Biology:Effects of climate change on agriculture
There are numerous effects of climate change on agriculture, many of which are making it harder for agricultural activities to provide global food security. Rising temperatures and changing weather patterns often result in lower crop yields due to water scarcity caused by drought, heat waves and flooding.[5] These effects of climate change can also increase the currently-rare risk of several regions suffering simultaneous crop failures, which would have significant consequences for the global food supply.[6][7] Many pests and plant diseases are also expected to either become more prevalent or to spread to new regions. The world's livestock are also expected to be affected by many of the same issues, from greater heat stress to animal feed shortfalls and the spread of parasites and vector-borne diseases.[5]:746
The increased atmospheric CO
2 level from human activities causes a CO
2 fertilization effect, which offsets some of the detrimental effects on agriculture due to climate change. However, it has little effect on C4 crops like maize,[8] and comes at the expense of lower levels of essential micronutrients.[5]:717 On the coasts, some agricultural land is expected to be lost to sea level rise, while melting glaciers could result in less irrigation water being available.[9] On the other hand, more arable land may become available as frozen land thaws. Other impacts include erosion and changes in soil fertility and the length of growing seasons. Negative impacts on food safety from bacteria like Salmonella or mycotoxin-producing fungi, also increase as the climate warms, increasing costs and food loss.[5]
There has been extensive research into the impact of climate change on individual crops, particularly the four staple crops—corn (maize), rice, wheat and soybeans—that are responsible for around two-thirds of all calories consumed by humans (both directly and indirectly as animal feed).[10] Yet, there are still other important uncertainties involved - from future population growth, which will only increase global food demand for the foreseeable future,[11] to the related yet largely separate challenges of soil erosion and groundwater depletion. On the other hand, a range of improvements to agricultural yields, collectively known as the Green Revolution, has already lifted yields per unit of land area by between 250% and 300% since the 1960, and some of that progress may be expected to continue.[5]:727
Altogether, there is a consensus that global food security will change relatively little in the near-term: 720 million to 811 million people were considered undernourished in 2021, with ~200,000 at a "catastrophic" level of food insecurity.[12] Compared to that figure, climate change is expected to place an extra 8 to 80 million people at risk of hunger by 2050 (depending on the intensity of future warming and the effectiveness of adaptation measures).[5]:717 Continued economic and agricultural development will likely improve food security for hundreds of millions of people by then.[13][11] Research and predictions that extend further into the future (to 2100 and beyond) are rather limited, and some scientists have voiced concerns about the impact on food security from currently-unprecedented extreme weather events enabled by the future climate.[14][15][16] Nevertheless, published scientific literature includes no expectation of a widespread global famine within the 21st century.[17][18]
A range of measures for climate change adaptation may reduce the risk of negative climate change impacts on agriculture. Those measures include changes in management practices, agricultural innovation, institutional changes, and climate-smart agriculture.[19] To create a sustainable food system, these measures are considered as essential as changes needed to reduce global warming in general.[20][21]
Direct impacts from changing weather patterns
Observed changes in adverse weather conditions
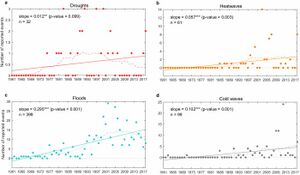
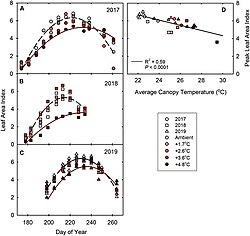
Agriculture is sensitive to weather, and major events like heatwaves or droughts or heavy rains (also known as low and high precipitation extremes) can cause substantial losses. For example, Australia 's farmers are very likely to suffer losses during the El Nino weather conditions, while 2003 European heat wave led to 13 billion euros in uninsured agriculture losses.[24] Climate change is known to increase the frequency and severity of heatwaves, and to make precipitation less predictable and more prone to extremes, but since climate change attribution is still a relatively new field, connecting specific weather events and the shortfalls they cause to climate change over natural variability is often difficult. Exceptions include West Africa, where the climate-induced intensification of extreme weather was found to have already decreased millet yields by 10–20%, and sorghum yields 5–15%. Similarly, it was found that climate change had intensified drought conditions in Southern Africa in 2007, which elevated food prices and caused "acute food insecurity" in the country of Lesotho. Agriculture in Southern Africa was also adversely affected by drought after climate change intensified the impacts of 2014–2016 El Niño event.[5]:724
In Europe, between 1950 and 2019, heat extremes have become more frequent and also more likely to occur consecutively, while cold extremes have declined. At the same time, Northern Europe and much of Eastern Europe was found to experience extreme precipitation more often, while the Mediterranean became more affected by drought.[25] Similarly, the severity of heatwave and drought impacts on European crop production was found to have tripled over a 50-year period – from losses of 2.2% during 1964–1990 to losses of 7.3% in 1991–2015.[26][22] In the summer of 2018, heat waves probably linked to climate change greatly reduced average yield in many parts of the world, especially Europe. During the month of August, more crop failures resulted in a rise in global food prices.[27]
On the other hand, floods, often linked to climate change, have also had notable adverse effects on agriculture in the recent years. In May 2019, floods shortened corn planting season in the Midwestern United States, lowering the projected yield from 15 billion bushels to 14.2.[28] During the 2021 European floods, estimates pointed to severe damage to the agricultural sector of Belgium, one of the countries hardest hit by the floods, including long-term effects like soil erosion.[29] In China , 2023 research found that extreme rainfall had cost the country about 8% of its rice output over the two preceding decades. This was considered comparable to losses caused by extreme heat over this period.[30]
Projected impacts from temperature increase

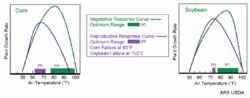
Changes in temperature and weather patterns will alter areas suitable for farming.[33] The current prediction is that temperatures will increase and precipitation will decrease in arid and semi-arid regions (Middle East, Africa, Australia , Southwest United States, and Southern Europe).[33][34] In addition, crop yields in tropical regions will be negatively affected by the projected moderate increase in temperature (1-2 °C) expected to occur during the first half of the century.[24] During the second half of the century, further warming is projected to decrease crop yields in all regions including Canada and the Northern United States.[34] Many staple crops are extremely sensitive to heat and when temperatures rise over 36 °C (97 °F), soybean seedlings are killed and corn pollen loses its vitality.[35][36]
Higher winter temperatures and more frost-free days in some regions can currently be disruptive, as they can cause phenological mismatch between flowering time of plants and the activity of pollinators, threatening their reproductive success.[37] In the longer term, however, they would result in longer growing seasons.[38][39] For example, a 2014 study found that maize yields in the Heilongjiang region of China increased by between 7 and 17% per decade as a result of rising temperatures.[40] On the other hand, a year 2017 meta-analysis comparing data from four different methods of estimating impact of warming (two types of climate model, statistical regressions and field experiments where land around certain crops was warmed by a certain amount to compare them with the controls) concluded that on a global scale, warming alone has consistently negative impacts on the yields of four most important crops, suggesting that any increases would be down to precipitation changes and the CO2 fertilization effect.[10]
Heat stress of livestock
Changes in agricultural water availability and reliability

2 fertilization effect, but at times not enough to avoid events like the 2020–2023 Horn of Africa drought.[3]
Both droughts and floods contribute to decreases in crop yields. On average, climate change increases the overall amount of water contained in the atmosphere by 7% per every 1 °C (1.8 °F), thus increasing precipitation.[42][43] However, this increase in precipitation is not distributed evenly in space (atmospheric circulation patterns already cause different areas to receive different amounts of rainfall) or time: heavy rainfall, with the potential to cause floods, becomes more frequent. This means that under the probable mid-range climate change scenario,[44][45] SSP2-4.5, precipitation events globally will become larger by 11.5%, yet the time between them will increase by an average of 5.1%. Under the highest-emission scenario SSP5-8.5, there will be an 18.5% increase in size of events and 9.6% increase in the duration between them. At the same time, water losses by plants through evotranspiration will increase almost everywhere due to higher temperatures.[41] While the CO
2 fertilization effect also reduces such losses by plants, it depends on the area's climate which effect will dominate. As such, the 2020–2023 Horn of Africa drought has been primarily attributed to the great increase in evotranspiration exacerbating the impact of persistent low rainfall, which would have been more manageable in the cooler preindustrial climate.[3]
In total, this means that droughts have been occurring more frequently on average because of climate change. Africa, southern Europe, the Middle East, most of the Americas, Australia, South and Southeast Asia are the parts of the globe where droughts are expected to become more frequent and intense in spite of the global increase in precipitation.[46] Droughts disturb terrestrial precipitation, evaporation and soil moisture,[47][48] and these impacts can be aggravated by population growth and urban expansion spurring on increased demand for water.[49] The ultimate outcome is water scarcity, which results in crop failures and the loss of pasture grazing land for livestock,[50] exacerbating pre-existing poverty in developing countries and leading to malnutrition and potentially famine.[51][35]
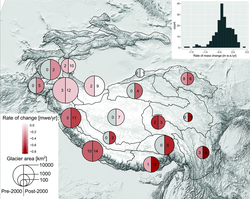
Irrigation of crops is able to reduce or even remove the impacts on yields of lower rainfall and higher temperatures - through localised cooling. However, using water resources for irrigation has downsides and is expensive.[33] Further, some sources of irrigation water may become less reliable. This includes irrigation driven by water runoff from glaciers during the summer, as there has already been an observed retreat of glaciers since 1850, and it is expected to continue, depleting the glacial ice and reducing or outright eliminating runoff.[53] In Asia, global warming of 1.5 °C (2.7 °F) will reduce the ice mass of Asia's high mountains by about 29-43%,:[54] Approximately 2.4 billion people live in the drainage basin of the Himalayan rivers:[55] In India alone, the river Ganges provides water for drinking and farming for more than 500 million people.[56][57] In the Indus River watershed, these mountain water resources contribute to up to 60% of irrigation outside of the monsoon season, and an additional 11% of total crop production.[9] Since Effects of climate change on the water cycle are projected to substantially increase precipitation in all but the westernmost parts of the watershed, the loss of the glaciers is expected to be offset: however, agriculture in the region will become more reliable on monsoon than ever, and hydropower generation would become less predictable and reliable.[58][52][59]
Impacts on plants caused by increasing atmospheric CO2 and methane
Elevated atmospheric carbon dioxide affects plants in a variety of ways. Elevated CO2 increases crop yields and growth through an increase in photosynthetic rate, and it also decreases water loss as a result of stomatal closing.[60]
Higher yields due to CO2 fertilisation
Reduced nutritional value of crops

2 concentrations, reconstructed from multiple studies through a meta-analysis.[62] The elevated concentration in this figure, 689 ppm, is over 50% greater than the current levels, yet it is expected to be approached under the "mid-range" climate change scenarios, and will be surpassed in the high-emission one.[63]
Changes in atmospheric carbon dioxide may reduce the nutritional quality of some crops, with for instance wheat having less protein and less of some minerals.[64]:439[65] The nutritional quality of C3 plants (e.g. wheat, oats, rice) is especially at risk: lower levels of protein as well as minerals (for example zinc and iron) are expected.[5]:1379 Food crops could see a reduction of protein, iron and zinc content in common food crops of 3 to 17%.[66] This is the projected result of food grown under the expected atmospheric carbon-dioxide levels of 2050. Using data from the UN Food and Agriculture Organization as well as other public sources, the authors analyzed 225 different staple foods, such as wheat, rice, maize, vegetables, roots and fruits.[67]
The effect of increased levels of atmospheric carbon dioxide on the nutritional quality of plants is not limited only to the above-mentioned crop categories and nutrients. A 2014 meta-analysis has shown that crops and wild plants exposed to elevated carbon dioxide levels at various latitudes have lower density of several minerals such as magnesium, iron, zinc, and potassium.[62]
Studies using Free-Air Concentration Enrichment have also shown that increases in CO2 lead to decreased concentrations of micronutrients in crop and non-crop plants with negative consequences for human nutrition,[68][62] including decreased B vitamins in rice.[69][70] This may have knock-on effects on other parts of ecosystems as herbivores will need to eat more food to gain the same amount of protein.[71]
Empirical evidence shows that increasing levels of CO
2 result in lower concentrations of many minerals in plant tissues. Doubling CO
2 levels results in an 8% decline, on average, in the concentration of minerals.[62] Declines in magnesium, calcium, potassium, iron, zinc and other minerals in crops can worsen the quality of human nutrition. Researchers report that the CO
2 levels expected in the second half of the 21st century will likely reduce the levels of zinc, iron, and protein in wheat, rice, peas, and soybeans. Some two billion people live in countries where citizens receive more than 60 percent of their zinc or iron from these types of crops. Deficiencies of these nutrients already cause an estimated loss of 63 million life-years annually.[72][73]
Alongside a decrease in minerals, evidence shows that plants contain 6% more carbon, 15% less nitrogen, 9% less phosphorus, and 9% less sulfur at double CO
2 conditions. The increase in carbon is mostly attributed to carbohydrates without a structural role in plants – the human-digestable, calorie-providing starch and simple sugars. The decrease in nitrogen translates directly into a decrease in the protein content. As a result, higher CO
2 not only reduce a plant's micronutrients, but also the quality of its macronutrient combination.[62]
Increasing damages from surface-level ozone
Anthropogenic methane emissions have a significant contribution to warming due to the high global warming potential of methane. At the same time, methane also acts as a precursor to surface ozone, which is a significant air pollutant. Its effects include lowering physiological functions and therefore the yield and quality of crops.[5]:732 Following methane levels, tropospheric ozone levels "increased substantially since the late 19th century",[5]:732 and according to a 2016 estimate, the four major crops (see later section) experienced yield losses of 5±1.5% relative to a no-climate change scenario due to ozone increases alone, which is nearly half of the negative impacts caused by the other impacts of climate change (10.9±3.2%), and cancels out most of the CO
2 fertilization effect (6.5±1.0%).[5]:724
Changes in the extent and quality of agricultural land

Erosion and soil fertility
