassiz to the Arctic Ocean" (in en). Nature 464 (7289): 740–743. doi:10.1038/nature08954. ISSN 0028-0836. PMID 20360738. Bibcode: 2010Natur.464..740M.
- ↑ Keigwin, L. D.; Klotsko, S.; Zhao, N.; Reilly, B.; Giosan, L.; Driscoll, N. W. (2018). "Deglacial floods in the Beaufort Sea preceded Younger Dryas cooling" (in en). Nature Geoscience 11 (8): 599–604. doi:10.1038/s41561-018-0169-6. ISSN 1752-0894. Bibcode: 2018NatGe..11..599K.
- ↑ Wang, Luo; Jiang, Wenying; Jiang, Dabang. (2018). "Prolonged Heavy Snowfall During the Younger Dryas". Journal of Geophysical Research: Atmospheres 123 (24): 137489. doi:10.1029/2018JD029271. Bibcode: 2018JGRD..12313748W.
- ↑ Eisenman, I.; Bitz, C. M.; Tziperman, E. (2009). "Rain driven by receding ice sheets as a cause of past climate change". Paleoceanography 24 (4): PA4209. doi:10.1029/2009PA001778. Bibcode: 2009PalOc..24.4209E. https://semanticscholar.org/paper/f7b5a8cfb9b3144758f4d5486c6755eb4f387951.
- ↑ LaViolette PA (2011). "Evidence for a Solar Flare Cause of the Pleistocene Mass Extinction" (PDF). Radiocarbon 53 (2): 303–323. doi:10.1017/S0033822200056575. https://journals.uair.arizona.edu/index.php/radiocarbon/article/view/3464/pdf. Retrieved 20 April 2012.
- ↑ Biello, David (2 January 2009). "Did a Comet Hit Earth 12,000 Years Ago?". Scientific American (Nature America, Inc.). https://www.scientificamerican.com/article/did-a-comet-hit-earth-12900-years-ago/.
Shipman, Matt (25 September 2012). "New research findings consistent with theory of impact event 12,900 years ago". Phys.org (Science X network). https://phys.org/news/2012-09-theory-impact-event-years.html. - ↑ "Very high-temperature impact melt products as evidence for cosmic airbursts and impacts 12,900 years ago". Proc. Natl. Acad. Sci. U.S.A. 109 (28): E1903–12. July 2012. doi:10.1073/pnas.1204453109. PMID 22711809. Bibcode: 2012PNAS..109E1903B.
- ↑ Pinter, Nicholas; Scott, Andrew C.; Daulton, Tyrone L.; Podoll, Andrew; Koeberl, Christian; Anderson, R. Scott; Ishman, Scott E. (2011). "The Younger Dryas impact hypothesis: A requiem". Earth-Science Reviews 106 (3–4): 247–264. doi:10.1016/j.earscirev.2011.02.005. Bibcode: 2011ESRv..106..247P.
- ↑ M. Boslough; K. Nicoll; V. Holliday; T. L. Daulton; D. Meltzer; N. Pinter; A. C. Scott; T. Surovell et al. (2012). Arguments and Evidence Against a Younger Dryas Impact Event. 198. 13–26. doi:10.1029/2012gm001209. ISBN 9781118704325.
- ↑ Daulton, TL; Amari, S; Scott, AC; Hardiman, MJ; Pinter, N; Anderson, R.S. (2017). "Comprehensive analysis of nanodiamond evidence reported to support the Younger Dryas Impact Hypothesis". Journal of Quaternary Science 32 (1): 7–34. doi:10.1002/jqs.2892. Bibcode: 2017JQS....32....7D. https://researchportal.port.ac.uk/portal/en/publications/comprehensive-analysis-of-nanodiamond-evidence-reported-to-support-the-younger-dryas-impact-hypothesis(f675f063-5d32-4cac-9b83-e7ce6a9432d6).html.
- ↑ "Chronological evidence fails to support claim of an isochronous widespread layer of cosmic impact indicators dated to 12,800 years ago". Proc. Natl. Acad. Sci. U.S.A. 111 (21): E2162–71. May 2014. doi:10.1073/pnas.1401150111. PMID 24821789. Bibcode: 2014PNAS..111E2162M.
