Biology:Chorismate synthase
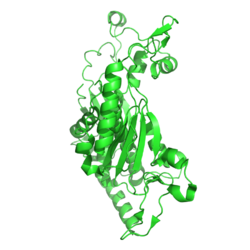
chorismate synthase | |||||||||
---|---|---|---|---|---|---|---|---|---|
![]() Chorismate synthase homotetramer, Streptococcus pneumoniae | |||||||||
Identifiers | |||||||||
EC number | 4.2.3.5 | ||||||||
CAS number | 9077-07-0 | ||||||||
Databases | |||||||||
IntEnz | IntEnz view | ||||||||
BRENDA | BRENDA entry | ||||||||
ExPASy | NiceZyme view | ||||||||
KEGG | KEGG entry | ||||||||
MetaCyc | metabolic pathway | ||||||||
PRIAM | profile | ||||||||
PDB structures | RCSB PDB PDBe PDBsum | ||||||||
Gene Ontology | AmiGO / QuickGO | ||||||||
|
The enzyme chorismate synthase (EC 4.2.3.5) catalyzes the chemical reaction
- 5-O-(1-carboxyvinyl)-3-phosphoshikimate [math]\displaystyle{ \rightleftharpoons }[/math] chorismate + phosphate
This enzyme belongs to the family of lyases, specifically those carbon-oxygen lyases acting on phosphates. The systematic name of this enzyme class is 5-O-(1-carboxyvinyl)-3-phosphoshikimate phosphate-lyase (chorismate-forming). This enzyme is also called 5-O-(1-carboxyvinyl)-3-phosphoshikimate phosphate-lyase. This enzyme participates in phenylalanine, tyrosine and tryptophan biosynthesis.
Chorismate synthase | |||||||||||
---|---|---|---|---|---|---|---|---|---|---|---|
Identifiers | |||||||||||
Symbol | Chorismate_synt | ||||||||||
Pfam | PF01264 | ||||||||||
InterPro | IPR000453 | ||||||||||
PROSITE | PDOC00628 | ||||||||||
SCOP2 | 1q1l / SCOPe / SUPFAM | ||||||||||
|
Chorismate synthase catalyzes the last of the seven steps in the shikimate pathway which is used in prokaryotes, fungi and plants for the biosynthesis of aromatic amino acids. It catalyzes the 1,4-trans elimination of the phosphate group from 5-enolpyruvylshikimate-3-phosphate (EPSP) to form chorismate which can then be used in phenylalanine, tyrosine or tryptophan biosynthesis. Chorismate synthase requires the presence of a reduced flavin mononucleotide (FMNH2 or FADH2) for its activity. Chorismate synthase from various sources shows[2][3] a high degree of sequence conservation. It is a protein of about 360 to 400 amino-acid residues.
Biological and practical function
The shikimate pathway synthesises precursors to aromatic amino acids, as well as other aromatic compounds that have various involvement with processes such as "UV protection, electron transport, signaling, communication, plant defense, and the wound response".[4] Because humans lack the shikimate pathway, but it is required for the survival of many microorganisms, the pathway and chorismate synthase in particular are considered to be potential targets for new antimicrobial treatments. For example, chorismate synthase is known to be essential to the survival of Mycobacterium tuberculosis, making the enzyme an attractive antibiotic target for control of this pathogen.[5]
Structural studies
As of late 2007, 9 structures have been solved for this class of enzymes, with PDB accession codes 1Q1L, 1QXO, 1R52, 1R53, 1SQ1, 1UM0, 1UMF, 1ZTB, and 2G85.
The crystal structure of chorismate synthase is a homotetramer with one FMN molecule non-covalently bound to each of the four monomers. Each monomer is made up of 9 alpha helices and 18 beta strands and the core is assembled in a unique beta-alpha-beta sandwich fold. The active sites for FMN-binding are made up of clusters of flexible loops and the area around these regions have highly positive electromagnetic potential. There are two histidine residues located at the active site which are thought to protonate the reduced flavin molecule and the leaving phosphate group of the substrate.[6]
Mechanism
The formation of chorismate from EPSP involves two eliminations, of phosphate and a proton (H+), from the substrate. In the first step of catalysis, phosphate is eliminated, assisted by proton transfer from a conserved histidine residue. At the same time, an electron is transferred from the FMN to the substrate, forming an FMN radical and a substrate radical. Next, the FMN radical rearranges, and then a hydrogen atom is transferred to FMN from the substrate, eliminating both radicals and generating the product. The reduced FMN then re-tautomerizes to its active form by donating a proton to a second conserved histidine.[7] Although the chorismate synthase reaction is FMN-dependent, there is no net redox change between substrate and product; the FMN merely acts as a catalyst.