The warmer atmospheric temperatures observed over the past decades are expected to lead to a more vigorous hydrological cycle, including more extreme rainfall events. Erosion and soil degradation is more likely to occur. Soil fertility would also be affected by global warming. Increased erosion in agricultural landscapes from anthropogenic factors can occur with losses of up to 22% of soil carbon in 50 years.[75]
Climate change will also cause soils to warm. In turn, this could cause the soil microbe population size to dramatically increase 40–150%. Warmer conditions would favor growth of certain bacteria species, shifting the bacterial community composition. Elevated carbon dioxide would increase the growth rates of plants and soil microbes, slowing the soil carbon cycle and favoring oligotrophs, which are slower-growing and more resource efficient than copiotrophs.[76]
Agricultural land loss from sea level rise
A rise in the sea level would result in an agricultural land loss, in particular in areas such as South East Asia.[77] Erosion, submergence of shorelines, salinity of the water table due to the increased sea levels, could mainly affect agriculture through inundation of low-lying lands. Low-lying areas such as Bangladesh, India and Vietnam will experience major loss of rice crop if sea levels rise as expected by the end of the century. Vietnam for example relies heavily on its southern tip, where the Mekong Delta lies, for rice planting. A one-metre rise in sea level will cover several square kilometres of rice paddies in Vietnam.[78]
Besides simply flooding agricultural land, sea level rise can also cause saltwater intrusion into freshwater wells, particularly if they are already below sea level. Once the concentration of saltwater exceeds 2-3%, the well becomes unusable. Notably, areas along an estimated 15% of the US coastline already have the majority of local groundwater below the sea level.[74]
Thawing of potentially arable land
Climate change may increase the amount of arable land by reducing the amount of frozen land. A 2005 study reports that temperature in Siberia has increased three-degree Celsius in average since 1960 (much more than the rest of the world).[79][needs update] However, reports about the impact of global warming on Russian agriculture[80] indicate conflicting probable effects: while they expect a northward extension of farmable lands,[81] they also warn of possible productivity losses and increased risk of drought.[82][needs update]
The Arctic region is expected to benefit from increased opportunities for agriculture and forestry.[83]
Response of insects, plant diseases and weeds
Climate change will alter pest, plant disease and weed distributions, with potential to reduce crop yields, including of staple crops like wheat, soybeans, and corn (maize).[84] Warmer temperatures can increase the metabolic rate and number of breeding cycles of insect populations.[84] Historically, cold temperatures at night and in the winter months would kill off insects, bacteria and fungi. The warmer, wetter winters are promoting fungal plant diseases like wheat rusts (stripe and brown/leaf) and soybean rust to travel northward.[85] The increasing incidence of flooding and heavy rains also promotes the growth of various other plant pests and diseases.[86]
Insect pollinators and pests
Climate change is expected to have a negative impact on many insects, greatly reducing their species distribution and thus increasing their risk of going extinct.[87] Around 9% of agricultural production is dependent in some way on insect pollination,[88] and some pollinator species are also adversely affected, with wild bumblebees known to be particularly vulnerable to recent warming.[89][90]
At the same time, insects are the most diverse animal taxa, and some species will benefit from the changes, including notable agricultural pests and disease vectors.[85] Insects that previously had only two breeding cycles per year could gain an additional cycle if warm growing seasons extend, causing a population boom. Temperate places and higher latitudes are more likely to experience a dramatic change in insect populations:[91] for instance, the Mountain Pine Beetle epidemic in British Columbia, Canada had killed millions of pine trees, partially because the winters were not cold enough to slow or kill the growing beetle larvae.[35] Likewise, potato tuber moth and Colorado potato beetle are predicted to spread into areas currently too cold for them.[92]
Further, effects of climate change on the water cycle often mean that both wet seasons and drought seasons will become more intense. Some insect species will breed more rapidly because they are better able to take advantage of such changes in conditions.[93] This includes certain insect pests, such as aphids and whiteflies:[35] similarly, locust swarms could also cause more damage as the result. A notable example was the 2019–2022 locust infestation focused on East Africa, considered the worst of its kind in many decades.[94][95]
The fall armyworm, Spodoptera frugiperda, is a highly invasive plant pest, which can cause have massive damage to crops, especially maize. In the recent years, it has spread to countries in Sub-Saharan Africa, and this spread is linked to climate change. It is expected that these highly invasive crop pests will spread to other parts of the planet since they have a high capacity to adapt to different environments.[4]
Invasive plant species
Weeds
A changing climate may favour the more biologically diverse weeds over the monocrops on many farms.[86] Characteristics of weeds such as their genetic diversity, cross-breeding ability, and fast-growth rates put them at an advantage in changing climates as these characteristics allow them to adapt readily in comparison to most farm's uniform crops, and give them a biological advantage.[35]
Weeds also undergo the same acceleration of cycles as cultivated crops, and would also benefit from CO2 fertilization. Since most weeds are C3 plants, they are likely to compete even more than now against C4 crops such as corn.[96] The increased CO
2 levels are also expected to increase the tolerance of weeds to herbicides, reducing their efficiency.[86] However, this may be counteracted by increased temperatures elevating their effectiveness.[96]
Plant pathogens
Currently, pathogens result in losses of 10-16% of the global harvest and this level is likely to rise as plants are at an ever-increasing risk of exposure to pests and pathogens.[97] Research has shown that climate change may alter the developmental stages of plant pathogens that can affect crops. This includes several pathogens associated with potato blackleg disease (e.g. Dickeya), as they grow and reproduce faster at higher temperatures.[98] The warming is also expected to elevate food safety issues and food spoilage caused by mycotoxin-producing fungi, and bacteria such as Salmonella.[99]
Climate change would cause an increase in rainfall in some areas, which would lead to an increase of atmospheric humidity and the duration of the wet seasons. Combined with higher temperatures, these conditions could favour the development of fungal diseases, such as late blight,[100][85] or bacterial infections such as Ralstonia solanacearum, which may also be able to spread more easily through flash flooding.[92]
Climate change has the capability of altering pathogen and host interactions, specifically the rates of pathogen infection and the resistance of the host plant.[101] Also impacted by plant disease are the economic costs associated with growing different plants that might yield less profit as well as treating and managing already diseased crops.[102] For instance, soybean rust is a vicious plant pathogen that can kill off entire fields in a matter of days, devastating farmers and costing billions in agricultural losses.[35] Change in weather patterns and temperature due to climate change leads to dispersal of plant pathogens as hosts migrate to areas with more favourable conditions. This increases crop losses due to diseases.[99][85] For instance, aphids act as vectors for many potato viruses and will be able to spread further due to increased temperatures.[103]
Impacts on crop yields
Observed impacts
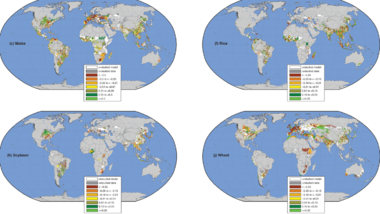
According to the IPCC Sixth Assessment Report from 2022, there is high confidence that in and of itself, climate change to date has left primarily negative impacts on both crop yields and quality of produce, although there has been some regional variation:[5]:724 more negative impacts have been observed for some crops in low-latitudes (maize and wheat), while positive impacts of climate change have been observed in some crops in high-latitudes (maize, wheat, and sugar beets).[105]:8 I.e. during the period 1981 to 2008, global warming has had negative impacts on wheat yield in especially tropical regions, with decreases in average global yields by 5.5%.[106] A study in 2019 tracked ~20,000 political units globally for 10 crops (maize, rice, wheat, soybean, barley, cassava, oil palm, rapeseed, sorghum and sugarcane), providing more detail on the spatial resolution and a larger number of crops than previously studied.[104] It found that crop yields across Europe, Sub-Saharan Africa and Australia had in general decreased because of climate change (compared to the baseline value of 2004–2008 average data), though exceptions are present. The impact of global climate change on yields of different crops from climate trends ranged from -13.4% (oil palm) to 3.5% (soybean). The study also showed that impacts are generally positive in Latin America. Impacts in Asia and Northern and Central America are mixed.[104]
While the Green Revolution had ensured the growth of overall crop production per land area of 250% to 300% since the 1960,[5]:727 with around 44% attributed to newer crop varieties alone,[107] it is believed this growth would have been even greater without the counteracting role of climate change on major crop yields over the same period. Between 1961 and 2021, global agricultural productivity could have been 21% greater than it actually was, if it did not have to contend with climate change. Such shortfalls would have affected food security of vulnerable populations the most:[5]:724 a study in 2019 showed that climate change has already increased the risk of food insecurity in many food insecure countries.[104] Even in developed countries such as Australia , extreme weather associated with climate change has been found to cause a wide range of cascading spillovers through supply chain disruption, in addition to its primary impact on fruit, vegetable and livestock sectors and the rural communities reliant on them.[108]
Between 1961 and 1985, cereal production more than doubled in developing nations, largely due to the development of irrigation, fertilizer, and seed varieties.[109] Even in the absence of further scientific/technological developments, many of the existing advancements have not been evenly distributed, and their spread from the developed world to the developing world is expected to drive some improvements on its own. Further, agricultural expansion has slowed down in the recent years, but this trend is widely expected to reverse in the future in order to maintain the global food supply under all but the most optimistic climate change scenarios consistent with the Paris Agreement.[110][63]
Generalized yield projections

In 2007, the IPCC Fourth Assessment Report had suggested that global production potential would increase up to around 3 °C (5.4 °F) of globally averaged warming, as productivity increases for cereals in high latitudes would outweigh decreases in the low latitudes and global aggregate yields of rain-fed agriculture would increase by 5–20% in the first half of the 21st century. Warming exceeding this level would very likely see global declines in yields.[111][112]:14–15 Since then, subsequent reports had been more negative on the global production potential.[5]
The US National Research Council assessed the literature on the effects of climate change on crop yields in 2011,[31] and provided central estimates for key crops.[31]:160 A meta-analysis in 2014 revealed consensus that yield is expected to decrease in the second half of the century, and with greater effect in tropical than temperate regions.[113]
Impacts on yields for four major crops
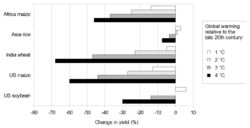
There is a large number of agricultural crops, but not all of them are equally important. Most climate change assessments focus on "four major crops" - maize (corn), rice, wheat and soybeans - which are consumed directly and indirectly, as animal feed (the main purpose of soybeans). The three cereals are collectively responsible for half of the total human calorie intake,[114] and together with soybeans, they account for two thirds.[10] Different methods have been used to project future yields of these crops, and by 2019, the consensus was that warming would lead to aggregate declines of the four. Maize and soybean would decrease with any warming, whereas rice and wheat production might peak at 3 °C (5.4 °F) of warming.[64]:453
In 2021, a paper which used an ensemble of 21 climate models estimated that under the most intense climate change scenario used at the time, RCP8.5, global yields of these four crops would decline by between 3–12% around 2050 and by 11–25% by the year 2100. The losses were concentrated in what are currently the major agricultural producers and exporters. For instance, even by 2050, some agricultural areas of Australia , Brazil , South Africa , Southeast China, Southern Europe and the United States would suffer production losses of mostly maize and soybeans exceeding 25%.[115] A similar finding - that some major "breadbaskets" would begin to see unequivocal impacts of climate change, both positive and negative, before the year 2040 - had been established in another study from the same year.[116] Since it represents the worst-case scenario of continually increasing emissions with no efforts to reduce them, RCP8.5 is often considered unrealistic,[117] and a less intense RCP4.5 scenario (which still leads to nearly 3 °C (5.4 °F) by century's end, far in excess of the Paris Agreement goals) is now usually considered a better match for the current trajectory.[44][45]
Maize
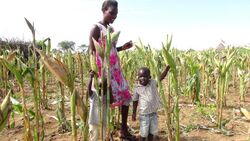
Out of the four crops, maize is considered the most vulnerable to warming, with one meta-analysis concluding that every 1 °C (1.8 °F) of global warming reduces maize yields by 7.4%.[10]
It is also a C4 carbon fixation plant, meaning that it experiences little benefit from the increased CO
2 levels.[8] When the results from modelling experiments comparing the combined output of latest earth system models and dedicated agricultural crop models were published in 2021, the most notable new finding was the substantial reduction in projected global yields of maize. While the previous generation suggested that under the low-warming scenario, maize productivity would increase by around 5% by the end of the century, the latest had shown a reduction of 6% under the equivalent scenario, SSP1-2.6. Under the high-emission SSP5-8.5, there was a global decline of 24% by 2100, as opposed to the earlier suggestion of a 1% increase.[116]
Rice
Studies indicate that on their own, temperature changes reduce global rice yields by 3.2% for every 1 °C (1.8 °F) of global warming.[10] Projections become more complicated once the changes in precipitation, CO
2 fertilization effect and other factors need to be taken into account: for instance, climate impacts on rice growth in East Asia had been a net positive so far,[5]:728 although 2023 research suggested that by the end of the century, China could lose up to 8% of its rice yield due to increases in extreme rainfall events alone.[30] As of 2021, global projections of rice yields from the most advanced climate and agricultural models were less consistent than they were for wheat and maize, and less able to identify a clear statistically significant trend.[116]
Wheat
Climate change impacts on rainfed wheat will vary depending on the region and local climatic conditions. Studies in Iran surrounding changes in temperature and rainfall are representative for several different parts of the world since there exists a wide range of climatic conditions. They range from temperate to hot-arid to cold semi-arid. Scenarios based on increasing temperature by up to 2.5 °C (4.5 °F) and rainfall decreases by up to 25% show wheat grain yield losses can be significant. The losses can be as much as 45% in temperate areas and over 50% in hot-arid areas. But in cold semi-arid areas yields can be increased somewhat (about 15%). Adaptation strategies with the most promise center around dates for seed planting. Late planting in November to January can have significant positive impacts on yields due to the seasonality of rainfall.[118] However, those experiments did not consider the effects of CO
2 increases.
