Biology:Wheat
Wheat | |
---|---|
![]() | |
Scientific classification ![]() | |
Kingdom: | Plantae |
Clade: | Tracheophytes |
Clade: | Angiosperms |
Clade: | Monocots |
Clade: | Commelinids |
Order: | Poales |
Family: | Poaceae |
Subfamily: | Pooideae |
Tribe: | Triticeae |
Genus: | Triticum L.[1] |
Type species | |
Triticum aestivum | |
Species[2] | |
List of Triticum species:
|
Wheat is a grass widely cultivated for its seed, a cereal grain that is a worldwide staple food. The many species of wheat together make up the genus Triticum (/ˈtrɪtɪkəm/);[3] the most widely grown is common wheat (T. aestivum). The archaeological record suggests that wheat was first cultivated in the regions of the Fertile Crescent around 9600 BC. Botanically, the wheat kernel is a caryopsis, a type of fruit.
Wheat is grown on more land area than any other food crop (220.7 million hectares or 545 million acres in 2021). World trade in wheat is greater than for all other crops combined. In 2021, world wheat production was 771 million tonnes (850 million short tons), making it the second most-produced cereal after maize (known as corn in the US and Australia; wheat is often called corn in other countries). Since 1960, world production of wheat and other grain crops has tripled and is expected to grow further through the middle of the 21st century. Global demand for wheat is increasing because of the usefulness of gluten to the food industry.
Wheat is an important source of carbohydrates. Globally, it is the leading source of vegetable proteins in human food, having a protein content of about 13%, which is relatively high compared to other major cereals but relatively low in protein quality (supplying essential amino acids). When eaten as the whole grain, wheat is a source of multiple nutrients and dietary fiber. In a small part of the general population, gluten – which comprises most of the protein in wheat – can trigger coeliac disease, noncoeliac gluten sensitivity, gluten ataxia, and dermatitis herpetiformis.
Description
Wheat is a stout grass of medium to tall height. Its stem is jointed and usually hollow, forming a straw. There can be many stems on one plant. It has long narrow leaves, their bases sheathing the stem, one above each joint. At the top of the stem is the flower head, containing some 20 to 100 flowers. Each flower contains both male and female parts. The flower, which is wind-pollinated, is housed in a pair of small leaflike glumes. The two (male) stamens and (female) stigmas protrude outside the glumes. The flowers are grouped into spikelets, each with between two and six flowers. Each fertilised carpel develops into a wheat grain or berry; botanically a fruit, it is often called a seed. The grains ripen to a golden yellow; a head of grain is called an ear.[4]
Leaves emerge from the shoot apical meristem in a telescoping fashion until the transition to reproduction i.e. flowering.[5] The last leaf produced by a wheat plant is known as the flag leaf. It is denser and has a higher photosynthetic rate than other leaves, to supply carbohydrate to the developing ear. In temperate countries the flag leaf, along with the second and third highest leaf on the plant, supply the majority of carbohydrate in the grain and their condition is paramount to yield formation.[6][7] Wheat is unusual among plants in having more stomata on the upper (adaxial) side of the leaf, than on the under (abaxial) side.[8] It has been theorised that this might be an effect of it having been domesticated and cultivated longer than any other plant.[9] Winter wheat generally produces up to 15 leaves per shoot and spring wheat up to 9[10] and winter crops may have up to 35 tillers (shoots) per plant (depending on cultivar).[10]
Wheat roots are among the deepest of arable crops, extending as far down as 2 metres (6 ft 7 in).[11] While the roots of a wheat plant are growing, the plant also accumulates an energy store in its stem, in the form of fructans,[12] which helps the plant to yield under drought and disease pressure,[13] but it has been observed that there is a trade-off between root growth and stem non-structural carbohydrate reserves. Root growth is likely to be prioritised in drought-adapted crops, while stem non-structural carbohydrate is prioritised in varieties developed for countries where disease is a bigger issue.[14]
Depending on variety, wheat may be awned or not awned. Producing awns incurs a cost in grain number,[15] but wheat awns photosynthesise more efficiently than their leaves with regards to water usage,[16] so awns are much more frequent in varieties of wheat grown in hot drought-prone countries than those generally seen in temperate countries. For this reason, awned varieties could become more widely grown due to climate change. In Europe, however, a decline in climate resilience of wheat has been observed.[17]
History
Domestication
Hunter-gatherers in West Asia harvested wild wheats for thousands of years before they were domesticated,[18] perhaps as early as 21,000 BC,[19] but they formed a minor component of their diets.[20] This phase of pre-domestication cultivation lasted at least a thousand years, during which early cultivars were spread around the region and slowly developed the traits that would come to characterise their domesticated forms.[21]
Repeated harvesting and sowing of the grains of wild grasses led to the creation of domestic strains, as mutant forms ('sports') of wheat were more amenable to cultivation. In domesticated wheat, grains are larger, and the seeds (inside the spikelets) remain attached to the ear by a toughened rachis during harvesting.[22] In wild strains, a more fragile rachis allows the ear to shatter easily, dispersing the spikelets.[23] Selection for larger grains and non-shattering heads by farmers might not have been deliberately intended, but simply have occurred because these traits made gathering the seeds easier; nevertheless such 'incidental' selection was an important part of crop domestication. As the traits that improve wheat as a food source involve the loss of the plant's natural seed dispersal mechanisms, highly domesticated strains of wheat cannot survive in the wild.[24]
Wild einkorn wheat (T. monococcum subsp. boeoticum) grows across Southwest Asia in open parkland and steppe environments.[25] It comprises three distinct races, only one of which, native to Southeast Anatolia, was domesticated.[26] The main feature that distinguishes domestic einkorn from wild is that its ears do not shatter without pressure, making it dependent on humans for dispersal and reproduction.[25] It also tends to have wider grains.[25] Wild einkorn was collected at sites such as Tell Abu Hureyra (c. 10,700–9000 BC) and Mureybet (c. 9800–9300 BC), but the earliest archaeological evidence for the domestic form comes after c. 8800 BC in southern Turkey, at Çayönü, Cafer Höyük, and possibly Nevalı Çori.[25] Genetic evidence indicates that it was domesticated in multiple places independently.[26]
Wild emmer wheat (T. turgidum subsp. dicoccoides) is less widespread than einkorn, favouring the rocky basaltic and limestone soils found in the hilly flanks of the Fertile Crescent.[25] It is more diverse, with domesticated varieties falling into two major groups: hulled or non-shattering, in which threshing separates the whole spikelet; and free-threshing, where the individual grains are separated. Both varieties probably existed in prehistory, but over time free-threshing cultivars became more common.[25] Wild emmer was first cultivated in the southern Levant, as early as 9600 BC.[27][28] Genetic studies have found that, like einkorn, it was domesticated in southeastern Anatolia, but only once.[26][29] The earliest secure archaeological evidence for domestic emmer comes from Çayönü, c. 8300–7600 BC, where distinctive scars on the spikelets indicated that they came from a hulled domestic variety.[25] Slightly earlier finds have been reported from Tell Aswad in Syria, c. 8500–8200 BC, but these were identified using a less reliable method based on grain size.[25]
Early farming
Einkorn and emmer are considered two of the founder crops cultivated by the first farming societies in Neolithic West Asia.[25] These communities also cultivated naked wheats (T. aestivum and T. durum) and a now-extinct domesticated form of Zanduri wheat (T. timopheevii),[30] as well as a wide variety of other cereal and non-cereal crops.[31] Wheat was relatively uncommon for the first thousand years of the Neolithic (when barley predominated), but became a staple after around 8500 BC.[31] Early wheat cultivation did not demand much labour. Initially, farmers took advantage of wheat's ability to establish itself in annual grasslands by enclosing fields against grazing animals and re-sowing stands after they had been harvested, without the need to systematically remove vegetation or till the soil.[32] They may also have exploited natural wetlands and floodplains to practice décrue farming, sowing seeds in the soil left behind by receding floodwater.[33][34][35] It was harvested with stone-bladed sickles.[36] The ease of storing wheat and other cereals led farming households to become gradually more reliant on it over time, especially after they developed individual storage facilities that were large enough to hold more than a year's supply.[37]
Wheat grain was stored after threshing, with the chaff removed.[37] It was then processed into flour using ground stone mortars.[38] Bread made from ground einkorn and the tubers of a form of club rush (Bolboschoenus glaucus) was made as early as 12,400 BC.[39] At Çatalhöyük (c. 7100–6000 BC), both wholegrain wheat and flour was used to prepare bread, porridge and gruel.[40][41] Apart from food, wheat may also have been important to Neolithic societies as a source of straw, which could be used for fuel, wicker-making, or wattle and daub construction.[42]
Spread
Domestic wheat was quickly spread to regions where its wild ancestors did not grow naturally. Emmer was introduced to Cyprus as early as 8600 BC and einkorn c. 7500 BC;[43][44] emmer reached Greece by 6500 BC, Egypt shortly after 6000 BC, and Germany and Spain by 5000 BC.[45] "The early Egyptians were developers of bread and the use of the oven and developed baking into one of the first large-scale food production industries."[46] By 4000 BC, wheat had reached the British Isles and Scandinavia.[47][48][49] Wheat likely appeared in China 's lower Yellow River around 2600 BC.[50]
The oldest evidence for hexaploid wheat has been confirmed through DNA analysis of wheat seeds, dating to around 6400–6200 BC, recovered from Çatalhöyük.[51] (As of 2023) the earliest known wheat with sufficient gluten for yeasted breads was found in a granary at Assiros in Macedonia dated to 1350 BC.[52] From the Middle East, wheat continued to spread across Europe and to the Americas in the Columbian exchange. In the British Isles, wheat straw (thatch) was used for roofing in the Bronze Age, and remained in common use until the late 19th century.[53][54] White wheat bread was historically a high status food, but during the nineteenth century it became in Britain an item of mass consumption, displacing oats, barley and rye from diets in the North of the country. It became "a sign of a high degree of culture".[55] After 1860, the enormous expansion of wheat production in the United States flooded the world market, lowering prices by 40%, and (along with the expansion of potato growing) made a major contribution to the nutritional welfare of the poor.[56]
Sumerian cylinder seal impression dating to c. 3200 BC showing an ensi and his acolyte feeding a sacred herd wheat stalks; Ninurta was an agricultural deity and, in a poem known as the "Sumerian Georgica", he offers detailed advice on farming
Evolution
Phylogeny
Some wheat species are diploid, with two sets of chromosomes, but many are stable polyploids, with four sets of chromosomes (tetraploid) or six (hexaploid).[57] Einkorn wheat (Triticum monococcum) is diploid (AA, two complements of seven chromosomes, 2n=14).[58] Most tetraploid wheats (e.g. emmer and durum wheat) are derived from wild emmer, T. dicoccoides. Wild emmer is itself the result of a hybridization between two diploid wild grasses, T. urartu and a wild goatgrass such as Ae. speltoides.[59] The hybridization that formed wild emmer (AABB, four complements of seven chromosomes in two groups, 4n=28) occurred in the wild, long before domestication, and was driven by natural selection. Hexaploid wheats evolved in farmers' fields as wild emmer hybridized with another goatgrass, Ae. squarrosa or Ae. tauschii, to make the hexaploid wheats including bread wheat.[57][60]
A 2007 molecular phylogeny of the wheats gives the following not fully-resolved cladogram of major cultivated species; the large amount of hybridisation makes resolution difficult. Markings like "6N" indicate the degree of polyploidy of each species:[57]