Two classes of chorismate synthase exist, differing in how the reduced state of the FMN cofactor is maintained. Bifunctional chorismate synthase is present in fungi and contains an NAD(P)H-dependent flavin reductase domain.[5] Monofunctional chorismate synthase is found in plants and E.coli and lacks a flavin reductase domain. It depends on a separate reductase enzyme to reduce the FMN.[5]
References
- ↑ 1ZTB Dias (2006). "Structure of chorismate synthase from Mycobacterium tuberculosis". Journal of Structural Biology 154 (2): 130–143. doi:10.1016/j.jsb.2005.12.008. PMID 16459102.; rendered with PyMOL
- ↑ "Molecular cloning and analysis of a cDNA coding for chorismate synthase from the higher plant Corydalis sempervirens Pers". J. Biol. Chem. 266 (32): 21434–21438. 1991. PMID 1718979.
- ↑ "Molecular cloning, characterization and analysis of the regulation of the ARO2 gene, encoding chorismate synthase, of Saccharomyces cerevisiae". Mol. Microbiol. 5 (9): 2143–2152. 1991. doi:10.1111/j.1365-2958.1991.tb02144.x. PMID 1837329.
- ↑ Macheroux, P.; Schmid, J. R.; Amrhein, N.; Schaller, A. (1999). "A unique reaction in a common pathway: Mechanism and function of chorismate synthase in the shikimate pathway". Planta 207 (3): 325–334. doi:10.1007/s004250050489. PMID 9951731.
- ↑ 5.0 5.1 5.2 Ely, F.; Nunes, J. E.; Schroeder, E. K.; Frazzon, J.; Palma, M. S.; Santos, D. S.; Basso, L. A. (2008). "The Mycobacterium tuberculosis Rv2540c DNA sequence encodes a bifunctional chorismate synthase". BMC Biochemistry 9: 13. doi:10.1186/1471-2091-9-13. PMID 18445278.
- ↑ Ahn, H. J.; Yoon, H. J.; Lee, B. I.; Suh, S. W. (2004). "Crystal Structure of Chorismate Synthase: A Novel FMN-binding Protein Fold and Functional Insights". Journal of Molecular Biology 336 (4): 903–915. doi:10.1016/j.jmb.2003.12.072. PMID 15095868. https://zenodo.org/record/891107.
- ↑ Kitzing, Karina; Auweter, Sigrid; Amrhein, Nikolaus; Macheroux, Peter (March 2004). "Mechanism of chorismate synthase: Role of the two invariant histidine residues in the active site". Journal of Biological Chemistry 279 (10): 9451-9461. doi:10.1074/jbc.M312471200. PMID 14668332. https://www.jbc.org/article/S0021-9258(17)47857-4/fulltext. Retrieved 20 Jan 2024.
- "Properties of chorismate synthase in Neurospora crassa". J. Biol. Chem. 248 (13): 4602–9. 1973. PMID 4146266.
- "The enzymic synthesis of chorismic and prephenic acids from 3-enolpyruvylshikimic acid 5-phosphate". J. Biol. Chem. 242 (1): 82–90. 1967. PMID 4289188.
- "Chorismate synthase of Neurospora crassa: a flavoprotein". Arch. Biochem. Biophys. 165 (2): 505–18. 1974. doi:10.1016/0003-9861(74)90276-8. PMID 4155270.
- "The transient kinetics of Escherichia coli chorismate synthase: substrate consumption, product formation, phosphate dissociation, and characterization of a flavin intermediate". Biochemistry 35 (30): 9907–16. 1996. doi:10.1021/bi952958q. PMID 8703965.
- "A Secondary beta Deuterium Kinetic Isotope Effect in the Chorismate Synthase Reaction". Bioorganic Chemistry 28 (4): 191–204. 2000. doi:10.1006/bioo.2000.1174. PMID 11034781.
- "Studies with substrate and cofactor analogues provide evidence for a radical mechanism in the chorismate synthase reaction". J. Biol. Chem. 275 (46): 35825–30. 2000. doi:10.1074/jbc.M005796200. PMID 10956653.
External links
![]() | Original source: https://en.wikipedia.org/wiki/Chorismate synthase.
Read more |