Globally, temperature changes alone are expected to reduce annual wheat yield by 6% for every 1 °C (1.8 °F) of global warming.[10] However, other factors such as precipitation and the CO
2 fertilization effect benefit wheat yields far more. In November 2021, the results from modelling experiments comparing the combined output of latest earth system models and dedicated agricultural crop models were published. While it projected a consistent decrease in future global yields of maize, particularly under greater warming, it found the opposite for wheat yields. When the previous generation of models suggested a 9% increase in global wheat yields by 2100 under the high-emission scenario, the updated results indicate that under its highest-warming SSP5-8.5 scenario, they would increase by 18%.[116]
Soybeans

Studies have shown that when CO
2 levels rise, soybean leaves are less nutritious; therefore plant-eating beetles have to eat more to get their required nutrients.[35] In addition, soybeans are less capable of defending themselves against the predatory insects under high CO
2. The CO
2 diminishes the plant's jasmonic acid production, an insect-killing poison that is excreted when the plant senses it is being attacked. Without this protection, beetles are able to eat the soybean leaves freely, resulting in a lower crop yield.[35] This is not a problem unique to soybeans, and many plant species' defense mechanisms are impaired in a high CO
2 environment.[97]
Studies indicate that on their own, temperature changes reduce global soybean yields by 3.1% for every 1 °C (1.8 °F) of global warming.[10] These projections become more complicated once the changes in precipitation, CO
2 fertilization effect and other factors need to be taken into account: as of 2021, global projections of soybean yields from the most advanced climate and agricultural models were less able to establish a strong trend when compared to the projections for maize and wheat.[116]
Other crops
Climate change induced by increasing greenhouse gases is likely to differ across crops and countries.[119]
Millet and sorghum

Millet and sorghum are not as widely consumed as the four major crops, but they are crucial staples in many African countries. A paper published in the year 2022 found that under the highest-warming SSP5-8.5 scenario, changes in temperature and soil moisture would reduce the aggregate yields of millet, sorghum, maize and soybeans by between 9% and 32%, depending on the model. Notably, this was a less pessimistic result than in the earlier models, which the authors attributed to simulating soil moisture directly, rather than attempting to indirectly account for it by tracking precipitation changes caused by effects of climate change on the water cycle.[120]
Lentils (besides soybeans)
Climate change induced drought stress in Africa will likely lead to a reduction in the nutritional quality of the common bean.[121] This would primarily impact populations in poorer countries less able to compensate by eating more food, more varied diets, or possibly taking supplements.
Potatoes
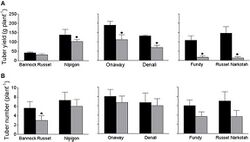
As well as affecting potatoes directly, climate change will also affect the distributions and populations of many potato diseases and pests. For instance, late blight is predicted to become a greater threat in some areas (e.g. in Finland [92]) and become a lesser threat in others (e.g. in the United Kingdom [123] Altogether, one 2003 estimate suggests that future (2040–2069) worldwide potato yield would be 18-32% lower than it was at the time, driven by declines in hotter areas like Sub-Saharan Africa,[92] unless farmers and potato cultivars can adapt to the new environment.[85]
Grapevines (wine production)
Impacts on livestock rearing
Global food security and undernutrition

Scientific understanding of how climate change would impact global food security has evolved over time. The latest IPCC Sixth Assessment Report in 2022 suggested that by 2050, the number of people at risk of hunger will increase under all scenarios by between 8 and 80 million people, with nearly all of them in Sub-Saharan Africa, South Asia and Central America. However, this comparison was done relative to a world where no climate change had occurred, and so it does not rule out the possibility of an overall reduction in hunger risk when compared to present-day conditions.[5]:717
The earlier Special Report on Climate Change and Land suggested that under a relatively high emission scenario (RCP6.0), cereals may become 1–29% more expensive in 2050 depending on the socioeconomic pathway.[64]:439 Compared to a scenario where climate change is absent, this would put between 1–181 million people with low income at risk of hunger.[64]
It is difficult to project the impact of climate change on utilization (protecting food against spoilage, being healthy enough to absorb nutrients, etc.) In 2016, a modelling study suggested that by mid-century, the most intense climate change scenario would reduce per capita global food availability by 3.2%, with a 0.7% decrease in red meat consumption and a 4% decrease in fruit and vegetable consumption. According to its numbers, 529,000 people would die between 2010 and 2050 as the result, primarily in South Asia and East Asia: two-thirds of those deaths would be caused by the lack of micronutrients from reduced fruit and vegetable supply, rather than outright starvation. Acting to slow climate change would reduce these projections by up to 71%.[125] Food prices are also expected to become more volatile.[126]
As of 2017, around 821 million people had suffered from hunger. This was equivalent to about 11% of the world population: regionally, this included 23.2% of sub-Saharan Africa, 16.5% of the Caribbean and 14.8% of South Asia.[13] In 2021, 720 million to 811 million people were considered undernourished in 2021 (of whom 200,000, 32.3 million and 112.3 million people were at a "catastrophic", "emergency" and "crisis" levels of food insecurity, respectively).[12]
In 2020, research suggested that the baseline projected level of socioeconomic development (Shared Socioeconomic Pathway 2) would reduce this number to 122 million globally by 2050, even as the population grows to reach 9.2 billion. The impact of climate change would at most increase this 2050 figure by around 80 million, and the negative impact could be reduced to 20 million through enabling easier food trade with measures such as eliminating tariffs.[13]
In 2021, a meta-analysis of 57 studies on food security was more pessimistic, suggesting that the year 2050 population at risk of hunger would be around 500 million under SSP2. Some variations of Shared Socioeconomic Pathways with high climate change and a lack of equitable global development instead resulted in an outright increase of global hunger by up to 30% from its 2010 levels.[11]
For the earlier IPCC Fourth Assessment Report in 2007, the analysis of four main SRES pathways had shown with medium confidence (about 50% certainty)[127]) that trends of social and economic development in three of them (A1, B1, B2) would see the number of undernourished people decline to 100-130 million people by the year 2080, while trends in A2 projected 770 million undernourished - similar to the contemporary (early 21st century) figures of ~700 million people. Once the impact of climate change implied by those scenarios was taken into account, A1, B1 and B2 scenarios would see 100-380 million undernourished by 2080 (still a major decline in hunger from 2006 levels), and A2 would see 740–1,300 million, although there was only low (20% certainty) to medium certainty in these figures.[128] Sub-Saharan Africa would likely overtake Asia as the world's most food-insecure region, primarily due to differing socioeconomic trends.[129]
Impacts of extreme weather and synchronized crop failures
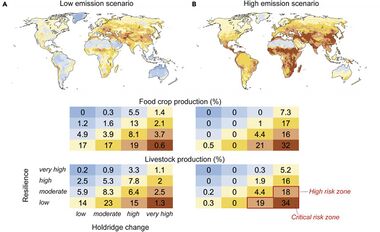
Some scientists consider the aforementioned projections of crop yields and food security of limited use, because in their view, they primarily model climate change as a change in mean climate state, and are not as well-equipped to consider climatic extremes. For instance, a paper published in 2021 had also attempted to calculate the number of people facing hunger in 2050 - but now on the assumption that a climate event with a 1% (i.e. once in 100 years) likelihood of occurring in the new climate (meaning it would have been effectively impossible in the present climate) were to impact that year. It estimated that such an event would increase the baseline number by 11–33% even in the low-emission scenario, and by 20–36% in the high-emission one. If such an event were to impact more vulnerable regions like South Asia, then they would have required triple their 2021 level of known food reserves to absorb the blow.[14]
Notably, other papers show that simulating recent historical extreme events in climate models, such as the 2003 European heatwave, typically results in lower impacts than what had been observed in the real world, indicating that the impacts of future extreme events are also likely to be underestimated.[15][130]
The difference between climatic mean and extremes may be particularly important for determining areas where agriculture may stop being viable. In 2021, a research team aimed to extend climate model projections of mean changes in temperature and the water cycle to the year 2500. They suggested that under the second-strongest warming scenario RCP6.0, land area capable of supporting four major temperate crops (maize, potato, soybean and wheat) would become about 11% smaller by 2100 and 18.3% smaller by 2500, while for major tropical crops (cassava, rice, sweet potato, sorghum, taro, and yam), it would decline by only 2.3% around 2100, yet by around 15% by 2500. Under the low-emission scenario RCP2.6, changes are much smaller, with around 3% decline in suitable land area for temperate crops by 2500 and an equivalent gain for tropical trops by then.[131]
Yet, another paper from 2021 suggested that by 2100, under the high-emission SSP5-8.5, 31% and 34% of the current crop and livestock production would leave what the authors have defined as a "safe climatic space": that is, those areas (most of South Asia and the Middle East, as well as parts of sub-Saharan Africa and Central America) would experience very rapid shift in Holdridge life zones (HLZ) and associated weather, while also being low in social resilience. Notably, a similar fraction of global crop and livestock production would also experience a large change in HLZ, but in more developed areas which would have better chances of adapting. In contrast, under the low-emissions SSP1-2.6, 5% and 8% of crop and livestock production would leave what is defined as the safe climatic space.[16]
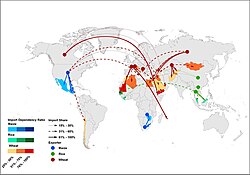
Also in 2021, it was suggested that the high-emission scenario would result in a 4.5-fold increase in the probability of breadbasket failures (defined as a yield loss of 10% or more) by 2030, which could then increase 25 times by 2050.[133] This corresponds to reaching 1.5 °C (2.7 °F) and 2 °C (3.6 °F) thresholds under that scenario: earlier research suggested that for maize, this would increase risks for multiple simultaneous breadbasket failures (yield loss of 10% or more) from 6% under the late-20th century climate to 40% and 54%, respectively.[6]
Some countries are particularly dependent on imports from certain exporters, meaning that a crop failure in those countries would hit them disproportionately. I.e. a ban on export of staple crops from Russia , Thailand and the United States alone would place around 200 million people (90% from Sub-Saharan Africa) at risk of starvation.[132]
Additionally, there is the issue of representing synchronization - where extreme climate events happen to strike multiple important producer regions around the same time. It was estimated that if hypothetically, every region with a synchronized growing season were to experience crop failure at the same time, it would cause losses of four major crops between 17% and 34%.[134] More realistically, analysis of historic data suggested that there have already been synchronized climate events associated with up to 20% yield losses.[135]
According to a 2016 estimate, if global maize, rice and wheat exports declined by 10%, 55 million people in 58 poor countries lose at least 5% of their food supply.[132] Further, two specific Rossby wave pattern are known to induce simultaneous heat extremes in either Eastern Asia, Eastern Europe and Central North America, or in Western Asia, Western Europe and Western Central North America, respectively. These heat extremes have already been shown to cause 3-4% declines in crop yield across the affected regions:[136] yet, concerningly, climate models overestimate the impacts of such historic events in North America and underestimate them elsewhere, simulating effectively no net yield loss.[7]
Labour and economic impacts
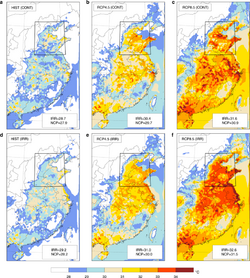