Triticeae |
| ||||||||||||||||||||||||||||||||||||||||||
Taxonomy
During 10,000 years of cultivation, numerous forms of wheat, many of them hybrids, have developed under a combination of artificial and natural selection. This complexity and diversity of status has led to much confusion in the naming of wheats.[61][62]
Major species
Hexaploid species (6N)
- Common wheat or bread wheat (T. aestivum) – The most widely cultivated species in the world.[63]
- Spelt (T. spelta) – Another species largely replaced by bread wheat, but in the 21st century grown, often organically, for artisanal bread and pasta.[64]
Tetraploid species (4N)
- Durum (T. durum) – A wheat widely used today, and the second most widely cultivated wheat.[63]
- Emmer (T. turgidum subsp. dicoccum and T. t. conv. durum) – A species cultivated in ancient times, derived from wild emmer, T. dicoccoides, but no longer in widespread use.[65]
- Khorasan or Kamut (T. turgidum ssp. turanicum, also called T. turanicum) is an ancient grain type; Khorasan is a historical region in modern-day Afghanistan and the northeast of Iran. The grain is twice the size of modern wheat and has a rich nutty flavor.[66]
Diploid species (2N)
- Einkorn (T. monococcum). Domesticated from wild einkorn, T. boeoticum, at the same time as emmer wheat.[67]
Hulled versus free-threshing species
The four wild species of wheat, along with the domesticated varieties einkorn,[68] emmer[69] and spelt,[70] have hulls. This more primitive morphology (in evolutionary terms) consists of toughened glumes that tightly enclose the grains, and (in domesticated wheats) a semi-brittle rachis that breaks easily on threshing. The result is that when threshed, the wheat ear breaks up into spikelets. To obtain the grain, further processing, such as milling or pounding, is needed to remove the hulls or husks. Hulled wheats are often stored as spikelets because the toughened glumes give good protection against pests of stored grain.[68] In free-threshing (or naked) forms, such as durum wheat and common wheat, the glumes are fragile and the rachis tough. On threshing, the chaff breaks up, releasing the grains.[71]
As a food
Naming of grain classes
Wheat grain classes are named by color, season, and hardness.[72] The classes used in the United States are:[73][74]
- Durum – Hard, translucent, light-colored grain used to make semolina flour for pasta and bulghur; high in protein, specifically, gluten protein.[73][74]
- Hard Red Spring – Hard, brownish, high-protein wheat used for bread and hard baked goods. Bread flour and high-gluten flours are commonly made from hard red spring wheat. It is primarily traded on the Minneapolis Grain Exchange.[73][74]
- Hard Red Winter – Hard, brownish, mellow high-protein wheat used for bread, hard baked goods and as an adjunct in other flours to increase protein in pastry flour for pie crusts. Some brands of unbleached all-purpose flours are commonly made from hard red winter wheat alone. It is primarily traded on the Kansas City Board of Trade. Many varieties grown from Kansas south are descendant from a variety known as "turkey red", which was brought to Kansas by Mennonite immigrants from Russia.[73][74][75] Marquis wheat was developed to prosper in the shorter growing season in Canada, and is grown as far south as southern Nebraska.[76]
- Soft Red Winter – Soft, low-protein wheat used for cakes, pie crusts, biscuits, and muffins. Cake flour, pastry flour, and some self-rising flours with baking powder and salt added, for example, are made from soft red winter wheat. It is primarily traded on the Chicago Board of Trade.[73][74]
- Hard White – Hard, light-colored, opaque, chalky, medium-protein wheat planted in dry, temperate areas. Used for bread and brewing.[73][74]
- Soft White – Soft, light-colored, very low protein wheat grown in temperate moist areas. Used for pie crusts and pastry.[73][74]
Food value and uses
Nutritional value per 100 g (3.5 oz) | |
---|---|
Energy | 1,368 kJ (327 kcal) |
71.18 g | |
Sugars | 0.41 |
Dietary fiber | 12.2 g |
1.54 g | |
12.61 g | |
Vitamins | Quantity %DV† |
Thiamine (B1) | 33% 0.383 mg |
Riboflavin (B2) | 10% 0.115 mg |
Niacin (B3) | 36% 5.464 mg |
Pantothenic acid (B5) | 19% 0.954 mg |
Vitamin B6 | 23% 0.3 mg |
Folate (B9) | 10% 38 μg |
Choline | 6% 31.2 mg |
Vitamin E | 7% 1.01 mg |
Vitamin K | 2% 1.9 μg |
Minerals | Quantity %DV† |
Calcium | 3% 29 mg |
Iron | 25% 3.19 mg |
Magnesium | 35% 126 mg |
Manganese | 190% 3.985 mg |
Phosphorus | 41% 288 mg |
Potassium | 8% 363 mg |
Sodium | 0% 2 mg |
Zinc | 28% 2.65 mg |
Other constituents | Quantity |
Water | 13.1 g |
Selenium | 70.7 µg |
| |
†Percentages are roughly approximated using US recommendations for adults. Source: USDA Nutrient Database |
Wheat is a staple cereal worldwide.[77][58] Raw wheat berries can be ground into flour or, using hard durum wheat only, can be ground into semolina; germinated and dried creating malt; crushed or cut into cracked wheat; parboiled (or steamed), dried, crushed and de-branned into bulgur also known as groats.[78] If the raw wheat is broken into parts at the mill, as is usually done, the outer husk or bran can be used in several ways. Wheat is a major ingredient in such foods as bread, porridge, crackers, biscuits, muesli, pancakes, pasta, pies, pastries, pizza, semolina, cakes, cookies, muffins, rolls, doughnuts, gravy, beer, vodka, boza (a fermented beverage), and breakfast cereals.[79] In manufacturing wheat products, gluten is valuable to impart viscoelastic functional qualities in dough,[80] enabling the preparation of diverse processed foods such as breads, noodles, and pasta that facilitate wheat consumption.[81][82]
Nutrition
Raw red winter wheat is 13% water, 71% carbohydrates including 12% dietary fiber, 13% protein, and 2% fat (table). Some 75–80% of the protein content is as gluten.[80] In a reference amount of 100 grams (3.5 oz), wheat provides 1,368 kilojoules (327 kilocalories) of food energy and is a rich source (20% or more of the Daily Value, DV) of multiple dietary minerals, such as manganese, phosphorus, magnesium, zinc, and iron (table). The B vitamins, niacin (36% DV), thiamine (33% DV), and vitamin B6 (23% DV), are present in significant amounts (table).
Wheat is a significant source of vegetable proteins in human food, having a relatively high protein content compared to other major cereals.[83] However, wheat proteins have a low quality for human nutrition, according to the DIAAS protein quality evaluation method.[84][85] Though they contain adequate amounts of the other essential amino acids, at least for adults, wheat proteins are deficient in the essential amino acid lysine.[82][86] Because the proteins present in the wheat endosperm (gluten proteins) are particularly poor in lysine, white flours are more deficient in lysine compared with whole grains.[82] Significant efforts in plant breeding are made to develop lysine-rich wheat varieties, without success, (As of 2017).[87] Supplementation with proteins from other food sources (mainly legumes) is commonly used to compensate for this deficiency,[88] since the limitation of a single essential amino acid causes the others to break down and become excreted, which is especially important during growth.[82]
Health advisories
Consumed worldwide by billions of people, wheat is a significant food for human nutrition, particularly in the least developed countries where wheat products are primary foods.[82][89] When eaten as the whole grain, wheat supplies multiple nutrients and dietary fiber recommended for children and adults.[81][82][90][91] In genetically susceptible people, wheat gluten can trigger coeliac disease.[80][92] Coeliac disease affects about 1% of the general population in developed countries.[92][93] The only known effective treatment is a strict lifelong gluten-free diet.[92] While coeliac disease is caused by a reaction to wheat proteins, it is not the same as a wheat allergy.[92][93] Other diseases triggered by eating wheat are non-coeliac gluten sensitivity[93][94] (estimated to affect 0.5% to 13% of the general population[95]), gluten ataxia, and dermatitis herpetiformis.[94] Certain short-chain carbohydrates present in wheat, known as FODMAPs (mainly frutose polymers), may be the cause of non-coeliac gluten sensitivity. (As of 2019), reviews have concluded that FODMAPs only explain certain gastrointestinal symptoms, such as bloating, but not the extra-digestive symptoms that people with non-coeliac gluten sensitivity may develop health disorders.[96][97][98] Other wheat proteins, amylase-trypsin inhibitors, have been identified as the possible activator of the innate immune system in coeliac disease and non-coeliac gluten sensitivity.[97][98] These proteins are part of the plant's natural defense against insects and may cause intestinal inflammation in humans.[97][99]
Production and consumption
Global
Country | Millions of tonnes |
---|---|
![]() |
136.9 |
![]() |
109.6 |
![]() |
78.1 |
![]() |
44.8 |
![]() |
36.6 |
![]() |
32.2 |
Template:Pak | 27.5 |
World | 771 |
Source: UN Food and Agriculture Organization[100] |
Production of wheat (2019)[101]
In 2021, world wheat production was 771 million tonnes, led by China, India, and Russia which collectively provided 42% of the world total.[100] (As of 2019), the largest exporters were Russia (32 million tonnes), United States (27), Canada (23) and France (20), while the largest importers were Indonesia (11 million tonnes), Egypt (10.4) and Turkey (10.0).[102] In 2021, wheat was grown on 220.7 million hectares or 545 million acres worldwide, more than any other food crop.[103] World trade in wheat is greater than for all other crops combined.[104] Global demand for wheat is increasing due to the unique viscoelastic and adhesive properties of gluten proteins, which facilitate the production of processed foods, whose consumption is increasing as a result of the worldwide industrialization process and westernization of diets.[82][105]
Historical factors
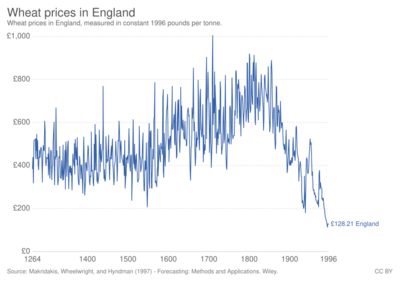
Wheat became a central agriculture endeavor in the worldwide British Empire in the 19th century, and remains of great importance in Australia, Canada and India.[107] In Australia, with vast lands and a limited work force, expanded production depended on technological advances, especially regarding irrigation and machinery. By the 1840s there were 900 growers in South Australia. They used "Ridley's Stripper", to remove the heads of grain, a reaper-harvester perfected by John Ridley in 1843.[108] In Canada, modern farm implements made large scale wheat farming possible from the late 1840s. By 1879, Saskatchewan was the center, followed by Alberta, Manitoba and Ontario, as the spread of railway lines allowed easy exports to Britain. By 1910, wheat made up 22% of Canada's exports, rising to 25% in 1930 despite the sharp decline in prices during the worldwide Great Depression.[109] Efforts to expand wheat production in South Africa, Kenya and India were stymied by low yields and disease. However, by 2000 India had become the second largest producer of wheat in the world.[110] In the 19th century the American wheat frontier moved rapidly westward. By the 1880s 70% of American exports went to British ports. The first successful grain elevator was built in Buffalo in 1842.[111] The cost of transport fell rapidly. In 1869 it cost 37 cents to transport a bushel of wheat from Chicago to Liverpool. In 1905 it was 10 cents.[112]
In the 20th century, global wheat output expanded by about 5-fold, but until about 1955 most of this reflected increases in wheat crop area, with lesser (about 20%) increases in crop yields per unit area. After 1955 however, there was a ten-fold increase in the rate of wheat yield improvement per year, and this became the major factor allowing global wheat production to increase. Thus technological innovation and scientific crop management with synthetic nitrogen fertilizer, irrigation and wheat breeding were the main drivers of wheat output growth in the second half of the century. There were some significant decreases in wheat crop area, for instance in North America.[113] Better seed storage and germination ability (and hence a smaller requirement to retain harvested crop for next year's seed) is another 20th-century technological innovation. In Medieval England, farmers saved one-quarter of their wheat harvest as seed for the next crop, leaving only three-quarters for food and feed consumption. By 1999, the global average seed use of wheat was about 6% of output.[114] In the 21st century, rising temperatures associated with global warming are reducing wheat yield in several locations.[115]
Peak wheat
Agronomy
Growing wheat