- ↑ Kinze, Charles R. (26 Aug 2014). "Nanodiamond-Rich Layer across Three Continents Consistent with Major Cosmic Impact at 12,800 Cal BP". Journal of Geology 122 (9/2014): 475–506. doi:10.1086/677046. ISSN 0022-1376. Bibcode: 2014JG....122..475K. https://cloudfront.escholarship.org/dist/prd/content/qt7vz406nv/qt7vz406nv.pdf?t=nwp5c3.
- ↑ Cohen, Julie (2014-08-28). "Nanodiamonds Are Forever | The UCSB Current". News.ucsb.edu. http://www.news.ucsb.edu/2014/014368/nanodiamonds-are-forever#sthash.Jz8DHJU3.dpuf. Retrieved 2015-11-24.
- ↑ Wolbach, Wendy S.; Ballard, Joanne P.; Mayewski, Paul A.; Adedeji, Victor; Bunch, Ted E. (2018). "Extraordinary Biomass-Burning Episode and Impact Winter Triggered by the Younger Dryas Cosmic Impact ∼12,800 Years Ago. 1. Ice Cores and Glaciers". Journal of Geology 126 (2): 165–184. doi:10.1086/695703. Bibcode: 2018JG....126..165W. https://semanticscholar.org/paper/ed0863a4d001c50bf9d1473f1fe09630c8be63ac.
- ↑ Wolbach, Wendy S.; Ballard, Joanne P.; Mayewski, Paul A.; Parnell, Andrew C.; Cahill, Niamh (2018). "Extraordinary Biomass-Burning Episode and Impact Winter Triggered by the Younger Dryas Cosmic Impact ∼12,800 Years Ago. 2. Lake, Marine, and Terrestrial Sediments". Journal of Geology 126 (2): 185–205. doi:10.1086/695704. Bibcode: 2018JG....126..185W. https://semanticscholar.org/paper/95973f135b3919130883692b8e50466c9d5d0417.
- ↑ Thackeray, J. Francis; Scott, Louis; Pieterse, P. (2019) (in en). The Younger Dryas interval at Wonderkrater (South Africa) in the context of a platinum anomaly. http://wiredspace.wits.ac.za/handle/10539/28129. Retrieved 9 October 2019.
- ↑ "African evidence support Younger Dryas Impact Hypothesis" (in en). ScienceDaily. https://www.sciencedaily.com/releases/2019/10/191002110329.htm. Retrieved 9 October 2019.
- ↑ Pino, Mario; Abarzúa, Ana M.; Astorga, Giselle; Martel-Cea, Alejandra; Cossio-Montecinos, Nathalie; Navarro, R. Ximena; Lira, Maria Paz; Labarca, Rafael et al. (2019). "Sedimentary record from Patagonia, southern Chile supports cosmic-impact triggering of biomass burning, climate change, and megafaunal extinctions at 12.8 ka" (in en). Scientific Reports 9 (1): 4413. doi:10.1038/s41598-018-38089-y. PMID 30867437. Bibcode: 2019NatSR...9.4413P.
- ↑ Firestone, R. B.; West, A.; Kennett, J. P.; Becker, L.; Bunch, T. E.; Revay, Z. S.; Schultz, P. H.; Belgya, T. et al. (2007). "Evidence for an extraterrestrial impact 12,900 years ago that contributed to the megafaunal extinctions and the Younger Dryas cooling" (in en). Proceedings of the National Academy of Sciences 104 (41): 16016–16021. doi:10.1073/pnas.0706977104. PMID 17901202. Bibcode: 2007PNAS..10416016F.
- ↑ "Evidence from Chile Supports Younger Dryas Extraterrestrial Impact Hypothesis | Geology, Paleontology | Sci-News.com". http://www.sci-news.com/paleontology/evidence-chile-younger-dryas-extraterrestrial-impact-hypothesis-07012.html. Retrieved 9 October 2019.
- ↑ Frechen, J. (1959). "Die Tuffe des Laacher Vulkangebietes als quartargeologische Leitgesteine and Zeitmarken". Fortschritte der Geologie Rheinland and Westfalen 4: 363–370.
- ↑ Bogaard, P. v. d.; Schmincke, H. -U. (October 1984). "The eruptive center of the late quaternary Laacher see tephra". Geologische Rundschau 73 (3): 933–980. doi:10.1007/bf01820883. ISSN 0016-7835. Bibcode: 1984GeoRu..73..933B.