As extreme weather events become more common and more intense, floods and droughts can destroy crops and eliminate food supply, while disrupting agricultural activities and rendering workers jobless.[39][138] With more costs to the farmer, some will no longer find it financially feasible to farm: i.e. some farmers may choose to permanently leave drought-affected areas.[139] Agriculture employs the majority of the population in most low-income countries and increased costs can result in worker layoffs or pay cuts.[51] Other farmers will respond by raising their food prices; a cost which is directly passed on to the consumer and impacts the affordability of food. Some farms do not sell their produce but instead feed a family or community; without that food, people will not have enough to eat. This results in decreased production, increased food prices, and potential starvation in parts of the world.[140] The agriculture industry in India makes up 52% of their employment and the Canadian Prairies supply 51% of Canadian agriculture; any changes in the production of food crops from these areas could have profound effects on the economy.[38]
Notably, one estimate suggests that a warming of 3 °C (5.4 °F) relative to late 20th century (i.e. closer to 4 °C (7.2 °F) when compared to preindustrial temperatures - a level associated with the SSP5-8.5 scenario) would cause labour capacity in Sub-Saharan Africa and Southeast Asia to decline by 30 to 50%, as the number of days when outdoor workers experience heat stress increases: up to 250 days the worst-affected parts of these two continents and of Central and South America. This could then increase crop prices by around 5%.[5]:717:725
Similarly, North China Plain is also expected to be highly affected, in part due to the region's extensive irrigation networks resulting in unusually moist air. In scenarios without aggressive action to stop climate change, some heatwaves could become extreme enough to cause mass mortality in outdoor labourers, although they will remain relatively uncommon (up to around once per decade starting from 2l00 under the most extreme scenario).[137]
Further, the role of climate change in undernutrition and micronutrient deficiencies can be calculated as the loss of "years of full health".[5]:717One estimate presented in 2016 suggests that under the scenario of strong warming and low adaptation due to high global conflict and rivalry, such losses may take up 0.4% of the global GDP and 4% of the GDP in India and the South Asian region by the year 2100.[124]
Long-term predictions (beyond 2050)
There are fewer projections looking beyond 2050. In general, even as climate change would cause increasingly severe effects on food production, most scientists do not anticipate it to result in mass human mortality within this century.[18][17] This is in part because the studies also anticipate at least some continuation of the ongoing agricultural improvements, yet also because of agricultural expansion. For instance, a 2013 paper estimated that if the high warming of RCP 8.5 scenario was not alleviated by CO
2 fertilization effect, it would reduce aggregate yields by 17% by the year 2050: yet, it anticipated that this would be mostly offset through an 11% increase in cropland area.[110]
Similarly, one of the assumptions of Shared Socioeconomic Pathways is a significant increase in land allocated to agriculture (and a corresponding decrease in forest and "other natural land" area) in every pathway besides the SSP1 (officially subtitled "Sustainability" or "Taking the Green Road"), where the inverse occurs - and which has both the lowest level of future warming and the lowest projected population growth.[63]
Regional impacts
Africa
Asia
For East and Southeast Asia, an estimate in 2007 stated that crop yields could increase up to 20% by the mid-21st century.[112]:13 In Central and South Asia, projections suggested that yields might decrease by up to 30%, over the same time period. Taken together, the risk of hunger was projected to remain very high in several developing countries.[needs update]
Different Asian Countries gain various impact from climate change. China , for example, benefits from a 1.5 °C (2.7 °F) temperature increase scenario accompanying with carbon fertilization and leading to a 3% gain of US$18 billion per year; however, India will face two thirds of the continent's aggregate losses on agriculture because its high corp net revenue suffers from the high spring temperature.[141] In the Indo-Gangetic plain of India, heat stress and water availability are predicted to have significant negative impacts on yield of wheat.[142] Direct impacts of increased mean and maximum temperatures is predicted to reduce wheat yields by up to 10%. The impact of reduced availability of water for irrigation is more significant, running at yield losses up to 35%.
Due to climate change, livestock production will be decreased in Bangladesh by diseases, scarcity of forage, heat stress and breeding strategies.[143]
Australia and New Zealand
Without further adaptation to climate change, projected impacts would likely be substantial. By 2030, production from agriculture and forestry was projected to decline over much of southern and eastern Australia, and over parts of eastern New Zealand.[144] In New Zealand, initial benefits were projected close to major rivers and in western and southern areas.[144]
Europe
For Southern Europe, it was predicted in 2007 that climate change would reduce crop productivity.[112]:14 In Central and Eastern Europe, forest productivity was expected to decline. In Northern Europe, the initial effect of climate change was projected to increase crop yields. The 2019 European Environment Agency report "Climate change adaptation in the agricultural sector in Europe" again confirmed this. According to this 2019 report, projections indicate that yields of non-irrigated crops like wheat, corn and sugar beet would decrease in southern Europe by up to 50% by 2050 (under a high-end emission scenario). This could result in a substantial decrease in farm income by that date. Also farmland values are projected to decrease in parts of southern Europe by more than 80% by 2100, which could result in land abandonment. The trade patterns are also said to be impacted, in turn affecting agricultural income. Also, increased food demand worldwide could exert pressure on food prices in the coming decades.[145] In Ukraine , where temperatures are increasing throughout the year and precipitation is predicted to increase, winter wheat yields (wheat sown in winter) could increase by 20-40% in the north and northwestern regions by 2050, as compared to 2010.[146]
Latin America
The major agricultural products of Latin America include livestock and grains; such as maize, wheat, soybeans, and rice.[147][148] Increased temperatures and altered hydrological cycles are predicted to translate to shorter growing seasons, overall reduced biomass production, and lower grain yields.[148][149] Brazil , Mexico and Argentina alone contribute 70-90% of the total agricultural production in Latin America.[148] In these and other dry regions, maize production is expected to decrease.[147][148] A study summarizing a number of impact studies of climate change on agriculture in Latin America indicated that wheat is expected to decrease in Brazil, Argentina and Uruguay.[148] Livestock, which is the main agricultural product for parts of Argentina, Uruguay, southern Brazil, Venezuela, and Colombia is likely to be reduced.[147][148] Variability in the degree of production decrease among different regions of Latin America is likely.[147] For example, one 2003 study that estimated future maize production in Latin America predicted that by 2055 maize in eastern Brazil will have moderate changes while Venezuela is expected to have drastic decreases.[147]
Increased rainfall variability has been one of the most devastating consequences of climate change in Central America and Mexico. From 2009 to 2019, the region saw years of heavy rainfall in between years of below average rainfall.[150] The spring rains of May and June have been particularly erratic, posing issues for farmers plant their maize crops at the onset of the spring rains. Most subsistence farmers in the region have no irrigation and thus depend on the rains for their crops to grow. In Mexico, only 21% of farms are irrigated, leaving the remaining 79% dependent on rainfall.[151]
Suggested potential adaptation strategies to mitigate the impacts of global warming on agriculture in Latin America include using plant breeding technologies and installing irrigation infrastructure.[148]
North America
- Droughts are becoming more frequent and intense in arid and semiarid western North America as temperatures have been rising, advancing the timing and magnitude of spring snow melt floods and reducing river flow volume in summer.[152] Direct effects of climate change include increased heat and water stress, altered crop phenology, and disrupted symbiotic interactions. These effects may be exacerbated by climate changes in river flow, and the combined effects are likely to reduce the abundance of native trees in favour of non-native herbaceous and drought-tolerant competitors, reduce the habitat quality for many native animals, and slow litter decomposition and nutrient cycling. Climate change effects on human water demand and irrigation may intensify these effects.[153]
In Canada , notable increases are predicted for spring-sown wheat.[154]
Adaptation
Changes in management practices
Adaptation in agriculture is often not policy driven, but farmers make their own decisions in response to the situation they face. Changes in management practices might be the most important adaptation option.Changes in locations of agriculture and international trade in food commodities might also contribute to adaptation efforts.[citation needed]
Agricultural innovation
Agricultural innovation is essential to addressing the potential issues of climate change. This includes better management of soil, water-saving technology, matching crops to environments, introducing different crop varieties, crop rotations, appropriate fertilization use, and supporting community-based adaptation strategies.[140][155] On a government and global level, research and investments into agricultural productivity and infrastructure must be done to get a better picture of the issues involved and the best methods to address them. Government policies and programs must provide environmentally sensitive government subsidies, educational campaigns and economic incentives as well as funds, insurance and safety nets for vulnerable populations.[156][140][97] In addition, providing early warning systems, and accurate weather forecasts to poor or remote areas will allow for better preparation.[140]
Technological solutions to pests and weeds
There are a few proposed solutions to the issue of expanding pest populations (pest control). One proposed solution is to increase the number of pesticides used on crops.[157] This has the benefit of being relatively cost effective and simple, but may be ineffective. Many pest insects have been building up a pesticide resistance. Another proposed solution is to utilize biological control agents.[157] This includes things like planting rows of native vegetation in between rows of crops. This solution is beneficial in its overall environmental impact. Not only are more native plants getting planted, but pest insects are no longer building up an immunity to pesticides. However, planting additional native plants requires more room.
Institutional changes
A mere focus on agricultural technology will not be sufficient. Work is underway to enable and fund institutional change, and to develop dynamic policies for long-term climate change adaptation in agriculture.[158]
A 2013 study by the International Crops Research Institute for the Semi-Arid Tropics aimed to find science-based, pro-poor approaches and techniques that would enable Asia's agricultural systems to cope with climate change, while benefiting poor and vulnerable farmers. The study's recommendations ranged from improving the use of climate information in local planning and strengthening weather-based agro-advisory services, to stimulating diversification of rural household incomes and providing incentives to farmers to adopt natural resource conservation measures to enhance forest cover, replenish groundwater and use renewable energy.[159]
Climate-smart agriculture
Greenhouse gas emissions from agriculture
See also
Note: This topic belongs to "Global warming" portal
- 2022 food crises
- Agroecology
- Climate change and invasive species
- Climate change and meat production
- Climate resilience
- Effects of climate change
- Effects of climate change on fisheries
- Environmental issues with agriculture
- Special Report on Climate Change and Land (2019 IPCC report)
References
- ↑ "Climate change added $4bn to damage of Japan's Typhoon Hagibis" (in en). 18 May 2022. https://www.worldweatherattribution.org/climate-change-added-4bn-to-damage-of-japans-typhoon-hagibis/.
- ↑ "Carbon Dioxide Fertilization Greening Earth, Study Finds". 25 April 2016. http://www.nasa.gov/feature/goddard/2016/carbon-dioxide-fertilization-greening-earth.
- ↑ Jump up to: 3.0 3.1 3.2 "Human-induced climate change increased drought severity in Horn of Africa" (in en). 27 April 2023. https://www.worldweatherattribution.org/human-induced-climate-change-increased-drought-severity-in-southern-horn-of-africa/.
- ↑ Jump up to: 4.0 4.1 "Global bioclimatic suitability for the fall armyworm, Spodoptera frugiperda (Lepidoptera: Noctuidae), and potential co-occurrence with major host crops under climate change scenarios" (in en). Climatic Change 161 (4): 555–566. 1 August 2020. doi:10.1007/s10584-020-02722-5. ISSN 1573-1480. Bibcode: 2020ClCh..161..555Z.