Wheat is an annual crop. It can be planted in autumn and harvested in early summer as winter wheat in climates that are not too severe, or planted in spring and harvested in autumn as spring wheat. It is normally planted after tilling the soil by ploughing and then harrowing to kill weeds and create an even surface. The seeds are then scattered on the surface, or drilled into the soil in rows. Winter wheat lies dormant during a winter freeze. It needs to develop to a height of 10 to 15 cm before the cold intervenes, so as to be able to survive the winter; it requires a period with the temperature at or near freezing, its dormancy then being broken by the thaw or rise in temperature. Spring wheat does not undergo dormancy. Wheat requires a deep soil, preferably a loam with organic matter, and available minerals including soil nitrogen, phosphorus, and potassium. An acid and peaty soil is not suitable. Wheat needs some 30 to 38 cm of rain in the growing season to form a good crop of grain.[116]
The farmer may intervene while the crop is growing to add fertilizer, water by irrigation, or pesticides such as herbicides to kill broad-leaved weeds or insecticides to kill insect pests. The farmer may assess soil minerals, soil water, weed growth, or the arrival of pests to decide timely and cost-effective corrective actions, and crop ripeness and water content to select the right moment to harvest. Harvesting involves reaping, cutting the stems to gather the crop; and threshing, breaking the ears to release the grain; both steps are carried out by a combine harvester. The grain is then dried so that it can be stored safe from mould fungi.[116]
Crop development
File:Wheat developmental stages.tif
Wheat normally needs between 110 and 130 days between sowing and harvest, depending upon climate, seed type, and soil conditions. Optimal crop management requires that the farmer have a detailed understanding of each stage of development in the growing plants. In particular, spring fertilizers, herbicides, fungicides, and growth regulators are typically applied only at specific stages of plant development. For example, it is currently recommended that the second application of nitrogen is best done when the ear (not visible at this stage) is about 1 cm in size (Z31 on Zadoks scale). Knowledge of stages is also important to identify periods of higher risk from the climate. Farmers benefit from knowing when the 'flag leaf' (last leaf) appears, as this leaf represents about 75% of photosynthesis reactions during the grain filling period, and so should be preserved from disease or insect attacks to ensure a good yield. Several systems exist to identify crop stages, with the Feekes and Zadoks scales being the most widely used. Each scale is a standard system which describes successive stages reached by the crop during the agricultural season.[117] For example, the stage of pollen formation from the mother cell, and the stages between anthesis and maturity, are susceptible to high temperatures, and this adverse effect is made worse by water stress.[118]
Anthesis stage
Farming techniques
Technological advances in soil preparation and seed placement at planting time, use of crop rotation and fertilizers to improve plant growth, and advances in harvesting methods have all combined to promote wheat as a viable crop. When the use of seed drills replaced broadcasting sowing of seed in the 18th century, another great increase in productivity occurred. Yields of pure wheat per unit area increased as methods of crop rotation were applied to land that had long been in cultivation, and the use of fertilizers became widespread.[119]
Improved agricultural husbandry has more recently included pervasive automation, starting with the use of threshing machines,[120] and progressing to large and costly machines like the combine harvester which greatly increased productivity.[121] At the same time, better varieties such as Norin 10 wheat, developed in Japan in the 1930s,[122] or the dwarf wheat developed by Norman Borlaug in the Green Revolution, greatly increased yields.[123][124]
In addition to gaps in farming system technology and knowledge, some large wheat grain-producing countries have significant losses after harvest at the farm and because of poor roads, inadequate storage technologies, inefficient supply chains and farmers' inability to bring the produce into retail markets dominated by small shopkeepers. Some 10% of total wheat production is lost at farm level, another 10% is lost because of poor storage and road networks, and additional amounts are lost at the retail level.[125]
In the Punjab region of the Indian subcontinent, as well as North China, irrigation has been a major contributor to increased grain output. More widely over the last 40 years, a massive increase in fertilizer use together with the increased availability of semi-dwarf varieties in developing countries, has greatly increased yields per hectare.[126] In developing countries, use of (mainly nitrogenous) fertilizer increased 25-fold in this period. However, farming systems rely on much more than fertilizer and breeding to improve productivity. A good illustration of this is Australian wheat growing in the southern winter cropping zone, where, despite low rainfall (300 mm), wheat cropping is successful even with relatively little use of nitrogenous fertilizer. This is achieved by crop rotation with leguminous pastures. The inclusion of a canola crop in the rotations has boosted wheat yields by a further 25%.[127] In these low rainfall areas, better use of available soil-water (and better control of soil erosion) is achieved by retaining the stubble after harvesting and by minimizing tillage.[128]
Combine harvester cuts the wheat stems, threshes the wheat, crushes the chaff and blows it across the field, and loads the grain onto a tractor trailer.
Pests and diseases
Pests[129] – or pests and diseases, depending on the definition – consume 21.47% of the world's wheat crop annually.[130]
Diseases
There are many wheat diseases, mainly caused by fungi, bacteria, and viruses.[131] Plant breeding to develop new disease-resistant varieties, and sound crop management practices are important for preventing disease. Fungicides, used to prevent the significant crop losses from fungal disease, can be a significant variable cost in wheat production. Estimates of the amount of wheat production lost owing to plant diseases vary between 10 and 25% in Missouri.[132] A wide range of organisms infect wheat, of which the most important are viruses and fungi.[133]
The main wheat-disease categories are:
- Seed-borne diseases: these include seed-borne scab, seed-borne Stagonospora (previously known as Septoria), common bunt (stinking smut), and loose smut. These are managed with fungicides.[134]
- Leaf- and head- blight diseases: Powdery mildew, leaf rust, Septoria tritici leaf blotch, Stagonospora (Septoria) nodorum leaf and glume blotch, and Fusarium head scab.[134][135]
- Crown and root rot diseases: Two of the more important of these are 'take-all' and Cephalosporium stripe. Both of these diseases are soil borne.[134]
- Stem rust diseases: Caused by Puccinia graminis f. sp. tritici (basidiomycete) fungi e.g. Ug99[136]
- Wheat blast: Caused by Magnaporthe oryzae Triticum.[137]
- Viral diseases: Wheat spindle streak mosaic (yellow mosaic) and barley yellow dwarf are the two most common viral diseases. Control can be achieved by using resistant varieties.[134]
A historically significant disease of cereals including wheat, though commoner in rye is ergot; it is unusual among plant diseases in also causing sickness in humans who ate grain contaminated with the fungus involved, Claviceps purpurea.[138]
Animal pests
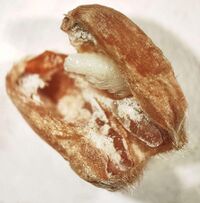
Among insect pests of wheat is the wheat stem sawfly, a chronic pest in the Northern Great Plains of the United States and in the Canadian Prairies.[139] Wheat is the food plant of the larvae of some Lepidoptera (butterfly and moth) species including the flame, rustic shoulder-knot, setaceous Hebrew character and turnip moth. Early in the season, many species of birds and rodents feed upon wheat crops. These animals can cause significant damage to a crop by digging up and eating newly planted seeds or young plants. They can also damage the crop late in the season by eating the grain from the mature spike. Recent post-harvest losses in cereals amount to billions of dollars per year in the United States alone, and damage to wheat by various borers, beetles and weevils is no exception.[140] Rodents can also cause major losses during storage, and in major grain growing regions, field mice numbers can sometimes build up explosively to plague proportions because of the ready availability of food.[141] To reduce the amount of wheat lost to post-harvest pests, Agricultural Research Service scientists have developed an "insect-o-graph", which can detect insects in wheat that are not visible to the naked eye. The device uses electrical signals to detect the insects as the wheat is being milled. The new technology is so precise that it can detect 5–10 infested seeds out of 30,000 good ones.[142]
Breeding objectives
In traditional agricultural systems, wheat populations consist of landraces, informal farmer-maintained populations that often maintain high levels of morphological diversity. Although landraces of wheat are no longer extensively grown in Europe and North America, they continue to be important elsewhere. The origins of formal wheat breeding lie in the nineteenth century, when single line varieties were created through selection of seed from a single plant noted to have desired properties. Modern wheat breeding developed in the first years of the twentieth century and was closely linked to the development of Mendelian genetics. The standard method of breeding inbred wheat cultivars is by crossing two lines using hand emasculation, then selfing or inbreeding the progeny. Selections are identified (shown to have the genes responsible for the varietal differences) ten or more generations before release as a variety or cultivar.[143]
Major breeding objectives include high grain yield, good quality, disease- and insect resistance and tolerance to abiotic stresses, including mineral, moisture and heat tolerance. Wheat has been the subject of mutation breeding, with the use of gamma-, x-rays, ultraviolet light (collectively, radiation breeding), and sometimes harsh chemicals. The varieties of wheat created through these methods are in the hundreds (going as far back as 1960), more of them being created in higher populated countries such as China.[144] Bread wheat with high grain iron and zinc content has been developed through gamma radiation breeding,[145] and through conventional selection breeding.[146] International wheat breeding is led by the International Maize and Wheat Improvement Center in Mexico. ICARDA is another major public sector international wheat breeder, but it was forced to relocate from Syria to Lebanon in the Syrian Civil War.[147]
Pathogens and wheat are in a constant process of coevolution.[148] Spore-producing wheat rusts are substantially adapted towards successful spore propagation, which is essentially to say its R0.[148] These pathogens tend towards high-R0 evolutionary attractors.[148]
For higher yields
The presence of certain versions of wheat genes has been important for crop yields. Genes for the 'dwarfing' trait, first used by Japanese wheat breeders to produce Norin 10 short-stalked wheat, have had a huge effect on wheat yields worldwide, and were major factors in the success of the Green Revolution in Mexico and Asia, an initiative led by Norman Borlaug.[149] Dwarfing genes enable the carbon that is fixed in the plant during photosynthesis to be diverted towards seed production, and they also help prevent the problem of lodging.[150] "Lodging" occurs when an ear stalk falls over in the wind and rots on the ground, and heavy nitrogenous fertilization of wheat makes the grass grow taller and become more susceptible to this problem.[151] By 1997, 81% of the developing world's wheat area was planted to semi-dwarf wheats, giving both increased yields and better response to nitrogenous fertilizer.[152]
T. turgidum subsp. polonicum, known for its longer glumes and grains, has been bred into main wheat lines for its grain size effect, and likely has contributed these traits to Triticum petropavlovskyi and the Portuguese landrace group Arrancada.[153] As with many plants, MADS-box influences flower development, and more specifically, as with other agricultural Poaceae, influences yield. Despite that importance, (As of 2021) little research has been done into MADS-box and other such spikelet and flower genetics in wheat specifically.[153]
The world record wheat yield is about 17 tonnes per hectare (15,000 pounds per acre), reached in New Zealand in 2017.[154] A project in the UK, led by Rothamsted Research has aimed to raise wheat yields in the country to 20 t/ha (18,000 lb/acre) by 2020, but in 2018 the UK record stood at 16 t/ha (14,000 lb/acre), and the average yield was just 8 t/ha (7,100 lb/acre).[155][156]
