Physics:Heat pump
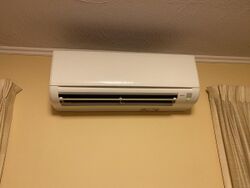
Part of a series about |
Sustainable energy |
---|
![]() |
Overview |
Energy conservation |
Renewable energy |
Sustainable transport |
|
A heat pump is a device that uses work to transfer heat from a cool space to a warm space by transferring thermal energy using a refrigeration cycle, cooling the cool space and warming the warm space. In cold weather a heat pump can move heat from the cool outdoors to warm a house; the pump may also be designed to move heat from the house to the warmer outdoors in warm weather. As they transfer heat rather than generating heat, they are more energy-efficient than other ways of heating or cooling a home.[1]
A gaseous refrigerant is compressed so its temperature rises. When operating as a heater in cold weather, the warmed gas flows to a heat exchanger in the indoor space where some of its thermal energy is transferred to that indoor space, causing the gas to condense to its liquid state. The liquified refrigerant flows to a heat exchanger in the outdoor space where the pressure falls, the liquid evaporates and the temperature of the gas falls. It is now colder than the temperature of the outdoor space being used as a heat source. It can again take up energy from the heat source, be compressed and repeat the cycle.
Air source heat pumps are the most common models, while other types include ground source heat pumps, water source heat pumps and exhaust air heat pumps. Large-scale heat pumps are also used in district heating systems.[2]
The efficiency of a heat pump is expressed as a coefficient of performance (COP), or seasonal coefficient of performance (SCOP). The higher the number, the more efficient a heat pump is. When used for space heating, heat pumps are typically more energy-efficient than electric resistance and other heaters.
Because of their high efficiency and the increasing share of fossil-free sources in electrical grids, heat pumps are playing a key role in climate change mitigation.[3][4] Consuming 1 kWh of electricity, they can transfer 1[5] to 4.5[6] kWh of thermal energy into a building. The carbon footprint of heat pumps depends on how electricity is generated, but they usually reduce emissions.[7] Heat pumps could satisfy over 80% of global space and water heating needs with a lower carbon footprint than gas-fired condensing boilers: however, in 2021 they only met 10%.[8]
Principle of operation
Heat flows spontaneously from a region of higher temperature to a region of lower temperature. Heat does not flow spontaneously from lower temperature to higher, but it can be made to flow in this direction if work is performed. The work required to transfer a given amount of heat is usually much less than the amount of heat; this is the motivation for using heat pumps in applications such as the heating of water and the interior of buildings.[9]
The amount of work required to drive an amount of heat Q from a lower-temperature reservoir such as ambient air to a higher-temperature reservoir such as the interior of a building is: [math]\displaystyle{ W = \frac{ Q}{\mathrm{COP}} }[/math] where
- [math]\displaystyle{ W }[/math] is the work performed on the working fluid by the heat pump's compressor.
- [math]\displaystyle{ Q }[/math] is the heat transferred from the lower-temperature reservoir to the higher-temperature reservoir.
- [math]\displaystyle{ \mathrm{COP} }[/math] is the instantaneous coefficient of performance for the heat pump at the temperatures prevailing in the reservoirs at one instant.
The coefficient of performance of a heat pump is greater than unity so the work required is less than the heat transferred, making a heat pump a more efficient form of heating than electrical resistance heating. As the temperature of the higher-temperature reservoir increases in response to the heat flowing into it, the coefficient of performance decreases, causing an increasing amount of work to be required for each unit of heat being transferred.[9]
The coefficient of performance, and the work required by a heat pump can be calculated easily by considering an ideal heat pump operating on the reversed Carnot cycle:
- If the low-temperature reservoir is at a temperature of 270 K (−3 °C) and the interior of the building is at 280 K (7 °C) the relevant coefficient of performance is 27. This means only 1 joule of work is required to transfer 27 joules of heat from a reservoir at 270 K to another at 280 K. The one joule of work ultimately ends up as thermal energy in the interior of the building so for each 27 joules of heat that are removed from the low-temperature reservoir, 28 joules of heat are added to the building interior, making the heat pump even more attractive from an efficiency perspective.
- As the temperature of the interior of the building rises progressively to 300 K (27 °C) the coefficient of performance falls progressively to 9. This means each joule of work is responsible for transferring 9 joules of heat out of the low-temperature reservoir and into the building. Again, the 1 joule of work ultimately ends up as thermal energy in the interior of the building so 10 joules of heat are added to the building interior.
History
Milestones:
- 1748
- William Cullen demonstrates artificial refrigeration.
- 1834
- Jacob Perkins builds a practical refrigerator with dimethyl ether.