- ↑ Jump up to: 5.00 5.01 5.02 5.03 5.04 5.05 5.06 5.07 5.08 5.09 5.10 5.11 5.12 5.13 5.14 5.15 5.16 5.17 5.18 Bezner Kerr, R., T. Hasegawa, R. Lasco, I. Bhatt, D. Deryng, A. Farrell, H. Gurney-Smith, H. Ju, S. Lluch-Cota, F. Meza, G. Nelson, H. Neufeldt, and P. Thornton, 2022: Chapter 5: Food, Fibre, and Other Ecosystem Products. In: Climate Change 2022: Impacts, Adaptation and Vulnerability. Contribution of Working Group II to the Sixth Assessment Report of the Intergovernmental Panel on Climate Change [H.-O. Pörtner, D.C. Roberts, M. Tignor, E.S. Poloczanska, K. Mintenbeck, A. Alegría, M. Craig, S. Langsdorf, S. Löschke, V. Möller, A. Okem, B. Rama (eds.)]. Cambridge University Press, Cambridge, UK and New York, NY, USA, doi:10.1017/9781009325844.007.
- ↑ Jump up to: 6.0 6.1 Gaupp, Franziska; Hall, Jim; Mitchell, Dann; Dadson, Simon (23 May 2019). "Increasing risks of multiple breadbasket failure under 1.5 and 2 °C global warming". Agricultural Systems 175: 34–45. doi:10.1016/j.agsy.2019.05.010. Bibcode: 2019AgSys.175...34G. http://pure.iiasa.ac.at/id/eprint/15923/1/AS_manuscript_revised_2nd.pdf.
- ↑ Jump up to: 7.0 7.1 Kornhuber, Kai; Lesk, Corey; Schleussner, Carl F.; Jägermeyr, Jonas; Pfleiderer, Peter; Horton, Radley M. (4 July 2023). "Risks of synchronized low yields are underestimated in climate and crop model projections". Nature Communications 14: 3528. doi:10.1038/s41467-023-38906-7. PMID 37402712. Bibcode: 2023NatCo..14.3528K.
- ↑ Jump up to: 8.0 8.1 Ainsworth, Elizabeth A.; Long, Stephen P. (2 November 2020). "30 years of free-air carbon dioxide enrichment (FACE): What have we learned about future crop productivity and its potential for adaptation?" (in en). Global Change Biology 27 (1): 27–49. doi:10.1111/gcb.15375. PMID 33135850.
- ↑ Jump up to: 9.0 9.1 "Importance of snow and glacier meltwater for agriculture on the Indo-Gangetic Plain" (in en). Nature Sustainability 2 (7): 594–601. July 2019. doi:10.1038/s41893-019-0305-3. ISSN 2398-9629. Bibcode: 2019NatSu...2..594B. http://www.nature.com/articles/s41893-019-0305-3.
- ↑ Jump up to: 10.0 10.1 10.2 10.3 10.4 10.5 10.6 Zhao, Chuang; Liu, Bing; Piao, Shilong; Wang, Xuhui; Lobell, David B.; Huang, Yao; Huang, Mengtian; Yao, Yitong et al. (15 August 2017). "Temperature increase reduces global yields of major crops in four independent estimates" (in en). Proceedings of the National Academy of Sciences of the United States of America 114 (35): 9326–9331. doi:10.1073/pnas.1701762114. PMID 28811375. Bibcode: 2017PNAS..114.9326Z.
- ↑ Jump up to: 11.0 11.1 11.2 van Dijk, Michiel; Morley, Tom; Rau, Marie Luise; Saghai, Yashar (21 July 2021). "A meta-analysis of projected global food demand and population at risk of hunger for the period 2010–2050". Nature Food 4 (7): 416–426. doi:10.1038/s43016-021-00322-9.
- ↑ Jump up to: 12.0 12.1 ((FAO, IFAD, UNICEF, WFP and WHO)) (2021). The State of Food Security and Nutrition in the World 2021. Transforming food systems for food security, improved nutrition and affordable healthy diets for all, In brief (Report). FAO. doi:10.4060/cb5409en. ISBN 978-92-5-134634-1.
- ↑ Jump up to: 13.0 13.1 13.2 Janssens, Charlotte; Havlík, Petr; Krisztin, Tamás; Baker, Justin; Frank, Stefan; Hasegawa, Tomoko; Leclère, David; Ohrel, Sara et al. (20 July 2020). "Global hunger and climate change adaptation through international trade". Nature Climate Change 10 (9): 829–835. doi:10.1038/s41558-020-0847-4. PMID 33564324. Bibcode: 2020NatCC..10..829J.
- ↑ Jump up to: 14.0 14.1 Hasegawa, Tomoko; Sakurai, Gen; Fujimori, Shinichiro; Takahashi, Kiyoshi; Hijioka, Yasuaki; Masui, Toshihiko (9 August 2021). "Extreme climate events increase risk of global food insecurity and adaptation needs". Nature Food 2 (8): 587–595. doi:10.1038/s43016-021-00335-4. PMID 37118168.
- ↑ Jump up to: 15.0 15.1 Schewe, Jacob; Gosling, Simon N.; Reyer, Christopher; Zhao, Fang; Ciais, Philippe; Elliott, Joshua; Francois, Louis; Huber, Veronika et al. (1 March 2019). "State-of-the-art global models underestimate impacts from climate extremes". Nature Communications 10 (1): 1005. doi:10.1038/s41467-019-08745-6. PMID 30824763. Bibcode: 2019NatCo..10.1005S.
- ↑ Jump up to: 16.0 16.1 16.2 Kummu, Matti; Heino, Matias; Taka, Maija; Varis, Olli; Viviroli, Daniel (21 May 2021). "Climate change risks pushing one-third of global food production outside the safe climatic space". One Earth 4 (5): 720–729. doi:10.1016/j.oneear.2021.04.017. PMID 34056573. Bibcode: 2021OEart...4..720K.
- ↑ Jump up to: 17.0 17.1 Mycoo, M., M. Wairiu, D. Campbell, V. Duvat, Y. Golbuu, S. Maharaj, J. Nalau, P. Nunn, J. Pinnegar, and O. Warrick, 2022: Chapter 3: Mitigation pathways compatible with long-term goals. In Climate Change 2022: Mitigation of Climate Change [ K. Riahi, R.Schaeffer, J.Arango, K. Calvin, C. Guivarch, T. Hasegawa, K. Jiang, E. Kriegler, R. Matthews, G. P. Peters, A. Rao, S. Robertson, A. M. Sebbit, J. Steinberger, M. Tavoni, D. P. van Vuuren]. Cambridge University Press, Cambridge, United Kingdom and New York, NY, USA, pp. 463–464 |doi= 10.1017/9781009157926.005
- ↑ Jump up to: 18.0 18.1 Bradshaw, Corey J. A.; Ehrlich, Paul R.; Beattie, Andrew; Ceballos, Gerardo; Crist, Eileen; Diamond, Joan; Dirzo, Rodolfo; Ehrlich, Anne H. et al. (2021). "Underestimating the Challenges of Avoiding a Ghastly Future". Frontiers in Conservation Science 1. doi:10.3389/fcosc.2020.615419.
- ↑ "Emergent risks and key vulnerabilities". Climate Change 2014: Impacts, Adaptation, and Vulnerability. Cambridge, United Kingdom and New York, NY, USA: Cambridge University Press. 2014. pp. 1039–1099. https://www.ipcc.ch/site/assets/uploads/2018/02/WGIIAR5-Chap19_FINAL.pdf. "Part A: Global and Sectoral Aspects. Contribution of Working Group II to the Fifth Assessment Report of the Intergovernmental Panel on Climate Change"
- ↑ Niles, Meredith T.; Ahuja, Richie; Barker, Todd; Esquivel, Jimena; Gutterman, Sophie; Heller, Martin C.; Mango, Nelson; Portner, Diana et al. (June 2018). "Climate change mitigation beyond agriculture: a review of food system opportunities and implications" (in en). Renewable Agriculture and Food Systems 33 (3): 297–308. doi:10.1017/S1742170518000029. ISSN 1742-1705.
- ↑ Anyiam, P. N.; Adimuko, G. C.; Nwamadi, C. P.; Guibunda, F. A.; Kamale, Y. J. (31 December 2021). "Sustainable Food System Transformation in a Changing Climate" (in en). Nigeria Agricultural Journal 52 (3): 105–115. ISSN 0300-368X. https://www.ajol.info/index.php/naj/article/view/223414.
- ↑ Jump up to: 22.0 22.1 "Severity of drought and heatwave crop losses tripled over the last five decades in Europe" (in en). Environmental Research Letters 16 (6): 065012. 18 March 2021. doi:10.1088/1748-9326/abf004. ISSN 1748-9326. Bibcode: 2021ERL....16f5012B.
Available under CC BY 4.0.
- ↑ Burroughs, Charles H; Montes, Christopher M; Moller, Christopher A; Mitchell, Noah G; Michael, Anne Marie; Peng, Bin; Kimm, Hyungsuk; Pederson, Taylor L et al. (13 March 2023). "Reductions in leaf area index, pod production, seed size, and harvest index drive yield loss to high temperatures in soybean". Journal of Experimental Botany 74 (5): 1629–1641. doi:10.1093/jxb/erac503. PMID 36571807.
- ↑ Jump up to: 24.0 24.1 "Crop and pasture response to climate change". Proceedings of the National Academy of Sciences of the United States of America 104 (50): 19686–19690. December 2007. doi:10.1073/pnas.0701728104. PMID 18077401. Bibcode: 2007PNAS..10419686T.
- ↑ Pradhan, Prajal; Seydewitz, Tobias; Zhou, Bin; Lüdeke, Matthias K. B.; Kropp, Juergen P. (18 July 2022). "Climate extremes are becoming more frequent, co-occurring, and persistent in Europe". Anthropocene Science 1 (2): 264–277. doi:10.1007/s44177-022-00022-4. Bibcode: 2022AnthS...1..264P.
- ↑ "Europe's heat and drought crop losses tripled in 50 years: study" (in en). phys.org. https://phys.org/news/2021-04-europe-drought-crop-losses-tripled.html.
- ↑ "This Summer's Heat Waves Could Be the Strongest Climate Signal Yet". Inside Climate News. 28 July 1018. https://insideclimatenews.org/news/27072018/summer-2018-heat-wave-wildfires-climate-change-evidence-crops-flooding-deaths-records-broken.
- ↑ "Climate Crisis Brings Historic Delay to Planting Season, Pressuring Farmers and Food Prices". Ecowatch. 29 May 2019. https://www.ecowatch.com/climate-crisis-farmers-planting-season-2638398287.html.
- ↑ Hope, Alan (16 July 2021). "Heavy rainfall and flash floods: another catastrophe for farmers?". The Brussels Times (Brussels). https://www.brusselstimes.com/news/business/177734/heavy-rainfall-and-flash-floods-another-catastrophe-for-farmers/.
- ↑ Jump up to: 30.0 30.1 Fu, Jin; Jian, Yiwei; Wang, Xuhui; Li, Laurent; Ciais, Philippe; Zscheischler, Jakob; Wang, Yin; Tang, Yanhong et al. (4 May 2023). "Extreme rainfall reduces one-twelfth of China's rice yield over the last two decades". Nature Food 4 (5): 416–426. doi:10.1038/s43016-023-00753-6. PMID 37142747. https://hal.science/hal-04299080/file/4196_0_art_file_37323_rf8n8j_convrt.pdf.
- ↑ Jump up to: 31.0 31.1 31.2 31.3 Climate Stabilization Targets: Emissions, Concentrations, and Impacts over Decades to Millennia. Washington, D.C.: National Academies Press. 2011-02-11. doi:10.17226/12877. ISBN 978-0-309-15176-4. http://www.nap.edu/catalog/12877.
- ↑ "Corn and Soybean Temperature Response". http://nca2009.globalchange.gov/corn-and-soybean-temperature-response.
- ↑ Jump up to: 33.0 33.1 33.2 "Irrigated agriculture and climate change: The influence of water supply variability and salinity on adaptation". Ecological Economics 77: 149–157. May 2012. doi:10.1016/j.ecolecon.2012.02.021.
- ↑ Jump up to: 34.0 34.1 "Developing climate change impact metrics for agriculture". The Integrated Assessment Journal 8 (1): 165–184. 2008. http://journals.sfu.ca/int_assess/index.php/iaj/article/viewFile/276/240.
- ↑ Jump up to: 35.0 35.1 35.2 35.3 35.4 35.5 35.6 35.7 Changing Planet, Changing Health: How the Climate Crisis Threatens Our Health and what We Can Do about it. University of California Press. 2011. ISBN 978-0-520-26909-5. https://archive.org/details/unset0000unse_c1j4.[page needed]
- ↑ "Predicting the effects of climate change on natural enemies of agricultural pests". Biological Control 52 (3): 296–306. March 2010. doi:10.1016/j.biocontrol.2009.01.022. Bibcode: 2010BiolC..52..296T.
- ↑ "Earliest Blooms Recorded in U.S. Due to Global Warming" (in en). 17 January 2013. https://www.nationalgeographic.com/news/2013/1/130116-spring-earlier-global-warming-plants-trees-blooming-science/.