For disease resistance
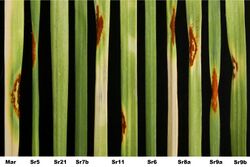
Wild grasses in the genus Triticum and related genera, and grasses such as rye have been a source of many disease-resistance traits for cultivated wheat breeding since the 1930s.[157] Some resistance genes have been identified against Pyrenophora tritici-repentis, especially races 1 and 5, those most problematic in Kazakhstan.[158] Wild relative, Aegilops tauschii is the source of several genes effective against TTKSK/Ug99 - Sr33, Sr45, Sr46, and SrTA1662 - of which Sr33 and SrTA1662 are the work of Olson et al., 2013, and Sr45 and Sr46 are also briefly reviewed therein.[159]
- Lr67 is an R gene, a dominant negative for partial adult resistance discovered and molecularly characterized by Moore et al., 2015. (As of 2018) Lr67 is effective against all races of leaf, stripe, and stem rusts, and powdery mildew (Blumeria graminis). This is produced by a mutation of two amino acids in what is predicted to be a hexose transporter. The product then heterodimerizes with the susceptible's product, with the downstream result of reducing glucose uptake.[160]
- Lr34 is widely deployed in cultivars due to its abnormally broad effectiveness, conferring resistance against leaf- and stripe-rusts, and powdery mildew.[161] Krattinger et al. 2009 finds Lr34 to also be an ABC transporter and conclude that this is the probably means of its effectiveness[161][162] and the reason that it produces a 'slow rusting'/adult resistance phenotype.[162]
- Pm8 is a widely used powdery mildew resistance introgressed from rye (Secale cereale).[163] It comes from the rye 1R chromosome, a source of many resistances since the 1960s.[163]
Resistance to Fusarium head blight (FHB, Fusarium ear blight) is also an important breeding target. Marker-assisted breeding panels involving kompetitive allele specific PCR can be used. Singh et al. 2019 identify a KASP genetic marker for a pore-forming toxin-like gene providing FHB resistance.[164]
To create hybrid vigor
Because wheat self-pollinates, creating hybrid seed to provide the possible benefits of heterosis, hybrid vigor (as in the familiar F1 hybrids of maize), is extremely labor-intensive; the high cost of hybrid wheat seed relative to its moderate benefits have kept farmers from adopting them widely[165][166] despite nearly 90 years of effort.[167][143] Commercial hybrid wheat seed has been produced using chemical hybridizing agents, plant growth regulators that selectively interfere with pollen development, or naturally occurring cytoplasmic male sterility systems. Hybrid wheat has been a limited commercial success in Europe (particularly France), the United States and South Africa.[168]
Synthetic hexaploids made by crossing the wild goatgrass wheat ancestor Aegilops tauschii,[169] and other Aegilops,[170] and various durum wheats are now being deployed, and these increase the genetic diversity of cultivated wheats.[171][172][173]
For gluten content
Modern bread wheat varieties have been cross-bred to contain greater amounts of gluten,[174] which affords significant advantages for improving the quality of breads and pastas from a functional point of view.[175] However, a 2020 study that grew and analyzed 60 wheat cultivars from between 1891 and 2010 found no changes in albumin/globulin and gluten contents over time. "Overall, the harvest year had a more significant effect on protein composition than the cultivar. At the protein level, we found no evidence to support an increased immunostimulatory potential of modern winter wheat."[176]
For water efficiency
Stomata (or leaf pores) are involved in both uptake of carbon dioxide gas from the atmosphere and water vapor losses from the leaf due to water transpiration. Basic physiological investigation of these gas exchange processes has yielded carbon isotope based method used for breeding wheat varieties with improved water-use efficiency. These varieties can improve crop productivity in rain-fed dry-land wheat farms.[177]
For insect resistance
The gene Sm1 protects against the orange wheat blossom midge.[178][179][180][181]
Genomics
Decoding the genome
In 2010, 95% of the genome of Chinese Spring line 42 wheat was decoded.[182] This genome was released in a basic format for scientists and plant breeders to use but was not fully annotated.[183] In 2012, an essentially complete gene set of bread wheat was published.[184] Random shotgun libraries of total DNA and cDNA from the T. aestivum cv. Chinese Spring (CS42) were sequenced to generate 85 Gb of sequence (220 million reads) and identified between 94,000 and 96,000 genes.[184] In 2018, a more complete Chinese Spring genome was released by a different team.[185] In 2020, 15 genome sequences from various locations and varieties around the world were reported, with examples of their own use of the sequences to localize particular insect and disease resistance factors.[180] Wheat Blast Resistance is controlled by R genes which are highly race-specific.[137]
Genetic engineering
For decades, the primary genetic modification technique has been non-homologous end joining (NHEJ). However, since its introduction, the CRISPR/Cas9 tool has been extensively adopted, for example:
- To intentionally damage three homologs of TaNP1 (a glucose-methanol-choline oxidoreductase gene) to produce a novel male sterility trait, by Li et al. 2020[186]
- Blumeria graminis f.sp. tritici resistance has been produced by Shan et al. 2013 and Wang et al. 2014 by editing one of the mildew resistance locus o genes (more specifically one of the Triticum aestivum MLO (TaMLO) genes)[186]
- Triticum aestivum EDR1 (TaEDR1) (the EDR1 gene, which inhibits Bmt resistance) has been knocked out by Zhang et al. 2017 to improve that resistance[186]
- Triticum aestivum HRC (TaHRC) has been disabled by Su et al. 2019 thus producing Gibberella zeae resistance.[186]
- Triticum aestivum Ms1 (TaMs1) has been knocked out by Okada et al. 2019 to produce another novel male sterility[186]
- and Triticum aestivum acetolactate synthase (TaALS) and Triticum aestivum acetyl-CoA-carboxylase (TaACC) were subjected to base changes by Zhang et al. 2019 (in two publications) to confer herbicide resistance to ALS inhibitors and ACCase inhibitors respectively[186]
(As of 2021) these examples illustrate the rapid deployment and results that CRISPR/Cas9 has shown in wheat disease resistance improvement.[186]
In art
The Dutch artist Vincent van Gogh created the series Wheat Fields between 1885 and 1890, consisting of dozens of paintings made mostly in different parts of rural France. They depict wheat crops, sometimes with farm workers, in varied seasons and styles, sometimes green, sometimes at harvest. Wheatfield with Crows was one of his last paintings, and is considered to be among his greatest works.[187][188]
In 1967, the American artist Thomas Hart Benton made his oil on wood painting Wheat, showing a row of uncut wheat plants, occupying almost the whole height of the painting, between rows of freshly-cut stubble. The painting is held by the Smithsonian American Art Museum.[189]
In 1982, the American conceptual artist Agnes Denes grew a two-acre field of wheat at Battery Park, Manhattan. The ephemeral artwork has been described as an act of protest. The harvested wheat was divided and sent to 28 world cities for an exhibition entitled "The International Art Show for the End of World Hunger".[190]
See also
- Effects of climate change on agriculture
- Gluten-free diet
- Intermediate wheatgrass: a perennial alternative to wheat
- Wheat germ oil
- Wheat production in the United States
- Wheat middlings
- Whole-wheat flour
References
- ↑ lectotype designated by Duistermaat, Blumea 32: 174 (1987)
- ↑ Serial No. 42236 ITIS 2002-09-22
- ↑ "Triticum". Merriam-Webster Dictionary. https://www.merriam-webster.com/dictionary/triticum.
- ↑ "wheat (plant)". britannica.com. http://www.britannica.com/EBchecked/topic/641558/wheat. Retrieved 23 December 2023.
- ↑ "Fertilising for High Yield and Quality – Cereals". https://www.ipipotash.org/udocs/ipi-bulletin-17-cereals.pdf.
- ↑ Pajević, Slobodanka; Krstić, Borivoj; Stanković, Živko; Plesničar, Marijana; Denčić, Srbislav (1999). "Photosynthesis of Flag and Second Wheat Leaves During Senescence". Cereal Research Communications 27 (1/2): 155–162. doi:10.1007/BF03543932.
- ↑ Araus, J. L.; Tapia, L.; Azcon-Bieto, J.; Caballero, A. (1986). "Photosynthesis, Nitrogen Levels, and Dry Matter Accumulation in Flag Wheat Leaves During Grain Filling". Biological Control of Photosynthesis. pp. 199–207. doi:10.1007/978-94-009-4384-1_18. ISBN 978-94-010-8449-9.
- ↑ Singh, Sarvjeet; Sethi, G.S. (1995). "Stomatal Size, Frequency and Distribution in Triticum Aestivum, Secale Cereale and Their Amphiploids". Cereal Research Communications 23 (1/2): 103–108.
- ↑ Milla, Rubén; De Diego-Vico, Natalia; Martín-Robles, Nieves (2013). "Shifts in stomatal traits following the domestication of plant species". Journal of Experimental Botany 64 (11): 3137–3146. doi:10.1093/jxb/ert147. PMID 23918960.
- ↑ 10.0 10.1 "Wheat Growth Guide". Agriculture and Horticulture Development Board. https://cereals.ahdb.org.uk/media/185687/g66-wheat-growth-guide.pdf.
- ↑ Das, N. R. (1 October 2008). Wheat Crop Management. Scientific Publishers. ISBN 9789387741287. https://books.google.com/books?id=XedeDwAAQBAJ&q=wheat+roots+2+metres&pg=PA39.
- ↑ Hogan, M. E.; Hendrix, J. E. (1986). "Labeling of Fructans in Winter Wheat Stems". Plant Physiology 80 (4): 1048–1050. doi:10.1104/pp.80.4.1048. PMID 16664718.
- ↑ Zhang, J.; Chen, W.; Dell, B.; Vergauwen, R.; Zhang, X.; Mayer, J. E.; Van Den Ende, W. (2015). "Wheat genotypic variation in dynamic fluxes of WSC components in different stem segments under drought during grain filling". Frontiers in Plant Science 6: 624. doi:10.3389/fpls.2015.00624. PMID 26322065.
- ↑ Lopes, Marta S.; Reynolds, Matthew P. (2010). "Partitioning of assimilates to deeper roots is associated with cooler canopies and increased yield under drought in wheat". Functional Plant Biology 37 (2): 147. doi:10.1071/FP09121.
- ↑ Rebetzke, G. J.; Bonnett, D. G.; Reynolds, M. P. (2016). "Awns reduce grain number to increase grain size and harvestable yield in irrigated and rainfed spring wheat". Journal of Experimental Botany 67 (9): 2573–2586. doi:10.1093/jxb/erw081. PMID 26976817.
- ↑ Duwayri, Mahmud (1984). "Effect of flag leaf and awn removal on grain yield and yield components of wheat grown under dryland conditions". Field Crops Research 8: 307–313. doi:10.1016/0378-4290(84)90077-7.
- ↑ Kahiluoto, Helena; Kaseva, Janne; Balek, Jan; Olesen, Jørgen E.; Ruiz-Ramos, Margarita et al. (2019). "Decline in climate resilience of European wheat". Proceedings of the National Academy of Sciences 116 (1): 123–128. doi:10.1073/pnas.1804387115. PMID 30584094. Bibcode: 2019PNAS..116..123K.
- ↑ Richter, Tobias; Maher, Lisa A. (2013). "Terminology, process and change: reflections on the Epipalaeolithic of South-west Asia". Levant 45 (2): 121–132. doi:10.1179/0075891413Z.00000000020. https://doi.org/10.1179/0075891413Z.00000000020.
- ↑ Piperno, Dolores R.; Weiss, Ehud; Holst, Irene; Nadel, Dani (August 2004). "Processing of wild cereal grains in the Upper Palaeolithic revealed by starch grain analysis". Nature 430 (7000): 670–673. doi:10.1038/nature02734. PMID 15295598. Bibcode: 2004Natur.430..670P. https://www.nature.com/articles/nature02734.
- ↑ Arranz-Otaegui, Amaia; González Carretero, Lara; Roe, Joe; Richter, Tobias (2018). ""Founder crops" v. wild plants: Assessing the plant-based diet of the last hunter-gatherers in southwest Asia". Quaternary Science Reviews 186: 263–283. doi:10.1016/j.quascirev.2018.02.011. Bibcode: 2018QSRv..186..263A. https://www.sciencedirect.com/science/article/pii/S0277379117306145.
- ↑ Fuller, Dorian Q.; Willcox, George; Allaby, Robin G. (2011). "Cultivation and domestication had multiple origins: arguments against the core area hypothesis for the origins of agriculture in the Near East". World Archaeology 43 (4): 628–652. doi:10.1080/00438243.2011.624747. https://doi.org/10.1080/00438243.2011.624747.
- ↑ Hughes, N.; Oliveira, H.R.; Fradgley, N.; Corke, F.; Cockram, J.; Doonan, J.H.; Nibau, C. (14 March 2019). "μCT trait analysis reveals morphometric differences between domesticated temperate small grain cereals and their wild relatives". The Plant Journal 99 (1): 98–111. doi:10.1111/tpj.14312. PMID 30868647.
- ↑ Tanno, K.; Willcox, G. (2006). "How fast was wild wheat domesticated?". Science 311 (5769): 1886. doi:10.1126/science.1124635. PMID 16574859.
- ↑ Purugganan, Michael D.; Fuller, Dorian Q. (1 February 2009). "The nature of selection during plant domestication". Nature (Springer) 457 (7231): 843–848. doi:10.1038/nature07895. PMID 19212403. Bibcode: 2009Natur.457..843P.