- ↑ 119.0 119.1 Baales, Michael; Jöris, Olaf; Street, Martin; Bittmann, Felix; Weninger, Bernhard; Wiethold, Julian (November 2002). "Impact of the Late Glacial Eruption of the Laacher See Volcano, Central Rhineland, Germany". Quaternary Research 58 (3): 273–288. doi:10.1006/qres.2002.2379. ISSN 0033-5894. Bibcode: 2002QuRes..58..273B. https://semanticscholar.org/paper/838e4e58eb1bddbc2f8d75b9e723a84051e577c5.
- ↑ Schmincke, Hans-Ulrich; Park, Cornelia; Harms, Eduard (November 1999). "Evolution and environmental impacts of the eruption of Laacher See Volcano (Germany) 12,900 a BP". Quaternary International 61 (1): 61–72. doi:10.1016/s1040-6182(99)00017-8. ISSN 1040-6182. Bibcode: 1999QuInt..61...61S.
- ↑ Rach, O.; Brauer, A.; Wilkes, H.; Sachse, D. (2014-01-19). "Delayed hydrological response to Greenland cooling at the onset of the Younger Dryas in western Europe". Nature Geoscience 7 (2): 109–112. doi:10.1038/ngeo2053. ISSN 1752-0894. Bibcode: 2014NatGe...7..109R. https://doi.pangaea.de/10.1594/PANGAEA.823778.
- ↑ 122.0 122.1 122.2 122.3 122.4 Baldini, James U. L.; Brown, Richard J.; Mawdsley, Natasha (2018-07-04). "Evaluating the link between the sulfur-rich Laacher See volcanic eruption and the Younger Dryas climate anomaly" (in English). Climate of the Past 14 (7): 969–990. doi:10.5194/cp-14-969-2018. ISSN 1814-9324. Bibcode: 2018CliPa..14..969B.
- ↑ Brauer, Achim; Haug, Gerald H.; Dulski, Peter; Sigman, Daniel M.; Negendank, Jörg F. W. (August 2008). "An abrupt wind shift in western Europe at the onset of the Younger Dryas cold period". Nature Geoscience 1 (8): 520–523. doi:10.1038/ngeo263. ISSN 1752-0894. Bibcode: 2008NatGe...1..520B.
- ↑ Lane, Christine S.; Brauer, Achim; Blockley, Simon P. E.; Dulski, Peter (2013-12-01). "Volcanic ash reveals time-transgressive abrupt climate change during the Younger Dryas". Geology 41 (12): 1251–1254. doi:10.1130/G34867.1. ISSN 0091-7613. Bibcode: 2013Geo....41.1251L. https://semanticscholar.org/paper/08fb2270958b07f0f5d8b0fa308ef9e849703106.
- ↑ Baldini, James U.L.; Brown, Richard J.; McElwaine, Jim N. (2015-11-30). "Was millennial scale climate change during the Last Glacial triggered by explosive volcanism?". Scientific Reports 5 (1): 17442. doi:10.1038/srep17442. ISSN 2045-2322. PMID 26616338. Bibcode: 2015NatSR...517442B.
- ↑ Miller, Gifford H.; Geirsdóttir, Áslaug; Zhong, Yafang; Larsen, Darren J.; Otto-Bliesner, Bette L.; Holland, Marika M.; Bailey, David A.; Refsnider, Kurt A. et al. (January 2012). "Abrupt onset of the Little Ice Age triggered by volcanism and sustained by sea-ice/ocean feedbacks". Geophysical Research Letters 39 (2): n/a. doi:10.1029/2011gl050168. ISSN 0094-8276. Bibcode: 2012GeoRL..39.2708M. https://www.pure.ed.ac.uk/ws/files/11341002/Abrupt2012.pdf.
- ↑ Zhong, Y.; Miller, G. H.; Otto-Bliesner, B. L.; Holland, M. M.; Bailey, D. A.; Schneider, D. P.; Geirsdottir, A. (2010-12-31). "Centennial-scale climate change from decadally-paced explosive volcanism: a coupled sea ice-ocean mechanism". Climate Dynamics 37 (11–12): 2373–2387. doi:10.1007/s00382-010-0967-z. ISSN 0930-7575. Bibcode: 2011ClDy...37.2373Z.