- ↑ Jump up to: 38.0 38.1 "Climate Change, Prairie Agriculture and Prairie Economy: The new normal". Canadian Journal of Agricultural Economics 59 (1): 19–44. March 2011. doi:10.1111/j.1744-7976.2010.01211.x. Bibcode: 2011CaJAE..59...19K.
- ↑ Jump up to: 39.0 39.1 Climate Change Impacts and Adaptation: A Canadian Perspective (Report). Natural Resources Canada. 2004. ISBN 0-662-33123-0. http://cfs.nrcan.gc.ca/pubwarehouse/pdfs/27428.pdf.[page needed]
- ↑ "The benefits of recent warming for maize production in high latitude China". Climatic Change 122 (1–2): 341–349. 2013. doi:10.1007/s10584-013-1009-8.
- ↑ Jump up to: 41.0 41.1 Ficklin, Darren L.; Null, Sarah E.; Abatzoglou, John T.; Novick, Kimberly A.; Myers, Daniel T. (9 March 2022). "Hydrological Intensification Will Increase the Complexity of Water Resource Management". Earth's Future 10 (3): e2021EF002487. doi:10.1029/2021EF002487. Bibcode: 2022EaFut..1002487F. https://digitalcommons.usu.edu/wats_facpub/1124.
- ↑ Brown, Oliver L. I. (August 1951). "The Clausius-Clapeyron equation". Journal of Chemical Education 28 (8): 428. doi:10.1021/ed028p428. Bibcode: 1951JChEd..28..428B.
- ↑ Trenberth, Kevin E.; Smith, Lesley; Qian, Taotao; Dai, Aiguo; Fasullo, John (2007-08-01). "Estimates of the Global Water Budget and Its Annual Cycle Using Observational and Model Data". Journal of Hydrometeorology 8 (4): 758–769. doi:10.1175/jhm600.1. Bibcode: 2007JHyMe...8..758T.
- ↑ Jump up to: 44.0 44.1 Schuur, Edward A.G.; Abbott, Benjamin W.; Commane, Roisin; Ernakovich, Jessica; Euskirchen, Eugenie; Hugelius, Gustaf; Grosse, Guido; Jones, Miriam et al. (2022). "Permafrost and Climate Change: Carbon Cycle Feedbacks From the Warming Arctic". Annual Review of Environment and Resources 47: 343–371. doi:10.1146/annurev-environ-012220-011847. ""Medium-range estimates of Arctic carbon emissions could result from moderate climate emission mitigation policies that keep global warming below 3°C (e.g., RCP4.5). This global warming level most closely matches country emissions reduction pledges made for the Paris Climate Agreement..."".
- ↑ Jump up to: 45.0 45.1 Phiddian, Ellen (5 April 2022). "Explainer: IPCC Scenarios". https://cosmosmagazine.com/earth/climate/explainer-ipcc-scenarios/. ""The IPCC doesn’t make projections about which of these scenarios is more likely, but other researchers and modellers can. The Australian Academy of Science, for instance, released a report last year stating that our current emissions trajectory had us headed for a 3°C warmer world, roughly in line with the middle scenario. Climate Action Tracker predicts 2.5 to 2.9°C of warming based on current policies and action, with pledges and government agreements taking this to 2.1°C."
- ↑ "Drought under global warming: A review". Wiley Interdisciplinary Reviews: Climate Change 2: 45–65. 2011. doi:10.1002/wcc.81. Bibcode: 2011AGUFM.H42G..01D. https://zenodo.org/record/1229380.
- ↑ "Water scarcity predicted to worsen in more than 80% of croplands globally this century" (in en). American Geophysical Union. https://phys.org/news/2022-05-scarcity-worsen-croplands-globally-century.html.
- ↑ Liu, Xingcai; Liu, Wenfeng; Tang, Qiuhong; Liu, Bo; Wada, Yoshihide; Yang, Hong (April 2022). "Global Agricultural Water Scarcity Assessment Incorporating Blue and Green Water Availability Under Future Climate Change". Earth's Future 10 (4). doi:10.1029/2021EF002567. Bibcode: 2022EaFut..1002567L. https://www.dora.lib4ri.ch/eawag/islandora/object/eawag%3A24825.
- ↑ "Drought modeling – A review". Journal of Hydrology 403 (1–2): 157–175. 2011. doi:10.1016/j.jhydrol.2011.03.049. Bibcode: 2011JHyd..403..157M.
- ↑ "Measuring economic impacts of drought: A review and discussion". Disaster Prevention and Management 20 (4): 434–446. 2011. doi:10.1108/09653561111161752. Bibcode: 2011DisPM..20..434D. http://digitalcommons.unl.edu/natrespapers/196.
- ↑ Jump up to: 51.0 51.1 "Climate Change, Agriculture, and Poverty". Applied Economic Perspectives and Policy 32 (3): 355–385. June 2010. doi:10.1093/aepp/ppq016. http://ageconsearch.umn.edu/record/91437/files/Hertel_et_al._IATRC_Summer_2010.pdf.
- ↑ Jump up to: 52.0 52.1 Bolch, Tobias; Shea, Joseph M.; Liu, Shiyin; Azam, Farooq M.; Gao, Yang; Gruber, Stephan; Immerzeel, Walter W.; Kulkarni, Anil et al. (5 January 2019). "Status and Change of the Cryosphere in the Extended Hindu Kush Himalaya Region". The Hindu Kush Himalaya Assessment. pp. 209–255. doi:10.1007/978-3-319-92288-1_7. ISBN 978-3-319-92287-4. https://link.springer.com/chapter/10.1007/978-3-319-92288-1_3.
- ↑ "Glaciers Are Melting Faster Than Expected, UN Reports". ScienceDaily. https://www.sciencedaily.com/releases/2008/03/080317154235.htm.
- ↑ "Impact of a global temperature rise of 1.5 degrees Celsius on Asia's glaciers". Nature 549 (7671): 257–260. September 2017. doi:10.1038/nature23878. PMID 28905897. Bibcode: 2017Natur.549..257K.
- ↑ "Big melt threatens millions, says UN". People & the Planet. http://www.peopleandplanet.net/pdoc.php?id=3024.
- ↑ "Ganges, Indus may not survive: climatologists". Rediff.com India Limited. 24 July 2007. http://www.rediff.com/news/2007/jul/24indus.htm.
- ↑ "Himalaya glaciers melt unnoticed". 10 November 2004. http://news.bbc.co.uk/2/hi/science/nature/3998967.stm.
- ↑ Krishnan, Raghavan; Shrestha, Arun Bhakta; Ren, Guoyu; Rajbhandari, Rupak; Saeed, Sajjad; Sanjay, Jayanarayanan; Syed, Md. Abu.; Vellore, Ramesh et al. (5 January 2019). "Unravelling Climate Change in the Hindu Kush Himalaya: Rapid Warming in the Mountains and Increasing Extremes". The Hindu Kush Himalaya Assessment. pp. 57–97. doi:10.1007/978-3-319-92288-1_3. ISBN 978-3-319-92287-4. https://link.springer.com/chapter/10.1007/978-3-319-92288-1_3.
- ↑ Scott, Christopher A.; Zhang, Fan; Mukherji, Aditi; Immerzeel, Walter; Mustafa, Daanish; Bharati, Luna (5 January 2019). "Water in the Hindu Kush Himalaya". The Hindu Kush Himalaya Assessment. pp. 257–299. doi:10.1007/978-3-319-92288-1_8. ISBN 978-3-319-92287-4. https://link.springer.com/chapter/10.1007/978-3-319-92288-1_8.
- ↑ "Rising Carbon Dioxide Levels Will Help and Hurt Crops" (in en). NASA. 3 May 2016. https://www.nasa.gov/feature/goddard/2016/nasa-study-rising-carbon-dioxide-levels-will-help-and-hurt-crops.
- ↑ Jump up to: 61.0 61.1 "Global Warming and Agriculture" (in en-US). March 2008. https://www.imf.org/external/pubs/ft/fandd/2008/03/cline.htm.
- ↑ Jump up to: 62.0 62.1 62.2 62.3 62.4 "Hidden shift of the ionome of plants exposed to elevated CO2 depletes minerals at the base of human nutrition" (in En). eLife 3 (9): e02245. May 2014. doi:10.7554/eLife.02245. PMID 24867639.
- ↑ Jump up to: 63.0 63.1 63.2 Riahi, Keywan; van Vuuren, Detlef P.; Kriegler, Elmar; Edmonds, Jae; O'Neill, Brian C.; Fujimori, Shinichiro; Bauer, Nico; Calvin, Katherine et al. (1 February 2017). "The Shared Socioeconomic Pathways and their energy, land use, and greenhouse gas emissions implications: An overview". Global Environmental Change 42 (9): 153–168. doi:10.1016/j.gloenvcha.2016.05.009.
- ↑ Jump up to: 64.0 64.1 64.2 64.3 "Chapter 5: Food Security". Climate Change and Land: an IPCC special report on climate change, desertification, land degradation, sustainable land management, food security, and greenhouse gas fluxes in terrestrial ecosystems. 2019. https://www.ipcc.ch/site/assets/uploads/sites/4/2021/02/08_Chapter-5_3.pdf.
- ↑ "Worries grow that climate change will quietly steal nutrients from major food crops". Science News. 13 December 2017. https://www.sciencenews.org/article/nutrition-climate-change-top-science-stories-2017-yir.
- ↑ "Impact of anthropogenic CO2 emissions on global human nutrition" (in En). Nature Climate Change 8 (9): 834–839. 27 August 2018. doi:10.1038/s41558-018-0253-3. ISSN 1758-678X. Bibcode: 2018NatCC...8..834S.
- ↑ "Climate change will make hundreds of millions more people nutrient deficient" (in en). 27 August 2018. https://www.theguardian.com/science/2018/aug/27/climate-change-will-make-hundreds-of-millions-more-people-nutrient-deficient.
- ↑ "Rising atmospheric CO2 and human nutrition: toward globally imbalanced plant stoichiometry?". Trends in Ecology & Evolution 17 (10): 457–461. 2002. doi:10.1016/S0169-5347(02)02587-9.
- ↑ "Carbon dioxide (CO2) levels this century will alter the protein, micronutrients, and vitamin content of rice grains with potential health consequences for the poorest rice-dependent countries". Science Advances 4 (5): eaaq1012. May 2018. doi:10.1126/sciadv.aaq1012. PMID 29806023. Bibcode: 2018SciA....4.1012Z.
- ↑ "As CO2 increases, rice loses B vitamins and other nutrients". 23 May 2018. https://www.sciencenews.org/article/carbon-dioxide-increases-rice-loses-b-vitamins-nutrients.
- ↑ "Effects of Elevated Atmospheric Carbon Dioxide on Insect-Plant Interactions". Conservation Biology 13 (4): 700–712. 1999. doi:10.1046/j.1523-1739.1999.98267.x. Bibcode: 1999ConBi..13..700C.
- ↑ "Effects of elevated CO
2 on the protein concentration of food crops: a meta-analysis". Global Change Biology 14 (3): 565–575. 2008. doi:10.1111/j.1365-2486.2007.01511.x. Bibcode: 2008GCBio..14..565T. - ↑ "Increasing CO
2 threatens human nutrition". Nature 510 (7503): 139–42. June 2014. doi:10.1038/nature13179. PMID 24805231. Bibcode: 2014Natur.510..139M. - ↑ Jump up to: 74.0 74.1 Jasechko, Scott J.; Perrone, Debra; Seybold, Hansjörg; Fan, Ying; Kirchner, James W. (26 June 2020). "Groundwater level observations in 250,000 coastal US wells reveal scope of potential seawater intrusion". Nature Communications 11 (1): 3229. doi:10.1038/s41467-020-17038-2. PMID 32591535. Bibcode: 2020NatCo..11.3229J.
- ↑ "Towards constraining the magnitude of global agricultural sediment and soil organic carbon fluxes" (in en). Earth Surface Processes and Landforms 37 (6): 642–655. 1 May 2012. doi:10.1002/esp.3198. ISSN 1096-9837. Bibcode: 2012ESPL...37..642D.
- ↑ "Soil Microbiomes Under Climate Change and Implications for Carbon Cycling". Annual Review of Environment and Resources 45: 29–59. 2020. doi:10.1146/annurev-environ-012320-082720.
- ↑ "Chapter 9: Ocean, Cryosphere, and Sea Level Change". Climate Change 2021: The Physical Science Basis. Contribution of Working Group I to the Sixth Assessment Report of the Intergovernmental Panel on Climate. Cambridge University Press. 2021. https://www.ipcc.ch/report/ar6/wg1/downloads/report/IPCC_AR6_WGI_Chapter_09.pdf.