- ↑ 25.0 25.1 25.2 25.3 25.4 25.5 25.6 25.7 25.8 Zohary, Daniel; Hopf, Maria; Weiss, Ehud (2012). "Cereals". Domestication of Plants in the Old World (4 ed.). Oxford: Oxford University Press. doi:10.1093/acprof:osobl/9780199549061.001.0001. ISBN 978-0-19-954906-1.
- ↑ 26.0 26.1 26.2 Ozkan, H.; Brandolini, A.; Schäfer-Pregl, R.; Salamini, F. (2002). "AFLP analysis of a collection of tetraploid wheats indicates the origin of emmer and hard wheat domestication in southeast Turkey". Molecular Biology and Evolution 19 (10): 1797–1801. doi:10.1093/oxfordjournals.molbev.a004002. PMID 12270906.
- ↑ Feldman, Moshe; Kislev, Mordechai E. (2007). "Domestication of emmer wheat and evolution of free-threshing tetraploid wheat in "A Century of Wheat Research-From Wild Emmer Discovery to Genome Analysis", Published Online: 3 November 2008". Israel Journal of Plant Sciences 55 (3–4): 207–221. http://www.sciencefromisrael.com/app/home/contribution.asp?referrer=parent&backto=issue,2,14;journal,9,41;linkingpublicationresults,1:300170,1. Retrieved 6 July 2011.
- ↑ Colledge, Sue (2007). The origins and spread of domestic plants in southwest Asia and Europe. Left Coast Press. pp. 40–. ISBN 978-1-59874-988-5. https://books.google.com/books?id=D2nym35k_EcC&pg=PA40.
- ↑ Luo, M.-C.; Yang, Z.-L.; You, F. M.; Kawahara, T.; Waines, J. G.; Dvorak, J. (2007). "The structure of wild and domesticated emmer wheat populations, gene flow between them, and the site of emmer domestication". Theoretical and Applied Genetics 114 (6): 947–959. doi:10.1007/s00122-006-0474-0. PMID 17318496. https://doi.org/10.1007/s00122-006-0474-0.
- ↑ Czajkowska, Beata I.; Bogaard, Amy; Charles, Michael; Jones, Glynis; Kohler-Schneider, Marianne; Mueller-Bieniek, Aldona; Brown, Terence A. (2020-11-01). "Ancient DNA typing indicates that the "new" glume wheat of early Eurasian agriculture is a cultivated member of the Triticum timopheevii group". Journal of Archaeological Science 123: 105258. doi:10.1016/j.jas.2020.105258. Bibcode: 2020JArSc.123j5258C. https://www.sciencedirect.com/science/article/pii/S0305440320301795.
- ↑ 31.0 31.1 Arranz-Otaegui, Amaia; Roe, Joe (2023-09-01). "Revisiting the concept of the 'Neolithic Founder Crops' in southwest Asia". Vegetation History and Archaeobotany 32 (5): 475–499. doi:10.1007/s00334-023-00917-1. Bibcode: 2023VegHA..32..475A.
- ↑ Weide, Alexander; Green, Laura; Hodgson, John G.; Douché, Carolyne; Tengberg, Margareta; Whitlam, Jade; Dovrat, Guy; Osem, Yagil et al. (June 2022). "A new functional ecological model reveals the nature of early plant management in southwest Asia". Nature Plants 8 (6): 623–634. doi:10.1038/s41477-022-01161-7. PMID 35654954. https://www.nature.com/articles/s41477-022-01161-7.
- ↑ Sherratt, Andrew (February 1980). "Water, soil and seasonality in early cereal cultivation". World Archaeology 11 (3): 313–330. doi:10.1080/00438243.1980.9979770. http://www.tandfonline.com/doi/abs/10.1080/00438243.1980.9979770.
- ↑ Scott, James C. (2017). "The Domestication of Fire, Plants, Animals, and ... Us". Against the Grain: A Deep History of the Earliest States. New Haven: Yale University Press. p. 66. ISBN 978-0-3002-3168-7. https://books.google.com/books?id=UjYuDwAAQBAJ. Retrieved 19 March 2023. "The general problem with farming — especially plough agriculture — is that it involves so much intensive labor. One form of agriculture, however, eliminates most of this labor: 'flood-retreat' (also known as décrue or recession) agriculture. In flood-retreat agriculture, seeds are generally broadcast on the fertile silt deposited by an annual riverine flood."
- ↑ Graeber, David; Wengrow, David (2021). The dawn of everything: a new history of humanity. London: Allen Lane. p. 235. ISBN 978-0-241-40242-9.
- ↑ Maeda, Osamu; Lucas, Leilani; Silva, Fabio; Tanno, Ken-Ichi; Fuller, Dorian Q. (2016-08-01). "Narrowing the Harvest: Increasing sickle investment and the rise of domesticated cereal agriculture in the Fertile Crescent". Quaternary Science Reviews 145: 226–237. doi:10.1016/j.quascirev.2016.05.032. Bibcode: 2016QSRv..145..226M.
- ↑ 37.0 37.1 Weide, Alexander (29 November 2021). "Towards a Socio-Economic Model for Southwest Asian Cereal Domestication". Agronomy 11 (12): 2432. doi:10.3390/agronomy11122432.
- ↑ Dubreuil, Laure (2004-11-01). "Long-term trends in Natufian subsistence: a use-wear analysis of ground stone tools". Journal of Archaeological Science 31 (11): 1613–1629. doi:10.1016/j.jas.2004.04.003. Bibcode: 2004JArSc..31.1613D. https://www.sciencedirect.com/science/article/pii/S0305440304000731.
- ↑ Arranz-Otaegui, Amaia; Gonzalez Carretero, Lara; Ramsey, Monica N.; Fuller, Dorian Q.; Richter, Tobias (2018-07-31). "Archaeobotanical evidence reveals the origins of bread 14,400 years ago in northeastern Jordan". Proceedings of the National Academy of Sciences 115 (31): 7925–7930. doi:10.1073/pnas.1801071115. PMID 30012614. Bibcode: 2018PNAS..115.7925A.
- ↑ González Carretero, Lara; Wollstonecroft, Michèle; Fuller, Dorian Q. (2017-07-01). "A methodological approach to the study of archaeological cereal meals: a case study at Çatalhöyük East (Turkey)". Vegetation History and Archaeobotany 26 (4): 415–432. doi:10.1007/s00334-017-0602-6. PMID 28706348. PMC 5486841. Bibcode: 2017VegHA..26..415G. https://doi.org/10.1007/s00334-017-0602-6.
- ↑ Fuller, Dorian Q.; Carretero, Lara Gonzalez (2018-12-05). "The Archaeology of Neolithic Cooking Traditions: Archaeobotanical Approaches to Baking, Boiling and Fermenting". Archaeology International 21: 109–121. doi:10.5334/ai-391.
- ↑ Graeber, David; Wengrow, David (2021). The dawn of everything: a new history of humanity. London: Allen Lane. pp. 232. ISBN 978-0-241-40242-9.
- ↑ Vigne, Jean-Denis; Briois, François; Zazzo, Antoine; Willcox, George; Cucchi, Thomas; Thiébault, Stéphanie; Carrère, Isabelle; Franel, Yodrik et al. (2012-05-29). "First wave of cultivators spread to Cyprus at least 10,600 y ago". Proceedings of the National Academy of Sciences 109 (22): 8445–8449. doi:10.1073/pnas.1201693109. PMID 22566638. Bibcode: 2012PNAS..109.8445V.
- ↑ Lucas, Leilani; Colledge, Sue; Simmons, Alan; Fuller, Dorian Q. (2012-03-01). "Crop introduction and accelerated island evolution: archaeobotanical evidence from 'Ais Yiorkis and Pre-Pottery Neolithic Cyprus". Vegetation History and Archaeobotany 21 (2): 117–129. doi:10.1007/s00334-011-0323-1. Bibcode: 2012VegHA..21..117L. https://doi.org/10.1007/s00334-011-0323-1.
- ↑ Diamond, Jared (2005). Guns, Germs and Steel. Vintage. p. 97. ISBN 978-0-099-30278-0.
- ↑ Direct quotation: Grundas, S.T.: Chapter: "Wheat: The Crop", in Encyclopedia of Food Sciences and Nutrition p. 6130, 2003; Elsevier Science
- ↑ Piotrowski, Jan (26 February 2019). "Britons may have imported wheat long before farming it". https://www.newscientist.com/article/dn27044-britons-may-have-imported-wheat-long-before-farming-it/.
- ↑ Smith, Oliver; Momber, Garry; Bates, Richard et al. (2015). "Sedimentary DNA from a submerged site reveals wheat in the British Isles 8000 years ago". Science 347 (6225): 998–1001. doi:10.1126/science.1261278. PMID 25722413. Bibcode: 2015Sci...347..998S.
- ↑ Brace, Selina; Diekmann, Yoan; Booth, Thomas J.; van Dorp, Lucy; Faltyskova, Zuzana et al. (2019). "Ancient genomes indicate population replacement in Early Neolithic Britain". Nature Ecology & Evolution 3 (5): 765–771. doi:10.1038/s41559-019-0871-9. PMID 30988490. Bibcode: 2019NatEE...3..765B. "Neolithic cultures first appear in Britain circa 4000 bc, a millennium after they appeared in adjacent areas of continental Europe.".
- ↑ Long, Tengwen; Leipe, Christian; Jin, Guiyun; Wagner, Mayke; Guo, Rongzhen et al. (2018). "The early history of wheat in China from 14C dating and Bayesian chronological modelling". Nature Plants 4 (5): 272–279. doi:10.1038/s41477-018-0141-x. PMID 29725102.
- ↑ Bilgic, Hatice (2016). "Ancient DNA from 8400 Year-Old Çatalhöyük Wheat: Implications for the Origin of Neolithic Agriculture". PLOS One 11 (3): e0151974. doi:10.1371/journal.pone.0151974. PMID 26998604. Bibcode: 2016PLoSO..1151974B.
- ↑ "The science in detail – Wheats DNA – Research – Archaeology". The University of Sheffield. 19 July 2011. http://www.sheffield.ac.uk/archaeology/research/wheat/wheat2.
- ↑ Belderok, B. (2000). Bread-Making Quality of Wheat. Springer. p. 3. ISBN 0-7923-6383-3.
- ↑ Cauvain, S.P.; Cauvain, P. (2003). Bread Making. CRC Press. p. 540. ISBN 1-85573-553-9.
- ↑ Otter, Chris (2020). Diet for a large planet. University of Chicago Press. p. 50. ISBN 978-0-226-69710-9.
- ↑ Nelson, Scott Reynolds (2022). Oceans of Grain: How American Wheat Remade the World. Basic Books. pp. 3–4. ISBN 978-1-5416-4646-9.
- ↑ 57.0 57.1 57.2 57.3 Golovnina, K. A.; Glushkov, S. A.; Blinov, A. G.; Mayorov, V. I.; Adkison, L. R.; Goncharov, N. P. (2007-02-12). "Molecular phylogeny of the genus Triticum L". Plant Systematics and Evolution (Springer) 264 (3–4): 195–216. doi:10.1007/s00606-006-0478-x. Bibcode: 2007PSyEv.264..195G.
- ↑ 58.0 58.1 Belderok, Robert 'Bob'; Mesdag, Hans; Donner, Dingena A. (2000). Bread-Making Quality of Wheat. Springer. p. 3. ISBN 978-0-7923-6383-5.
- ↑ Friebe, B.; Qi, L.L.; Nasuda, S.; Zhang, P.; Tuleen, N.A.; Gill, B.S. (July 2000). "Development of a complete set of Triticum aestivum-Aegilops speltoides chromosome addition lines". Theoretical and Applied Genetics 101 (1): 51–58. doi:10.1007/s001220051448.
- ↑ Dvorak, Jan; Deal, Karin R.; Luo, Ming-Cheng; You, Frank M.; von Borstel, Keith; Dehghani, Hamid (2012-05-01). "The Origin of Spelt and Free-Threshing Hexaploid Wheat". Journal of Heredity 103 (3): 426–441. doi:10.1093/jhered/esr152. PMID 22378960.