- 1852
- Lord Kelvin describes the theory underlying heat pumps.[10]
- 1855–1857
- Peter von Rittinger develops and builds the first heat pump.[11]
- 1877
- In the period before 1875, heat pumps were for the time being pursued for vapour compression evaporation (open heat pump process) in salt works with their obvious advantages for saving wood and coal. In 1857, Peter von Rittinger was the first to try to implement the idea of vapor compression in a small pilot plant. Presumably inspired by Rittinger's experiments in Ebensee, Antoine-Paul Piccard from the University of Lausanne and the engineer J. H. Weibel from the Weibel–Briquet company in Geneva built the world's first really functioning vapor compression system with a two-stage piston compressor. In 1877 this first heat pump in Switzerland was installed in the Bex salt works.[10][12]
- 1928
- Aurel Stodola constructs a closed-loop heat pump (water source from Lake Geneva) which provides heating for the Geneva city hall to this day.
- 1937–1945
- During and after the First World War, Switzerland suffered from heavily difficult energy imports and subsequently expanded its hydropower plants. In the period before and especially during the Second World War, when neutral Switzerland was completely surrounded by fascist-ruled countries, the coal shortage became alarming again. Thanks to their leading position in energy technology, the Swiss companies Sulzer, Escher Wyss and Brown Boveri built and put in operation around 35 heat pumps between 1937 and 1945. The main heat sources were lake water, river water, groundwater, and waste heat. Particularly noteworthy are the six historic heat pumps from the city of Zurich with heat outputs from 100 kW to 6 MW. An international milestone is the heat pump built by Escher Wyss in 1937/38 to replace the wood stoves in the City Hall of Zurich. To avoid noise and vibrations, a recently developed rotary piston compressor was used. This historic heat pump heated the town hall for 63 years until 2001. Only then was it replaced by a new, more efficient heat pump,[10]
- 1945
- John Sumner, City Electrical Engineer for Norwich, installs an experimental water-source heat pump fed central heating system, using a nearby river to heat new Council administrative buildings. Seasonal efficiency ratio of 3.42. Average thermal delivery of 147 kW and peak output of 234 kW.[13]
- 1948
- Robert C. Webber is credited as developing and building the first ground-source heat pump.[14]
- 1951
- First large scale installation—the Royal Festival Hall in London is opened with a town gas-powered reversible water-source heat pump, fed by the Thames, for both winter heating and summer cooling needs.[13]
- 2019
- Kigali Amendment to phase out harmful refrigerants takes effect.
Types
Air-source heat pump
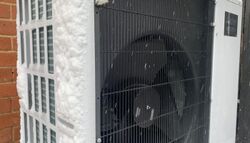
Air-source heat pumps are used to move heat between two heat exchangers, one outside the building which is fitted with fins through which air is forced using a fan and the other which either directly heats the air inside the building or heats water which is then circulated around the building through radiators or underfloor heating which releases the heat to the building. These devices can also operate in a cooling mode where they extract heat via the internal heat exchanger and eject it into the ambient air using the external heat exchanger. Some can be used to heat water for washing which is stored in a domestic hot water tank.[15]
Air-source heat pumps are relatively easy and inexpensive to install and have therefore historically been the most widely used heat pump type. In mild weather, coefficient of performance (COP) may be around 4,[16] while at temperatures below around −7 °C (19 °F) an air-source heat pump may still achieve a COP of 3.
While older air-source heat pumps performed relatively poorly at low temperatures and were better suited for warm climates, newer models with variable-speed compressors remain highly efficient in freezing conditions allowing for wide adoption and cost savings in places like Minnesota and Maine in the United States.[17]
Ground-source heat pump
Heat recovery ventilation
Exhaust air heat pumps extract heat from the exhaust air of a building and require mechanical ventilation. Two classes exist:
- Exhaust air-air heat pumps transfer heat to intake air.
- Exhaust air-water heat pumps transfer heat to a heating circuit that includes a tank of domestic hot water.
Solar-assisted heat pump
Water-source heat pump
A water-source heat pump works in a similar manner to a ground-source heat pump, except that it takes heat from a body of water rather than the ground. The body of water does, however, need to be large enough to be able to withstand the cooling effect of the unit without freezing or creating an adverse effect for wildlife.[18] The largest water-source heat pump was installed in the Danish town of Esbjerg in 2023.[19][20]
Others
A thermoacoustic heat pump operates as a thermoacoustic heat engine without refrigerant but instead uses a standing wave in a sealed chamber driven by a loudspeaker to achieve a temperature difference across the chamber.[21]
Electrocaloric heat pumps are solid state.[22]
Applications
The International Energy Agency estimated that, as of 2021, heat pumps installed in buildings have a combined capacity of more than 1000 GW.[8] They are used for heating, ventilation, and air conditioning (HVAC) and may also provide domestic hot water and tumble clothes drying.[23] The purchase costs are supported in various countries by consumer rebates.[24]
Space heating and sometimes also cooling
In HVAC applications, a heat pump is typically a vapor-compression refrigeration device that includes a reversing valve and optimized heat exchangers so that the direction of heat flow (thermal energy movement) may be reversed. The reversing valve switches the direction of refrigerant through the cycle and therefore the heat pump may deliver either heating or cooling to a building.