- ↑ "Coping With Climate Change". Rice Today (IRRI): 10–15. July–September 2007. http://www.irri.org/publications/today/pdfs/6-3/10-15.pdf. Retrieved 7 October 2009.
- ↑ German Research Indicates Warming in Siberia, Global Warming Today, Global Warming Today
- ↑ Federal Service for Hydrometeorology and Environmental Monitoring 5Roshydromet), Strategic Forecast of Climate Change in the Russian Federation 2010–2015 and Its Impact on Sectors of the Russian Economy (Moscow 2005)
- ↑ "The Danger of Climate Change for Russia – Expected Losses and Recommendations". Russian Analytical Digest (23): 2–4. 2007. https://ethz.ch/content/dam/ethz/special-interest/gess/cis/center-for-securities-studies/pdfs/RAD-23-2-4.pdf.
- ↑ "Global warming 'will hurt Russia'". New Scientist. 3 October 2003. https://www.newscientist.com/article/dn4232-global-warming-will-hurt-russia/.
- ↑ Parry, ML, ed (2007). "Chapter 15: Polar regions (Arctic and Antarctic): Executive summary". Climate Change 2007: Impacts, Adaptation and Vulnerability. Contribution of Working Group II to the Fourth Assessment Report of the Intergovernmental Panel on Climate Change. Cambridge University Press. http://www.ipcc.ch/publications_and_data/ar4/wg2/en/ch15s15-es.html. Retrieved 6 March 2022.
- ↑ Jump up to: 84.0 84.1 "Global Warming Could Trigger Insect Population Boom". Live Science. http://www.livescience.com/4296-global-warming-trigger-insect-population-boom.html.
- ↑ Jump up to: 85.0 85.1 85.2 85.3 85.4 "Climate change and diseases of food crops". Plant Pathology (British Society for Plant Pathology (Wiley-Blackwell)) 60 (1): 113–121. 10 January 2011. doi:10.1111/j.1365-3059.2010.02414.x. ISSN 0032-0862.
- ↑ Jump up to: 86.0 86.1 86.2 "Challenges for weed management in African rice systems in a changing climate". The Journal of Agricultural Science 149 (4): 427–435. August 2011. doi:10.1017/S0021859611000207. http://ecite.utas.edu.au/69596.
- ↑ Warren, R.; Price, J.; Graham, E.; Forstenhaeusler, N.; VanDerWal, J. (18 May 2018). "The projected effect on insects, vertebrates, and plants of limiting global warming to 1.5°C rather than 2°C" (in en). Science 360 (6390): 791–795. doi:10.1126/science.aar3646. PMID 29773751.
- ↑ "ECPA". https://www.ecpa.eu/.
- ↑ Soroye, Peter; Newbold, Tim; Kerr, Jeremy (7 Feb 2020). "Climate change contributes to widespread declines among bumble bees across continents". Science 367 (6478): 685–688. doi:10.1126/science.aax8591. PMID 32029628. Bibcode: 2020Sci...367..685S.
- ↑ "Bumblebees are disappearing at rates 'consistent with mass extinction'". https://www.usatoday.com/story/news/nation/2020/02/06/bumblebees-decline-due-climate-change/4679240002/.
- ↑ "Climate Change Impact: Insects". eLS (Norwegian Institute for Nature Research). November 2010. doi:10.1002/9780470015902.a0022555. ISBN 9780470016176. http://www.els.net/WileyCDA/ElsArticle/refId-a0022555.html.
- ↑ Jump up to: 92.0 92.1 92.2 92.3 "Climate Change and Its Repercussions for the Potato Supply Chain". Potato Research 51 (3–4): 223–237. October 2008. doi:10.1007/s11540-008-9107-0.
- ↑ "What Can Plasticity Contribute to Insect Responses to Climate Change?". Annual Review of Entomology (Annual Reviews) 61 (1): 433–451. 2016-03-11. doi:10.1146/annurev-ento-010715-023859. PMID 26667379.
- ↑ "Locust swarms and climate change" (in en). 6 February 2020. http://www.unenvironment.org/news-and-stories/story/locust-swarms-and-climate-change.
- ↑ "Real-time evaluation of FAO's response to desert locust upsurge ". https://www.fao.org/evaluation/evaluation-digest/desert-locusts/en/.
- ↑ Jump up to: 96.0 96.1 "Early Summer Weed Control". http://extension.unl.edu/statewide/sw3/6.17.15%20Early%20Summer%20Weed%20Control.pdf.
- ↑ Jump up to: 97.0 97.1 97.2 "Climate change, plant diseases and food security: an overview". Plant Pathology 60 (1): 2–14. 10 January 2011. doi:10.1111/j.1365-3059.2010.02411.x.
- ↑ "Why is Dickeya spp. (syn. Erwinia chrysanthemi) taking over? The ecology of a blackleg pathogen". http://www.knpv.org/db/upload/documents/Pests%20and%20climate%20change/van_der_Wolf_KNPV3_dec_2008.pdf.
- ↑ Jump up to: 99.0 99.1 "Climate change and plant disease management". Annual Review of Phytopathology 37: 399–426. September 1999. doi:10.1146/annurev.phyto.37.1.399. PMID 11701829.
- ↑ "Implications for a warmer, wetter world on the late blight pathogen: How CIP efforts can reduce risk for low-input potato farmers". CIP. http://www.icrisat.org/journal/SpecialProject/sp4.pdf.
- ↑ "Climate change and plant disease management". Annual Review of Phytopathology 37 (1): 399–426. September 1999. doi:10.1146/annurev.phyto.37.1.399. PMID 11701829.
- ↑ [chapter-https://www.taylorfrancis.com/chapters/edit/10.4324/9780203506608-14/plant-disease-climate-change-chakraborty-pangga "Plant disease and climate change"]. Plant Microbiology. Taylor & Francis. 2004. doi:10.4324/9780203506608. ISBN 978-0-203-50660-8. chapter-https://www.taylorfrancis.com/chapters/edit/10.4324/9780203506608-14/plant-disease-climate-change-chakraborty-pangga. Retrieved 2022-04-01.
- ↑ "Potato Research Priorities in Asia and the Pacific Region". FAO. http://www.fao.org/docrep/010/i0200e/I0200E08.htm.
- ↑ Jump up to: 104.0 104.1 104.2 104.3 "Climate change has likely already affected global food production". PLOS ONE 14 (5): e0217148. 2019. doi:10.1371/journal.pone.0217148. PMID 31150427. Bibcode: 2019PLoSO..1417148R.
- ↑ IPCC, 2019: Summary for Policymakers. In: Climate Change and Land: an IPCC special report on climate change, desertification, land degradation, sustainable land management, food security, and greenhouse gas fluxes in terrestrial ecosystems [P.R. Shukla, J. Skea, E. Calvo Buendia, V. Masson-Delmotte, H.- O. Pörtner, D. C. Roberts, P. Zhai, R. Slade, S. Connors, R. van Diemen, M. Ferrat, E. Haughey, S. Luz, S. Neogi, M. Pathak, J. Petzold, J. Portugal Pereira, P. Vyas, E. Huntley, K. Kissick, M. Belkacemi, J. Malley, (eds.)]. In press.
- ↑ "Climate trends and global crop production since 1980". Science 333 (6042): 616–620. July 2011. doi:10.1126/science.1204531. PMID 21551030. Bibcode: 2011Sci...333..616L.
- ↑ Gollin, Douglas; Hansen, Casper Worm; Wingender, Asger Mose (2021). "Two Blades of Grass: The Impact of the Green Revolution". Journal of Political Economy 129 (8): 2344–2384. doi:10.1086/714444. ISSN 0022-3808. https://www.journals.uchicago.edu/doi/10.1086/714444.
- ↑ Malik, Arunima; Li, Mengyu; Lenzen, Manfred; Fry, Jacob; Liyanapathirana, Navoda; Beyer, Kathleen; Boylan, Sinead; Lee, Amanda et al. (18 August 2022). "Impacts of climate change and extreme weather on food supply chains cascade across sectors and regions in Australia". Nature Food 3 (8): 631–643. doi:10.1038/s43016-022-00570-3. PMID 37118599.
- ↑ Conway, Gordon (1998). The doubly green revolution: food for all in the twenty-first century. Ithaca, NY: Comstock Pub. ISBN 978-0-8014-8610-4. https://archive.org/details/doublygreenrevol00conw. Ch. 4
- ↑ Jump up to: 110.0 110.1 Nelson, Gerald C.; Valin, Hugo; Sands, Ronald D.; Havlík, Petr; Ahammad, Helal; Deryng, Delphine; Elliott, Joshua; Fujimori, Shinichiro et al. (16 December 2013). "Climate change effects on agriculture: Economic responses to biophysical shocks". Proceedings of the National Academy of Sciences of the United States of America 111 (9): 3274–3279. doi:10.1073/pnas.1222465110. PMID 24344285.
- ↑ "19.3.1 Introduction to Table 19.1". Chapter 19: Assessing Key Vulnerabilities and the Risk from Climate Change. Climate change 2007: impacts, adaptation and vulnerability: contribution of Working Group II to the fourth assessment report of the Intergovernmental Panel on Climate Change. Cambridge University Press (CUP): Cambridge, UK: Print version: CUP. This version: IPCC website. 2007. ISBN 978-0-521-88010-7. http://www.ipcc.ch/publications_and_data/ar4/wg2/en/ch19s19-3-1.html. Retrieved 4 May 2011.
- ↑ Jump up to: 112.0 112.1 112.2 IPCC (2007). "Summary for Policymakers: C. Current knowledge about future impacts". Climate Change 2007: Impacts, Adaptation and Vulnerability. Contribution of Working Group II to the Fourth Assessment Report of the Intergovernmental Panel on Climate Change. Cambridge University Press. http://www.ipcc.ch/publications_and_data/ar4/wg2/en/spmsspm-c.html. Retrieved 6 March 2022.
- ↑ "A meta-analysis of crop yield under climate change and adaptation" (in en). Nature Climate Change 4 (4): 287–291. 2014. doi:10.1038/nclimate2153. ISSN 1758-678X. Bibcode: 2014NatCC...4..287C. http://eprints.whiterose.ac.uk/78340/13/Challinor-etal-AR5-RevisionsFinal_with_coversheet.pdf.
- ↑ McKie, Robin (16 July 2017). "Maize, rice, wheat: alarm at rising climate risk to vital crops". The Observer. https://www.theguardian.com/environment/2017/jul/15/climate-change-food-famine-study.
- ↑ Wing, Ian Sue; De Cian, Enrica; Mistry, Malcolm N. (5 June 2021). "Global vulnerability of crop yields to climate change". Journal of Environmental Economics and Management 109. doi:10.1016/j.jeem.2021.102462.
- ↑ Jump up to: 116.0 116.1 116.2 116.3 116.4 Jägermeyr, Jonas; Müller, Christoph; Ruane, Alex C.; Elliott, Joshua; Balkovic, Juraj; Castillo, Oscar; Faye, Babacar; Foster, Ian et al. (1 November 2021). "Climate impacts on global agriculture emerge earlier in new generation of climate and crop models". Nature Food 2 (11): 873–885. doi:10.1038/s43016-021-00400-y. PMID 37117503. https://research.utwente.nl/en/publications/917b35a5-919f-4d20-930b-a57dd3d1ca38.
- ↑ Hausfather, Zeke; Peters, Glen (29 January 2020). "Emissions – the 'business as usual' story is misleading". Nature 577 (7792): 618–20. doi:10.1038/d41586-020-00177-3. PMID 31996825. Bibcode: 2020Natur.577..618H.
- ↑ "Climate Change Impact Assessment and Adaptation Strategies for Rainfed Wheat in Contrasting Climatic Regions of Iran". Frontiers in Agronomy 3: 806146. 2021-12-20. doi:10.3389/fagro.2021.806146. ISSN 2673-3218.
- ↑ Agnolucci, Paolo; Rapti, Chrysanthi; Alexander, Peter; De Lipsis, Vincenzo; Holland, Robert A.; Eigenbrod, Felix; Ekins, Paul (September 2020). "Impacts of rising temperatures and farm management practices on global yields of 18 crops" (in en). Nature Food 1 (9): 562–571. doi:10.1038/s43016-020-00148-x. ISSN 2662-1355. PMID 37128016. https://www.nature.com/articles/s43016-020-00148-x.
- ↑ Proctor, Jonathan; Rigden, Angela; Chan, Duo; Huybers, Peter (19 September 2022). "More accurate specification of water supply shows its importance for global crop production". Nature Food 3 (9): 753–763. doi:10.1038/s43016-022-00592-x. PMID 37118152.