- ↑ Shewry, P. R. (2009-04-01). "Wheat". Journal of Experimental Botany 60 (6): 1537–1553. doi:10.1093/jxb/erp058. ISSN 0022-0957. PMID 19386614.
- ↑ Fuller, Dorian Q.; Lucas, Leilani (2014), Wheats: Origins and Development, Springer New York, pp. 7812–7817, doi:10.1007/978-1-4419-0465-2_2192, ISBN 9781441904263
- ↑ 63.0 63.1 Yang, Fan; Zhang, Jingjuan; Liu, Qier et al. (2022-02-17). "Improvement and Re-Evolution of Tetraploid Wheat for Global Environmental Challenge and Diversity Consumption Demand". International Journal of Molecular Sciences 23 (4): 2206. doi:10.3390/ijms23042206. PMID 35216323.
- ↑ Smithers, Rebecca (15 May 2014). "Spelt flour 'wonder grain' set for a price hike as supplies run low". The Guardian. https://www.theguardian.com/money/2014/may/15/spelt-grain-supplies-under-pressure-high-demand.
- ↑ {{citation | mode = cs1 | title = Triticum turgidum subsp. dicoccon | work = Germplasm Resources Information Network (GRIN) | url = https://npgsweb.ars-grin.gov/gringlobal/taxonomydetail.aspx?314587 | publisher = [[Organization:Agricultural Research ServAgricultural Research Service (ARS), United States Department of Agriculture (USDA) | access-date = 11 December 2017 }}
- ↑ Khlestkina, Elena K.; Röder, Marion S.; Grausgruber, Heinrich; Börner, Andreas (2006). "A DNA fingerprinting-based taxonomic allocation of Kamut wheat". Plant Genetic Resources 4 (3): 172–180. doi:10.1079/PGR2006120.
- ↑ Anderson, Patricia C. (1991). "Harvesting of Wild Cereals During the Natufian as seen from Experimental Cultivation and Harvest of Wild Einkorn Wheat and Microwear Analysis of Stone Tools". in Bar-Yosef, Ofer. Natufian Culture in the Levant. International Monographs in Prehistory. Ann Arbor, Michigan: Berghahn Books. p. 523.
- ↑ 68.0 68.1 Potts, D.T. (1996) Mesopotamia Civilization: The Material Foundations Cornell University Press. p. 62. ISBN:0-8014-3339-8.
- ↑ Nevo, Eviatar & A.B. Korol & A. Beiles & T. Fahima. (2002) Evolution of Wild Emmer and Wheat Improvement: Population Genetics, Genetic Resources, and Genome.... Springer. p. 8. ISBN:3-540-41750-8.
- ↑ Vaughan, J.G. & P.A. Judd. (2003) The Oxford Book of Health Foods. Oxford University Press. p. 35. ISBN:0-19-850459-4.
- ↑ "Field Crop Information - College of Agriculture and Bioresources | University of Saskatchewan". https://agbio.usask.ca/cdc/field-crop-information.php.
- ↑ Bridgwater, W. & Beatrice Aldrich. (1966) "Wheat". The Columbia-Viking Desk Encyclopedia. Columbia University. p. 1959.
- ↑ 73.0 73.1 73.2 73.3 73.4 73.5 73.6 "Flour types: Wheat, Rye, and Barley". The New York Times. 18 February 1981. https://www.nytimes.com/1981/02/18/garden/flour-types-wheat-rye-and-barley.html.
- ↑ 74.0 74.1 74.2 74.3 74.4 74.5 74.6 "Wheat: Background". USDA. http://www.ers.usda.gov/topics/crops/wheat/background.aspx.
- ↑ Moon, David (2008). "In the Russian Steppes: the Introduction of Russian Wheat on the Great Plains of the UNited States". Journal of Global History 3 (2): 203–225. doi:10.1017/s1740022808002611.
- ↑ "Marquis Wheat". http://www.thecanadianencyclopedia.ca/en/article/marquis-wheat/.
- ↑ Mauseth, James D. (2014). Botany. Jones & Bartlett Publishers. p. 223. ISBN 978-1-4496-4884-8. https://books.google.com/books?id=0BGEs95p5EsC&pg=PA223. "Perhaps the simplest of fruits are those of grasses (all cereals such as corn and wheat)...These fruits are caryopses."
- ↑ Ensminger, Marion; Ensminger, Audrey H. Eugene (1993). Foods & Nutrition Encyclopedia, Two Volume Set. CRC Press. p. 164. ISBN 978-0-8493-8980-1. https://books.google.com/books?id=XMA9gYIj-C4C&pg=PA164.
- ↑ "Wheat". https://foodallergycanada.ca/about-allergies/food-allergens/wheat/.
- ↑ 80.0 80.1 80.2 Shewry, P. R.; Halford, N. G.; Belton, P. S.; Tatham, A. S. (2002). "The structure and properties of gluten: An elastic protein from wheat grain". Philosophical Transactions of the Royal Society B: Biological Sciences 357 (1418): 133–42. doi:10.1098/rstb.2001.1024. PMID 11911770.
- ↑ 81.0 81.1 "Whole Grain Fact Sheet". European Food Information Council. 1 January 2009. http://www.eufic.org/article/en/expid/whole-grain-fact-sheet/.
- ↑ 82.0 82.1 82.2 82.3 82.4 82.5 82.6 Shewry, Peter R.; Hey, S. J. (2015). "Review: The contribution of wheat to human diet and health". Food and Energy Security 4 (3): 178–202. doi:10.1002/fes3.64. PMID 27610232.
- ↑ European Community, Community Research and Development Information Service (24 February 2016). "Genetic markers signal increased crop productivity potential". http://cordis.europa.eu/news/rcn/124823_en.html.
- ↑ Dietary protein quality evaluation in human nutrition. Food and Agriculture Organization of the United Nations. 2013. ISBN 978-92-5-107417-6. http://www.fao.org/ag/humannutrition/35978-02317b979a686a57aa4593304ffc17f06.pdf. Retrieved 1 June 2017.
- ↑ Wolfe, R. R. (August 2015). "Update on protein intake: importance of milk proteins for health status of the elderly". Nutrition Reviews 73 (Suppl 1): 41–47. doi:10.1093/nutrit/nuv021. PMID 26175489.
- ↑ Shewry, Peter R.. "Impacts of agriculture on human health and nutrition – Vol. II – Improving the Protein Content and Quality of Temperate Cereals: Wheat, Barley and Rye". UNESCO – Encyclopedia Life Support Systems (UNESCO-EOLSS). http://www.eolss.net/sample-chapters/c10/E5-21-04-04.pdf. "When compared with the WHO requirements of essential amino acids for humans, wheat, barley and rye are seen to be deficient in lysine, with threonine being the second limiting amino acid (Table 1)."
- ↑ Vasal, S. K.. "The role of high lysine cereals in animal and human nutrition in Asia". Food and Agriculture Organization of the United Nations. http://www.fao.org/docrep/007/y5019e/y5019e0b.htm.
- ↑ "Nutritional quality of cereals". Food and Agriculture Organization of the United Nations. http://www.fao.org/docrep/x2184e/x2184e05.htm.
- ↑ Shewry, Peter R. (2009). "Wheat". Journal of Experimental Botany 60 (6): 1537–53. doi:10.1093/jxb/erp058. PMID 19386614.
- ↑ "Whole Grain Resource for the National School Lunch and School Breakfast Programs: A Guide to Meeting the Whole Grain-Rich criteria". US Department of Agriculture, Food and Nutrition Service. January 2014. http://www.fns.usda.gov/sites/default/files/WholeGrainResource.pdf. "Additionally, menu planners are encouraged to serve a variety of foods that meet whole grain-rich criteria and may not serve the same product every day to count for the HUSSC whole grain-rich criteria."
- ↑ "All About the Grains Group". US Department of Agriculture, MyPlate. 2016. https://www.choosemyplate.gov/grains.
- ↑ 92.0 92.1 92.2 92.3 "Celiac disease". World Gastroenterology Organisation Global Guidelines. July 2016. http://www.worldgastroenterology.org/guidelines/global-guidelines/celiac-disease/celiac-disease-english.
- ↑ 93.0 93.1 93.2 "Definition and Facts for Celiac Disease". The National Institute of Diabetes and Digestive and Kidney Diseases, National Institutes of Health, US Department of Health and Human Services, Bethesda, MD. 2016. https://www.niddk.nih.gov/health-information/health-topics/digestive-diseases/celiac-disease/Pages/definition-facts.aspx.
- ↑ 94.0 94.1 Ludvigsson, Jonas F.; Leffler, Daniel A.; Bai, Julio C.; Biagi, Federico; Fasano, Alessio et al. (2012-02-16). "The Oslo definitions for coeliac disease and related terms". Gut (BMJ) 62 (1): 43–52. doi:10.1136/gutjnl-2011-301346. PMID 22345659.
- ↑ Molina-Infante, J.; Santolaria, S.; Sanders, D. S.; Fernández-Bañares, F. (May 2015). "Systematic review: noncoeliac gluten sensitivity". Alimentary Pharmacology & Therapeutics 41 (9): 807–820. doi:10.1111/apt.13155. PMID 25753138.
- ↑ Volta, Umberto; De Giorgio, Roberto; Caio, Giacomo; Uhde, Melanie; Manfredini, Roberto; Alaedini, Armin (2019). "Nonceliac Wheat Sensitivity". Gastroenterology Clinics of North America 48 (1): 165–182. doi:10.1016/j.gtc.2018.09.012. PMID 30711208.
- ↑ 97.0 97.1 97.2 Verbeke, K. (February 2018). "Nonceliac Gluten Sensitivity: What Is the Culprit?". Gastroenterology 154 (3): 471–473. doi:10.1053/j.gastro.2018.01.013. PMID 29337156.
- ↑ 98.0 98.1 Fasano, Alessio; Sapone, Anna; Zevallos, Victor; Schuppan, Detlef (2015). "Nonceliac Gluten Sensitivity". Gastroenterology 148 (6): 1195–1204. doi:10.1053/j.gastro.2014.12.049. PMID 25583468.
- ↑ Barone, Maria; Troncone, Riccardo; Auricchio, Salvatore (2014). "Gliadin Peptides as Triggers of the Proliferative and Stress/Innate Immune Response of the Celiac Small Intestinal Mucosa". International Journal of Molecular Sciences 15 (11): 20518–20537. doi:10.3390/ijms151120518. PMID 25387079.
- ↑ 100.0 100.1 "Wheat production in 2021 from pick lists: Crops/World regions/Production quantity/Year". UN Food and Agriculture Organization, Statistics Division, FAOSTAT. 2023. http://www.fao.org/faostat/en/#data/QC.
- ↑ World Food and Agriculture – Statistical Yearbook 2021. Rome: FAO. 2021. doi:10.4060/cb4477en. ISBN 978-92-5-134332-6. https://doi.org/10.4060/cb4477en.
- ↑ "Crops and livestock products". UN Food and Agriculture Organization, Statistics Division, FAOSTAT. 2021. http://www.fao.org/faostat/en/#data/TP.
- ↑ "Wheat area harvested, world total from pick lists: Crops/World regions/Area harvested/Year". 2023. http://www.fao.org/faostat/en/#data/QC.
- ↑ Curtis; Rajaraman; MacPherson (2002). "Bread Wheat". Food and Agriculture Organization of the United Nations. http://www.fao.org/docrep/006/y4011e/y4011e00.htm.
- ↑ Day, L.; Augustin, M.A.; Batey, I.L.; Wrigley, C.W. (2006). "Wheat-gluten uses and industry needs". Trends in Food Science & Technology (Elsevier) 17 (2): 82–90. doi:10.1016/j.tifs.2005.10.003.
- ↑ "Wheat prices in England". https://ourworldindata.org/grapher/wheat-prices-in-england.
- ↑ Palmer, Alan (1996). Dictionary of the British Empire and Commonwealth. pp. 193, 320, 338.