Because the two heat exchangers, the condenser and evaporator, must swap functions, they are optimized to perform adequately in both modes. Therefore, the Seasonal Energy Efficiency Rating (SEER) of a reversible heat pump is typically slightly less than those of two separately optimized machines. For equipment to receive the Energy Star rating, it must have a rating of at least 14 SEER. Pumps with ratings of 18 SEER or above are considered highly efficient. The highest efficiency heat pumps manufactured are up to 24 SEER.[25]
Water heating
In water heating applications, heat pumps may be used to heat or preheat water for swimming pools, homes or industry. Usually heat is extracted from outdoor air and transferred to an indoor water tank.[26][27]
District heating
Large (megawatt-scale) heat pumps are used for district heating.[28] However (As of 2022) about 90% of district heat is from fossil fuels. [29] In Europe, heat pumps account for a mere 1% of heat supply in district heating networks but several countries have targets to decarbonise their networks between 2030 and 2040.[2] Possible sources of heat for such applications are sewage water, ambient water (e.g. sea, lake and river water), industrial waste heat, geothermal energy, flue gas, waste heat from district cooling and heat from solar seasonal thermal energy storage. In Europe, more than 1500 MW of large-scale heat pumps were installed since the 1980s, of which about 1000 MW were in use in Sweden in 2017.[30] Large-scale heat pumps for district heating combined with thermal energy storage offer high flexibility for the integration of variable renewable energy. Therefore, they are regarded as a key technology for smart energy systems with high shares of renewable energy up to 100%, and advanced 4th generation district heating systems.[30][31][32] They are also a crucial element of cold district heating systems.[33]
Industrial heating
There is great potential to reduce the energy consumption and related greenhouse gas emissions in industry by application of industrial heat pumps. An international collaboration project completed in 2015 collected 39 examples of R&D-projects and 115 case studies worldwide.[34] The study shows that short payback periods of less than 2 years are possible, while achieving a high reduction of CO
2 emissions (in some cases more than 50%).[35][36] Industrial heat pumps can heat up to 200°C, and can meet the heating demands of many light industries.[37][38] In Europe alone, 15 GW of heat pumps could be installed in 3,000 facilities in the paper, food and chemicals industries.[2]
Performance
The performance of a heat pump is determined by the ability of the pump to extract heat from a low temperature environment (the source) and deliver it to a higher temperature environment (the sink).[39] Performance varies, depending on installation details, temperature differences, site elevation, location on site, pipe runs, flow rates, and maintenance.
Common performance metrics are the SEER (in cooling mode) and seasonal coefficient of performance (SCOP) (commonly used just for heating), although SCOP can be used for both modes of operation.[39] Larger values of either metric indicate better performance.[39] When comparing the performance of heat pumps, the term performance is preferred to efficiency, with coefficient of performance (COP) being used to describe the ratio of useful heat movement per work input.[39] An electrical resistance heater has a COP of 1.0, which is considerably lower than a well-designed heat pump which will typically have a COP of 3 to 5 with an external temperature of 10 °C and an internal temperature of 20 °C. Because the ground is a constant temperature source, a ground-source heat pump is not subjected to large temperature fluctuations, and therefore is the most energy-efficient type of heat pump.[39]
The "seasonal coefficient of performance" (SCOP) is a measure of the aggregate energy efficiency measure over a period of one year which is dependent on regional climate.[39] One framework for this calculation is given by the Commission Regulation (EU) No. 813/2013.[40]
A heat pump's operating performance in cooling mode is characterized in the US by either its energy efficiency ratio (EER) or seasonal energy efficiency ratio (SEER), both of which have units of BTU/(h·W) (note that 1 BTU/(h·W) = 0.293 W/W) and larger values indicate better performance.