- ↑ "Reduction in nutritional quality and growing area suitability of common bean under climate change induced drought stress in Africa". Scientific Reports 8 (1): 16187. November 2018. doi:10.1038/s41598-018-33952-4. PMID 30385766. Bibcode: 2018NatSR...816187H.
- ↑ Gervais, Taylor; Creelman, Alexa; Li, Xiu-Qing; Bizimungu, Benoit; De Koeyer, David; Dahal, Keshav (12 August 2021). "Potato Response to Drought Stress: Physiological and Growth Basis". Frontiers in Plant Science 12. doi:10.3389/fpls.2021.698060. PMID 34456939.
- ↑ "Climate change and potatoes: The risks, impacts and opportunities for UK potato production". Cranfield Water Science Institute. http://www.potato.org.uk/sites/default/files/%5Bcurrent-page%3Aarg%3A%3F%5D/CC%20impacts%20potatoes_Final_20Sept2011.pdf.
- ↑ Jump up to: 124.0 124.1 Hasegawa, Tomoko; Fujimori, Shinichiro; Takahashi, Kiyoshi; Yokohata, Tokuta; Masui, Toshihiko (29 January 2016). "Economic implications of climate change impacts on human health through undernourishment". Climatic Change 136 (2): 189–202. doi:10.1007/s10584-016-1606-4. Bibcode: 2016ClCh..136..189H.
- ↑ Springmann, Marco; Mason-D'Croz, Daniel; Robinson, Sherman; Garnett, Tara; Godfray, Charles J; Gollin, Douglas; Rayner, Mike; Ballon, Paola et al. (2 March 2016). "Global and regional health effects of future food production under climate change: a modelling study". The Lancet 387 (10031): 1937–1946. doi:10.1016/S0140-6736(15)01156-3. PMID 26947322. https://ora.ox.ac.uk/objects/uuid:6cac6d6f-d5a2-4f45-9dbf-8ebf22ab7a9a.
- ↑ "Climate Change and Global Food Systems: Potential Impacts on Food Security and Undernutrition". Annual Review of Public Health 38 (1): 259–277. March 2017. doi:10.1146/annurev-publhealth-031816-044356. PMID 28125383.
- ↑ "Box TS.2. Communication of uncertainty in the Working Group II Fourth Assessment". Technical summary. Climate change 2007: impacts, adaptation and vulnerability: contribution of Working Group II to the fourth assessment report of the Intergovernmental Panel on Climate Change. Cambridge University Press (CUP): Cambridge, UK: Print version: CUP. This version: IPCC website. 2007. ISBN 978-0-521-88010-7. http://www.ipcc.ch/publications_and_data/ar4/wg2/en/tssts-1.html. Retrieved 4 May 2011.
- ↑ "Executive summary". Chapter 5: Food, Fibre, and Forest Products. Climate change 2007: impacts, adaptation and vulnerability: contribution of Working Group II to the fourth assessment report of the Intergovernmental Panel on Climate Change. Cambridge University Press. 2007. ISBN 978-0-521-88010-7. http://www.ipcc.ch/publications_and_data/ar4/wg2/en/ch5s5-es.html. Retrieved 9 January 2013.
- ↑ "5.6.5 Food security and vulnerability". Chapter 5: Food, Fibre, and Forest Products. Climate change 2007: impacts, adaptation and vulnerability: contribution of Working Group II to the fourth assessment report of the Intergovernmental Panel on Climate Change. Cambridge University Press. 2007. ISBN 978-0-521-88010-7. http://www.ipcc.ch/publications_and_data/ar4/wg2/en/ch5s5-6-5.html. Retrieved 25 June 2011.
- ↑ Heinicke, Stefanie; Frieler, Katja; Jägermeyr, Jonas; Mengel, Matthias (18 March 2022). "Global gridded crop models underestimate yield responses to droughts and heatwaves". Environmental Research Letters 17 (4): 044026. doi:10.1088/1748-9326/ac592e. Bibcode: 2022ERL....17d4026H.
- ↑ Jump up to: 131.0 131.1 Lyon, Christopher; Saupe, Erin E.; Smith, Christopher J.; Hill, Daniel J.; Beckerman, Andrew P.; Stringer, Lindsay C.; Marchant, Robert; McKay, James et al. (2021). "Climate change research and action must look beyond 2100" (in en). Global Change Biology 28 (2): 349–361. doi:10.1111/gcb.15871. ISSN 1365-2486. PMID 34558764.
- ↑ Jump up to: 132.0 132.1 132.2 d'Amour, Christopher Bren; Wenz, Leonie; Kalkuh, Matthias; Steckel, Jan Christoph; Creutzig, Felix (29 February 2016). "Teleconnected food supply shocks". Environmental Research Letters 11 (3): 035007. doi:10.1088/1748-9326/11/3/035007. Bibcode: 2016ERL....11c5007B.
- ↑ Caparas, Monica; Zobel, Zachary; Castanho, Andrea D A; Schwalm, Christopher R (21 September 2021). "Increasing risks of crop failure and water scarcity in global breadbaskets by 2030". Environmental Research Letters 16 (10). doi:10.1088/1748-9326/ac22c1. Bibcode: 2021ERL....16j4013C.
- ↑ Mehrabi, Zia; Ramankutty, Navin (15 April 2019). "Synchronized failure of global crop production". Nature Ecology & Evolution 3 (5): 780–786. doi:10.1038/s41559-019-0862-x. PMID 30988491. Bibcode: 2019NatEE...3..780M.
- ↑ Hasegawa, Toshihiro; Wakatsuki, Hitomi; Nelson, Gerald C. (20 October 2022). "Evidence for and projection of multi-breadbasket failure caused by climate change". Current Opinion in Environmental Sustainability 58. doi:10.1016/j.cosust.2022.101217. Bibcode: 2022COES...5801217H.
- ↑ Kornhuber, Kai; Coumou, Dim; Vogel, Elisabeth; Lesk, Corey; Donges, Jonathan F.; Lehmann, Jascha; Horton, Radley M. (9 December 2019). "Amplified Rossby waves enhance risk of concurrent heatwaves in major breadbasket regions". Nature Climate Change 10 (1): 48–53. doi:10.1038/s41558-019-0637-z. Bibcode: 2019NatCC..10...48K. https://research.vu.nl/en/publications/08ff0c33-fdd1-41e7-8a08-20f528a5a999.
- ↑ Jump up to: 137.0 137.1 Kang, Suchul; Eltahir, Elfatih A. B. (31 July 2018). "North China Plain threatened by deadly heatwaves due to climate change and irrigation". Nature Communications 9. doi:10.1038/s41467-023-38906-7. PMID 37402712. Bibcode: 2023NatCo..14.3528K.
- ↑ "Are food insecure smallholder households making changes in their farming practices? Evidence from East Africa". Food Security 4 (3): 381–397. 2012. doi:10.1007/s12571-012-0194-z.
- ↑ "When Hard Jobs Turn Hazardous" (in en-US). The New York Times. 4 September 2021. ISSN 0362-4331. https://www.nytimes.com/2021/09/04/business/economy/when-hard-jobs-turn-hazardous.html.
- ↑ Jump up to: 140.0 140.1 140.2 140.3 "The role for scientists in tackling food insecurity and climate change". Agriculture & Food Security 1 (10): 10. 2012. doi:10.1186/2048-7010-1-10. Bibcode: 2012AgFS....1...10B.
- ↑ Mendelsohn, Robert (1 April 2014). "The Impact of Climate Change on Agriculture in Asia" (in en). Journal of Integrative Agriculture 13 (4): 660–665. doi:10.1016/S2095-3119(13)60701-7. ISSN 2095-3119.
- ↑ "Direct and indirect impacts of climate change on wheat yield in the Indo-Gangetic plain in India" (in en). Journal of Agriculture and Food Research 4: 100132. 2021. doi:10.1016/j.jafr.2021.100132.
- ↑ "Impact of Climate Change on Livestock in Bangladesh: A Review of What We Know and What We Need to Know". American Journal of Agricultural Science Engineering and Technology 3 (2): 18–25. 2016. doi:10.54536/ajaset.v3i2.40. http://ajaset.e-palli.com/wp-content/uploads/2013/12/IMPACT-OF-CLIMATE-CHANGE-ON-LIVESTOCK-IN-BANGLADESH-A-REVIEW-OF-WHAT-WE-KNOW-AND-WHAT-WE-NEED-TO-KNOW.pdf. Retrieved 6 March 2022.
- ↑ Jump up to: 144.0 144.1 "Chapter 11: Australia and New Zealand: Executive summary". Climate Change 2007: Impacts, Adaptation and Vulnerability. Contribution of Working Group II to the Fourth Assessment Report of the Intergovernmental Panel on Climate Change. Cambridge University Press. 2007. https://www.ipcc.ch/publications_and_data/ar4/wg2/en/ch11s11-es.html.
- ↑ "Climate change threatens future of farming in Europe — European Environment Agency". https://www.eea.europa.eu/highlights/climate-change-threatens-future-of.
- ↑ World Bank. 2021. Ukraine: Building Climate Resilience in Agriculture and Forestry. 151p.https://documents1.worldbank.org/curated/en/893671643276478711/pdf/Ukraine-Building-Climate-Resilience-in-Agriculture-and-Forestry.pdf
- ↑ Jump up to: 147.0 147.1 147.2 147.3 147.4 "The potential impacts of climate change on maize production in Africa and Latin America in 2055". Global Environmental Change 13 (1): 51–59. April 2003. doi:10.1016/S0959-3780(02)00090-0.
- ↑ Jump up to: 148.0 148.1 148.2 148.3 148.4 148.5 148.6 "Vulnerability of the agricultural sector of Latin America to climate change". Climate Research 9: 1–7. 1997. doi:10.3354/cr009001. Bibcode: 1997ClRes...9....1B. https://www.int-res.com/articles/cr/9/c009p001.pdf.
- ↑ "Climate Change, Agriculture, and Developing Countries: Does Adaptation Matter?". The World Bank Research Observer 14 (2): 277–293. 1 August 1999. doi:10.1093/wbro/14.2.277.
- ↑ "What's Really Driving Immigrants North from Central America". The State of Things. 22 February 2019. https://www.wunc.org/show/the-state-of-things/2019-02-22/whats-really-driving-immigrants-north-from-central-america.
- ↑ "Living Smallholder Vulnerability: The Everyday Experience of Climate Change in Calakmul, Mexico". Journal of Latin American Geography (University of Texas Press) 19 (2): 110–142. March 2020. doi:10.1353/lag.2020.0028.
- ↑ "Billion-Dollar Weather and Climate Disasters: Table of Events - National Centers for Environmental Information (NCEI)". http://www.ncdc.noaa.gov/billions/events.
- ↑ "Vulnerability of riparian ecosystems to elevated CO2 and climate change in arid and semiarid western North America". Global Change Biology 18 (3): 821–842. 2012. doi:10.1111/j.1365-2486.2011.02588.x. Bibcode: 2012GCBio..18..821P. http://www.fort.usgs.gov/Products/Publications/23228/23228.pdf.
- ↑ "Climate change impacts on Canadian yields of spring wheat, canola and maize for global warming levels of 1.5 °C, 2.0 °C, 2.5 °C and 3.0 °C". Environmental Research Letters 14 (7): 074005. 2019-07-01. doi:10.1088/1748-9326/ab17fb. ISSN 1748-9326. Bibcode: 2019ERL....14g4005Q.
- ↑ Climate Change: Impact on Agriculture and Costs of Adaptation (Report). Washington, DC: International Food Policy Research Institute. October 2009. http://ebrary.ifpri.org/utils/getfile/collection/p15738coll2/id/16557/filename/16558.pdf. Retrieved 12 August 2016.
- ↑ "Climate Change, Agriculture, and Poverty". Applied Economic Perspectives and Policy 32 (3): 355–385. June 2010. doi:10.1093/aepp/ppq016. http://ageconsearch.umn.edu/record/91437/files/Hertel_et_al._IATRC_Summer_2010.pdf.
- ↑ Jump up to: 157.0 157.1 "Agricultural Adaptation to Climate Change". http://agadapt.ucdavis.edu/pestsdiseases/.
- ↑ "Climate change and agriculture in South Asia: adaptation options in smallholder production systems" (in en). Environment, Development and Sustainability 22 (6): 5045–5075. 2020. doi:10.1007/s10668-019-00414-4. ISSN 1387-585X. Bibcode: 2020EDSus..22.5045A.
- ↑ Vulnerability to Climate Change: Adaptation Strategies and layers of Resilience , ICRISAT, Policy Brief No. 23, February 2013
External links
- Climate change (Food and Agriculture Organization of the United Nations)
- Climate adaptation & mitigation (CGIAR)
- Climate-smart agriculture (Worldbank)
![]() | Original source: https://en.wikipedia.org/wiki/Effects of climate change on agriculture.
Read more |