- ↑ Ridley, Annie E. (1904). A Backward Glance: The Story of John Ridley, a Pioneer. J. Clarke. p. 21. https://books.google.com/books?id=B44WAAAAYAAJ&dq=john+Ridley+australia+wheat&pg=PP21.
- ↑ Furtan, W. Hartley; Lee, George E. (1977). "Economic Development of the Saskatchewan Wheat Economy". Canadian Journal of Agricultural Economics 25 (3): 15–28. doi:10.1111/j.1744-7976.1977.tb02882.x. Bibcode: 1977CaJAE..25...15F. https://onlinelibrary.wiley.com/doi/10.1111/j.1744-7976.1977.tb02882.x.
- ↑ Joshi, A. K.; Mishra, B.; Chatrath, R.; Ortiz Ferrara, G.; Singh, Ravi P. (2007). "Wheat improvement in India: present status, emerging challenges and future prospects". Euphytica 157 (3): 431–446. doi:10.1007/s10681-007-9385-7. https://link.springer.com/10.1007/s10681-007-9385-7.
- ↑ Otter, Chris (2020). Diet for a large planet. USA: University of Chicago Press. p. 51. ISBN 978-0-226-69710-9.
- ↑ Otter, Chris (2020). Diet for a large planet. USA: University of Chicago Press. p. 69. ISBN 978-0-226-69710-9.
- ↑ Slafer, G.A.; Satorre, E.H. (1999). "Chapter 1". Wheat: Ecology and Physiology of Yield Determination. Haworth Press. ISBN 1-56022-874-1.
- ↑ Wright, B. D.; Pardey, P. G. (2002). "Agricultural R&D, productivity, and global food prospects". Plants, Genes and Crop Biotechnology. Jones & Bartlett Learning. pp. 22-51. ISBN 9780763715861. https://books.google.com/books?id=UF94McWtLP0C&pg=PA22.
- ↑ Asseng, S.; Ewert, F.; Martre, P.; Rötter, R. P.; Lobell, D. B. et al. (2015). "Rising temperatures reduce global wheat production". Nature Climate Change 5 (2): 143–147. doi:10.1038/nclimate2470. Bibcode: 2015NatCC...5..143A. http://eprints.whiterose.ac.uk/85540/1/Main_Asseng_2014-9-22.pdf.
- ↑ 116.0 116.1 "How To Grow Wheat Efficiently On A Large Farm". 10 May 2023. https://eos.com/blog/growing-wheat/.
- ↑ Slafer, G.A.; Satorre, E.H. (1999). Wheat: Ecology and Physiology of Yield Determination. Haworth Press. pp. 322–323. ISBN 1-56022-874-1.
- ↑ Saini, H.S.; Sedgley, M.; Aspinall, D. (1984). "Effect of heat stress during floral development on pollen tube growth and ovary anatomy in wheat (Triticum aestivum L.)". Australian Journal of Plant Physiology 10 (2): 137–144. doi:10.1071/PP9830137.
- ↑ Overton, Mark (1996). Agricultural Revolution in England: The transformation of the agrarian economy 1500-1850. Cambridge University Press. p. 1, and throughout. ISBN 978-0-521-56859-3. https://archive.org/details/isbn_9780521568593.
- ↑ Caprettini, Bruno; Voth, Hans-Joachim (2020). "Rage against the Machines: Labor-Saving Technology and Unrest in Industrializing England". American Economic Review: Insights 2 (3): 305–320. doi:10.1257/aeri.20190385.
- ↑ Constable, George; Somerville, Bob (2003). A Century of Innovation: Twenty Engineering Achievements That Transformed Our Lives, Chapter 7, Agricultural Mechanization. Washington, DC: Joseph Henry Press. ISBN 0-309-08908-5. http://www.greatachievements.org/?id=2955.
- ↑ Borojevic, Katarina; Borojevic, Ksenija (July–August 2005). "The Transfer and History of "Reduced Height Genes" (Rht) in Wheat from Japan to Europe". Journal of Heredity (Oxford University Press) 96 (4): 455–459. doi:10.1093/jhered/esi060. PMID 15829727.
- ↑ Shindler, Miriam (3 January 2016). "From east Asia to south Asia, via Mexico: how one gene changed the course of history". https://www.cimmyt.org/news/from-east-asia-to-south-asia-via-mexico-how-one-gene-changed-the-course-of-history/.
- ↑ Brown, L. R. (30 October 1970). "Nobel Peace Prize: developer of high-yield wheat receives award (Norman Ernest Borlaug)". Science 170 (957): 518–519. doi:10.1126/science.170.3957.518. PMID 4918766.
- ↑ Basavaraja, H.; Mahajanashetti, S.B.; Udagatti, N.C. (2007). "Economic Analysis of Post-harvest Losses in Food Grains in India: A Case Study of Karnataka". Agricultural Economics Research Review 20: 117–126. http://ageconsearch.umn.edu/bitstream/47429/2/8.pdf.
- ↑ Godfray, H.C.; Beddington, J. R.; Crute, I. R.; Haddad, L.; Lawrence, D. et al. (2010). "Food security: The challenge of feeding 9 billion people". Science 327 (5967): 812–818. doi:10.1126/science.1185383. PMID 20110467. Bibcode: 2010Sci...327..812G.
- ↑ Swaminathan, M. S. (2004). "Stocktake on cropping and crop science for a diverse planet". Proceedings of the 4th International Crop Science Congress, Brisbane, Australia. http://www.cropscience.org.au/icsc2004/plenary/0/2159_swaminathan.htm.
- ↑ "Umbers, Alan (2006, Grains Council of Australia Limited) Grains Industry trends in Production – Results from Today's Farming Practices". http://www.grainscouncil.com/EMS/06_Nov_02_Production_Farming_Practices.pdf.
- ↑ "Pest Management". 7 March 2018. http://www.agronomy.org/about-agronomy/pest-management/.
- ↑ Savary, Serge; Willocquet, Laetitia; Pethybridge, Sarah Jane; Esker, Paul; McRoberts, Neil; Nelson, Andy (4 February 2019). "The global burden of pathogens and pests on major food crops". Nature Ecology & Evolution (Springer Science and Business Media LLC) 3 (3): 430–439. doi:10.1038/s41559-018-0793-y. PMID 30718852. Bibcode: 2019NatEE...3..430S. https://research.utwente.nl/en/publications/cd23339a-cd2a-4075-b093-e74a05f71d98.
- ↑ Abhishek, Aditya (11 January 2021). "Disease of Wheat: Get To Know Everything About Wheat Diseases". https://agriculturereview.com/2021/01/disease-of-wheat-symptoms-and-management.html.
- ↑ "G4319 Wheat Diseases in Missouri, MU Extension". University of Missouri Extension. http://muextension.missouri.edu/explore/agguides/crops/g04319.htm.
- ↑ C.Michael Hogan. 2013. Wheat. Encyclopedia of Earth, National Council for Science and the Environment, Washington DC ed. P. Saundry
- ↑ 134.0 134.1 134.2 134.3 Singh, Jagdeep; Chhabra, Bhavit; Raza, Ali; Yang, Seung Hwan; Sandhu, Karansher S. (2023). "Important wheat diseases in the US and their management in the 21st century". Frontiers in Plant Science 13. doi:10.3389/fpls.2022.1010191. PMID 36714765.
- ↑ Gautam, P.; Dill-Macky, R. (2012). "Impact of moisture, host genetics and Fusarium graminearum isolates on Fusarium head blight development and trichothecene accumulation in spring wheat". Mycotoxin Research 28 (1): 45–58. doi:10.1007/s12550-011-0115-6. PMID 23605982.
- ↑ Singh, Ravi P.; Hodson, David; Huerta-Espino, Julio; Jin, Yue; Njau, Peter et al. (2008). Will Stem Rust Destroy the World's Wheat Crop?. Advances in Agronomy. 98. pp. 272–309. doi:10.1016/S0065-2113(08)00205-8. ISBN 9780123743558. https://naldc-legacy.nal.usda.gov/naldc/download.xhtml?id=36520&content=PDF.
- ↑ 137.0 137.1 Kumar, Sudheer; Kashyap, Prem; Singh, Gyanendra (2020). Wheat Blast (1 ed.). Boca Raton, Florida: CRC Press. p. 70. doi:10.1201/9780429470554. ISBN 978-0-429-47055-4. OCLC 1150902336.
- ↑ Harveson, Bob (2017-08-17). "Has Ergot Altered Events in World History?". unl.edu. https://cropwatch.unl.edu/2017/has-ergot-altered-events-world-history.
- ↑ Cárcamo, Héctor; Entz, Toby; Beres, Brian (2007). "Estimating Cephus cinctus wheat stem cutting damage – can we cut stem counts?". Journal of Agricultural and Urban Entomology 24 (3): 117–124. doi:10.3954/1523-5475-24.3.117. http://www.bioone.org/doi/abs/10.3954/1523-5475-24.3.117?journalCode=jaue.
- ↑ Biological Control of Stored-Product Pests. Biological Control News Volume II, Number 10 October 1995
- ↑ CSIRO Rodent Management Research Focus: Mice plagues
- ↑ "ARS, Industry Cooperation Yields Device to Detect Insects in Stored Wheat". USDA Agricultural Research Service. 24 June 2010. http://www.ars.usda.gov/is/pr/2010/100624.htm.
- ↑ 143.0 143.1 Bajaj, Y.P.S. (1990) Wheat. Springer Science+Business Media. pp. 161–163. ISBN:3-540-51809-6.
- ↑ "Mutant variety database". http://mvgs.iaea.org/.
- ↑ Verma, Shailender Kumar; Kumar, Satish; Sheikh, Imran et al. (3 March 2016). "Transfer of useful variability of high grain iron and zinc from Aegilops kotschyi into wheat through seed irradiation approach". International Journal of Radiation Biology 92 (3): 132–139. doi:10.3109/09553002.2016.1135263. PMID 26883304.
- ↑ MacNeil, Marcia (20 January 2021). "CIMMYT scientist Ravi Singh receives prestigious award from the Government of India". http://www.cimmyt.org/news/cimmyt-scientist-ravi-singh-receives-prestigious-award-from-the-government-of-india/.
- ↑ "Press Release: ICARDA safeguards world heritage of genetic resources during the conflict in Syria". http://www.icarda.org/media/news/press-release-icarda-safeguards-world-heritage-genetic-resources-during-conflict-syria.
- ↑ 148.0 148.1 148.2 Fabre, Frederic; Burie, Jean-Baptiste; Ducrot, Arnaud; Lion, Sebastien; Richard, Quentin; Demasse, Ramses (2022). "An epi-evolutionary model for predicting the adaptation of spore-producing pathogens to quantitative resistance in heterogeneous environments". Evolutionary Applications (John Wiley & Sons) 15 (1): 95–110. doi:10.1111/eva.13328. PMID 35126650.
- ↑ Würschum, Tobias; Langer, Simon M.; Longin, C. Friedrich H.; Tucker, Matthew R.; Leiser, Willmar L. (2017-09-26). "A modern Green Revolution gene for reduced height in wheat". The Plant Journal 92 (5): 892–903. doi:10.1111/tpj.13726. PMID 28949040.
- ↑ Kulshrestha, V. P.; Tsunoda, S. (1981-03-01). "The role of 'Norin 10' dwarfing genes in photosynthetic and respiratory activity of wheat leaves". Theoretical and Applied Genetics 60 (2): 81–84. doi:10.1007/BF00282421. PMID 24276628. https://doi.org/10.1007/BF00282421.
- ↑ Milach, S. C. K.; Federizzi, L. C. (2001-01-01). Dwarfing genes in plant improvement. Advances in Agronomy. 73. Academic Press. pp. 35–63. doi:10.1016/S0065-2113(01)73004-0. ISBN 9780120007738.
- ↑ Lupton, F. G. H.; Oliver, R. H.; Ruckenbauer, P. (2009-03-27). "An analysis of the factors determining yields in crosses between semi-dwarf and taller wheat varieties". The Journal of Agricultural Science 82 (3): 483–496. doi:10.1017/S0021859600051388.