- ↑ Kobashi, Takuro; Menviel, Laurie; Jeltsch-Thömmes, Aurich; Vinther, Bo M.; Box, Jason E.; Muscheler, Raimund; Nakaegawa, Toshiyuki; Pfister, Patrik L. et al. (2017-05-03). "Volcanic influence on centennial to millennial Holocene Greenland temperature change". Scientific Reports 7 (1): 1441. doi:10.1038/s41598-017-01451-7. ISSN 2045-2322. PMID 28469185. Bibcode: 2017NatSR...7.1441K.
- ↑ Sternai, Pietro; Caricchi, Luca; Castelltort, Sébastien; Champagnac, Jean-Daniel (2016-02-19). "Deglaciation and glacial erosion: A joint control on magma productivity by continental unloading". Geophysical Research Letters 43 (4): 1632–1641. doi:10.1002/2015gl067285. ISSN 0094-8276. Bibcode: 2016GeoRL..43.1632S.
- ↑ Zielinski, Gregory A.; Mayewski, Paul A.; Meeker, L. David; Grönvold, Karl; Germani, Mark S.; Whitlow, Sallie; Twickler, Mark S.; Taylor, Kendrick (1997-11-30). "Volcanic aerosol records and tephrochronology of the Summit, Greenland, ice cores". Journal of Geophysical Research: Oceans 102 (C12): 26625–26640. doi:10.1029/96jc03547. ISSN 0148-0227. Bibcode: 1997JGR...10226625Z. http://digitalcommons.library.umaine.edu/cgi/viewcontent.cgi?article=1263&context=ers_facpub.
- ↑ Nowell, David A. G.; Jones, M. Chris; Pyle, David M. (2006). "Episodic Quaternary volcanism in France and Germany". Journal of Quaternary Science 21 (6): 645–675. doi:10.1002/jqs.1005. ISSN 0267-8179. Bibcode: 2006JQS....21..645N.
- ↑ Cheng, Hai; Edwards, R. Lawrence; Broecker, Wallace S.; Denton, George H.; Kong, Xinggong; Wang, Yongjin; Zhang, Rong; Wang, Xianfeng (2009-10-09). "Ice Age Terminations". Science 326 (5950): 248–252. doi:10.1126/science.1177840. ISSN 0036-8075. PMID 19815769. Bibcode: 2009Sci...326..248C. https://semanticscholar.org/paper/14f44bb74ee0bc98c4da8f59207aa7b3ad5ae806.
- ↑ 133.0 133.1 133.2 133.3 Brakenridge, G. Robert. Core-Collapse Supernovae and the Younger Dryas/Terminal Rancholabrean Extinctions. Elsevier, 2011, Retrieved 23 September 2018
- ↑ Brannen, Peter (2018). The Ends of the World: Volcanic Apocalypses, Lethal Oceans, and Our Quest to Understand Earth's Past Mass Extinctions (1st ed.). Ecco. ISBN 978-0062364814.
External links
- "Study Confirms Mechanism for Current Shutdowns, European Cooling". Oregon State University. 2007. Archived from the original on 17 December 2010. https://web.archive.org/web/20101217183132/http://oregonstate.edu/dept/ncs/newsarch/2007/Apr07/currents.html. Retrieved 11 April 2011.
- Broecker WS (1999). "What If the Conveyor Were to Shut Down?". GSA Today 9 (1): 1–7. http://faculty.washington.edu/wcalvin/teaching/Broecker99.html. Retrieved 11 April 2011.
- Calvin WH (January 1998). "The Great Climate Flip-flop". The Atlantic Monthly 281: 47–64. http://williamcalvin.com/1990s/1998AtlanticClimate.htm. Retrieved 11 April 2011.
- "Arctic freshwater forcing of the Younger Dryas cold reversal". Nature 435 (7042): 662–665. June 2005. doi:10.1038/nature03617. PMID 15931219. Bibcode: 2005Natur.435..662T. Archived from the original on 21 August 2015. https://web.archive.org/web/20150821001847/http://ic.ucsc.edu/~acr/BeringResources/Articles%20of%20interest/Central%20Artic/Tarasov%20and%20Peltier%202005.pdf.
- Acosta et al., 2018. Climate change and peopling of the Neotropics during the Pleistocene-Holocene transition. Boletín de la Sociedad Geológica Mexicana. Boletín de la Sociedad Geológica Mexicana
- Cometary Debris May Have Destroyed Paleolithic Settlement 12,800 Years Ago, on: sci-news, Jul. 2, 2020