- ↑ 153.0 153.1 Adamski, Nikolai M.; Simmonds, James; Brinton, Jemima F. et al. (2021-05-01). "Ectopic expression of Triticum polonicum VRT-A2 underlies elongated glumes and grains in hexaploid wheat in a dosage-dependent manner". The Plant Cell (Oxford University Press) 33 (7): 2296–2319. doi:10.1093/plcell/koab119. PMID 34009390.
- ↑ "Guinness World Records – Highest Wheat Yield". 10 August 2022. http://www.guinnessworldrecords.com/world-records/highest-wheat-yield.
- ↑ Farmers Weekly (23 November 2018). "Lincs grower scoops top wheat and rapeseed yield awards". https://www.fwi.co.uk/arable/crop-management/lincs-grower-scoops-top-wheat-and-rapeseed-yield-awards.
- ↑ "Agricultural and Horticultural Development Board – 2018 GB Harvest Progress Results". https://cereals.ahdb.org.uk/markets/market-news/2018/september/28/gb-harvest-progress-2018-report-6.aspx.
- ↑ Hoisington, D.; Khairallah, M.; Reeves, T. et al. (1999). "Plant genetic resources: What can they contribute toward increased crop productivity?". Proceedings of the National Academy of Sciences 96 (11): 5937–43. doi:10.1073/pnas.96.11.5937. PMID 10339521. Bibcode: 1999PNAS...96.5937H.
- ↑ Dahm, Madeline (27 July 2021). "Genome-wide association study puts tan spot-resistant genes in the spotlight". http://wheat.org/genome-wide-association-study-puts-tan-spot-resistant-genes-in-the-spotlight/.
- ↑ Bohra, Abhishek; Kilian, Benjamin; Sivasankar, Shoba; Caccamo, Mario; Mba, Chikelu; McCouch, Susan R.; Varshney, Rajeev K. (2021). "Reap the crop wild relatives for breeding future crops". Trends in Biotechnology (Cell Press) 40 (4): 412–431. doi:10.1016/j.tibtech.2021.08.009. PMID 34629170.
- ↑ Kourelis, Jiorgos; van der Hoorn, Renier A.L. (2018-01-30). "Defended to the Nines: 25 Years of Resistance Gene Cloning Identifies Nine Mechanisms for R Protein Function". The Plant Cell (American Society of Plant Biologists (OUP)) 30 (2): 285–299. doi:10.1105/tpc.17.00579. PMID 29382771.
- ↑ 161.0 161.1 Dodds, Peter N.; Rathjen, John P. (2010-06-29). "Plant immunity: towards an integrated view of plant–pathogen interactions". Nature Reviews Genetics (Nature Portfolio) 11 (8): 539–548. doi:10.1038/nrg2812. PMID 20585331.
- ↑ 162.0 162.1 Furbank, Robert T.; Tester, Mark (2011). "Phenomics – technologies to relieve the phenotyping bottleneck". Trends in Plant Science (Cell Press) 16 (12): 635–644. doi:10.1016/j.tplants.2011.09.005. PMID 22074787.
- ↑ 163.0 163.1 Herrera, Leonardo; Gustavsson, Larisa; Åhman, Inger (2017). "A systematic review of rye (Secale cereale L.) as a source of resistance to pathogens and pests in wheat (Triticum aestivum L.)". Hereditas (BioMed Central) 154 (1): 1–9. doi:10.1186/s41065-017-0033-5. PMID 28559761.
- ↑ Kaur, Bhavjot; Mavi, G. S.; Gill, Manpartik S.; Saini, Dinesh Kumar (2020-07-02). "Utilization of KASP technology for wheat improvement". Cereal Research Communications (Springer Science+Business Media) 48 (4): 409–421. doi:10.1007/s42976-020-00057-6.
- ↑ Mike Abram for Farmers' Weekly. 17 May 2011. Hybrid wheat to make a return
- ↑ Bill Spiegel for agriculture.com 11 March 2013 Hybrid wheat's comeback
- ↑ "The Hybrid wheat website". 18 December 2013. http://www.hybridwheat.net/anglais/growing-hybrid-wheat-in-europe/history-of-hybrid-wheat/history-of-hybrid-wheat-627.aspx.
- ↑ Basra, Amarjit S. (1999) Heterosis and Hybrid Seed Production in Agronomic Crops. Haworth Press. pp. 81–82. ISBN:1-56022-876-8.
- ↑ Aberkane, Hafid; Payne, Thomas; Kishi, Masahiro; Smale, Melinda; Amri, Ahmed; Jamora, Nelissa (1 October 2020). "Transferring diversity of goat grass to farmers' fields through the development of synthetic hexaploid wheat". Food Security 12 (5): 1017–1033. doi:10.1007/s12571-020-01051-w.
- ↑ Kishii, Masahiro (9 May 2019). "An Update of Recent Use of Aegilops Species in Wheat Breeding". Frontiers in Plant Science (Frontiers Media SA) 10: 585. doi:10.3389/fpls.2019.00585. PMID 31143197.
- ↑ (12 May 2013) Cambridge-based scientists develop 'superwheat' BBC News UK, Retrieved 25 May 2013
- ↑ Synthetic hexaploids
- ↑ (2013) Synthetic hexaploid wheat UK National Institute of Agricultural Botany, Retrieved 25 May 2013
- ↑ Belderok, B. (1 January 2000). "Developments in bread-making processes". Plant Foods for Human Nutrition (Dordrecht, Netherlands) 55 (1): 1–86. doi:10.1023/A:1008199314267. PMID 10823487.
- ↑ Delcour, J. A.; Joye, I. J.; Pareyt, B.; Wilderjans, E.; Brijs, K.; Lagrain, B. (2012). "Wheat gluten functionality as a quality determinant in cereal-based food products". Annual Review of Food Science and Technology 3: 469–492. doi:10.1146/annurev-food-022811-101303. PMID 22224557. https://www.researchgate.net/publication/221728752.
- ↑ Pronin, Darina; Borner, Andreas; Weber, Hans; Scherf, Ann (10 July 2020). "Wheat (Triticum aestivum L.) Breeding from 1891 to 2010 Contributed to Increasing Yield and Glutenin Contents but Decreasing Protein and Gliadin Contents". Journal of Agricultural and Food Chemistry 68 (46): 13247–13256. doi:10.1021/acs.jafc.0c02815. PMID 32648759.
- ↑ Condon, AG; Farquhar, GD; Richards, RA (1990). "Genotypic variation in carbon isotope discrimination and transpiration efficiency in wheat. Leaf gas exchange and whole plant studies". Australian Journal of Plant Physiology 17: 9–22. doi:10.1071/PP9900009.
- ↑ Kassa, Mulualem T.; Haas, Sabrina; Schliephake, Edgar et al. (9 May 2016). "A saturated SNP linkage map for the orange wheat blossom midge resistance gene Sm1". Theoretical and Applied Genetics (Springer Science and Business Media LLC) 129 (8): 1507–1517. doi:10.1007/s00122-016-2720-4. PMID 27160855.
- ↑ Ahmad, Reaz (26 November 2020). "New genome sequencing rekindles hope for fighting wheat blast". http://www.dhakatribune.com/bangladesh/agriculture/2020/11/26/new-genome-sequencing-rekindles-hope-for-fighting-wheat-blast.
- ↑ 180.0 180.1 "Landmark study generates first genomic atlas for global wheat improvement". University of Saskatchewan. 25 November 2020. http://news.usask.ca/articles/research/2020/landmark-study-generates-first-genomic-atlas-for-global-wheat-improvement.php.
- ↑ Walkowiak, Sean; Gao, Liangliang; Monat, Cecile; Haberer, Georg; Kassa, Mulualem T. et al. (25 November 2020). "Multiple wheat genomes reveal global variation in modern breeding". Nature (Nature Research/Springer Nature) 588 (7837): 277–283. doi:10.1038/s41586-020-2961-x. PMID 33239791. Bibcode: 2020Natur.588..277W.
- ↑ "UK researchers release draft sequence coverage of wheat genome". Biotechnology and Biological Sciences Research Council. 27 August 2010. http://www.bbsrc.ac.uk/news/food-security/2010/100827-pr-uk-researchers-release-draft-wheat-genome.aspx.
- ↑ "UK scientists publish draft sequence coverage of wheat genome". Biotechnology and Biological Sciences Research Council. http://www.bbsrc.ac.uk/web/FILES/Publications/1102_wheat_genome_case_study.pdf.
- ↑ 184.0 184.1 Hall (2012). "Analysis of the bread wheat genome using whole-genome shotgun sequencing". Nature 491 (7426): 705–10. doi:10.1038/nature11650. PMID 23192148. Bibcode: 2012Natur.491..705B.
- ↑ "U of S crop scientists help crack the wheat genome code". University of Saskatchewan, Canada. 16 August 2018. https://news.usask.ca/articles/research/2018/u-of-s-crop-scientists-help-crack-the-wheat-genome-code-.php.
- ↑ 186.0 186.1 186.2 186.3 186.4 186.5 186.6 Li, Shaoya; Zhang, Chen; Li, Jingying; Yan, Lei; Wang, Ning; Xia, Lanqin (2021). "Present and future prospects for wheat improvement through genome editing and advanced technologies". Plant Communications (Chinese Academy of Sciences, Center for Excellence in Molecular Plant Sciences and Chinese Society for Plant Biology (Cell Press)) 2 (4): 100211. doi:10.1016/j.xplc.2021.100211. PMID 34327324.
- ↑ Cézanne to Picasso: Ambroise Vollard, Patron of the Avant-garde. Metropolitan Museum of Art. 2006. p. 11. ISBN 1588391957. https://books.google.com/books?id=B6JTCCBSZuoC&pg=PA11.
- ↑ McKenna, Tony (2015). Art, Literature and Culture from a Marxist Perspective. Springer. PT101. ISBN 978-1137526618. https://books.google.com/books?id=cqWFCwAAQBAJ&pg=PT101.
- ↑ "Wheat". Smithsonian Institution. https://americanart.si.edu/artwork/wheat-32285.
- ↑ Hessel, Katy (18 July 2022). "A field of wheat on a $4.5bn patch of New York: the prophetic eco art of Agnes Denes". The Guardian. https://www.theguardian.com/artanddesign/2022/jul/18/a-field-of-wheat-on-a-45bn-patch-of-new-york-the-prophetic-eco-art-of-agnes-denes.
Further reading
- The World Wheat Book : A History of Wheat Breeding
- Bonjean, Alain P.; Angus, William J. (2001). The World Wheat Book : A History of Wheat Breeding. 1. London: Lavoisier. ISBN 9781898298724. OCLC 59515318.
- Bonjean, Alain P. (2011). The World Wheat Book : A History of Wheat Breeding. 2. Paris: Lavoisier. ISBN 978-2-7430-1102-4. OCLC 707171112.
- Bonjean, Alain P.; Angus, William J.; Ginkel, Maarten van (2016). The World Wheat Book : A History of Wheat Breeding. 3. Paris: Lavoisier-Tec & doc. ISBN 978-2-7430-2091-0. OCLC 953081390.
- Head, Lesley; Atchison, Jennifer; Gates, Alison (2016). Ingrained: A Human Bio-geography of Wheat. London: Ashgate Publishing. ISBN 978-1-315-58854-4. OCLC 1082225627.
- Jasny Naum, The Wheats of Classical Antiquity. Johns Hopkins University Press, Baltimore, 1944. S2CID:82345748.
- Nelson, Scott Reynolds (2022). Oceans of Grain: How American Wheat Remade the World. Excerpt.
- Shiferaw, Bekele; Smale, Melinda; Braun, Hans-Joachim; Duveiller, Etienne; Reynolds, Mathew; Muricho, Geoffrey (2013). "Crops that feed the world 10. Past successes and future challenges to the role played by wheat in global food security". Food Security 5 (3): 291–317. doi:10.1007/s12571-013-0263-y.
External links
Wikidata ☰ Q12106 entry
![]() | Original source: https://en.wikipedia.org/wiki/Wheat.
Read more |