Pump type and source | Typical use | 35 °C (e.g. heated screed floor) |
45 °C (e.g. heated screed floor) |
55 °C (e.g. heated timber floor) |
65 °C (e.g. radiator or DHW) |
75 °C (e.g. radiator and DHW) |
85 °C (e.g. radiator and DHW) |
---|---|---|---|---|---|---|---|
High-efficiency air-source heat pump (ASHP), air at −20 °C[41] | 2.2 | 2.0 | ‐ | ‐ | ‐ | ‐ | |
Two-stage ASHP, air at −20 °C[42] | Low source temperature | 2.4 | 2.2 | 1.9 | ‐ | ‐ | ‐ |
High-efficiency ASHP, air at 0 °C[41] | Low output temperature | 3.8 | 2.8 | 2.2 | 2.0 | ‐ | ‐ |
Prototype transcritical CO2 (R744) heat pump with tripartite gas cooler, source at 0 °C[43] | High output temperature | 3.3 | ‐ | ‐ | 4.2 | ‐ | 3.0 |
Ground-source heat pump (GSHP), water at 0 °C[41] | 5.0 | 3.7 | 2.9 | 2.4 | ‐ | ‐ | |
GSHP, ground at 10 °C[41] | Low output temperature | 7.2 | 5.0 | 3.7 | 2.9 | 2.4 | ‐ |
Theoretical Carnot cycle limit, source −20 °C | 5.6 | 4.9 | 4.4 | 4.0 | 3.7 | 3.4 | |
Theoretical Carnot cycle limit, source 0 °C | 8.8 | 7.1 | 6.0 | 5.2 | 4.6 | 4.2 | |
Theoretical Lorentzen cycle limit (CO2 pump), return fluid 25 °C, source 0 °C[43] | 10.1 | 8.8 | 7.9 | 7.1 | 6.5 | 6.1 | |
Theoretical Carnot cycle limit, source 10 °C | 12.3 | 9.1 | 7.3 | 6.1 | 5.4 | 4.8 |
Carbon footprint
The carbon footprint of heat pumps depends on their individual efficiency and how electricity is produced. An increasing share of low-carbon energy sources such as wind and solar will lower the impact on the climate.
heating system | emissions of energy source | efficiency | resulting emissions for thermal energy |
---|---|---|---|
heat pump with onshore wind power | 11 gCO 2/kWh[44] |
400% (COP=4) | 3 gCO 2/kWh |
heat pump with global electricity mix | 436 gCO 2/kWh[45] (2022) |
400% (COP=4) | 109 gCO 2/kWh |
natural-gas thermal (high efficiency) | 201 gCO 2/kWh[46] |
90%[citation needed] | 223 gCO 2/kWh |
heat pump electricity by lignite (old power plant) and low performance |
1221 gCO 2/kWh[46] |
300% (COP=3) | 407 gCO 2/kWh |
In most settings, heat pumps will reduce CO
2 emissions compared to heating systems powered by fossil fuels.[47] In regions accounting for 70% of world energy consumption, the emissions savings of heat pumps compared with a high-efficiency gas boiler are on average above 45% and reach 80% in countries with cleaner electricity mixes.[2] These values can be improved by 10 percentage points, respectively, with alternative refrigerants. In the United States, 70% of houses could reduce emissions by installing a heat pump.[48][2] The rising share of renewable electricity generation in many countries is set to increase the emissions savings from heat pumps over time.[2]
Heating systems powered by green hydrogen are also low-carbon and may become competitors, but are much less efficient due to the energy loss associated with hydrogen conversion, transport and use. In addition, not enough green hydrogen is expected to be available before the 2030s or 2040s.[49][50]
Operation
Vapor-compression uses a circulating refrigerant as the medium which absorbs heat from one space, compresses it thereby increasing its temperature before releasing it in another space. The system normally has 8 main components: a compressor, a reservoir, a reversing valve which selects between heating and cooling mode, two thermal expansion valves (one used when in heating mode and the other when used in cooling mode) and two heat exchangers, one associated with the external heat source/sink and the other with the interior. In heating mode the external heat exchanger is the evaporator and the internal one being the condenser; in cooling mode the roles are reversed.
Circulating refrigerant enters the compressor in the thermodynamic state known as a saturated vapor[51] and is compressed to a higher pressure, resulting in a higher temperature as well. The hot, compressed vapor is then in the thermodynamic state known as a superheated vapor and it is at a temperature and pressure at which it can be condensed with either cooling water or cooling air flowing across the coil or tubes. In heating mode this heat is used to heat the building using the internal heat exchanger, and in cooling mode this heat is rejected via the external heat exchanger.
The condensed, liquid refrigerant, in the thermodynamic state known as a saturated liquid, is next routed through an expansion valve where it undergoes an abrupt reduction in pressure. That pressure reduction results in the adiabatic flash evaporation of a part of the liquid refrigerant. The auto-refrigeration effect of the adiabatic flash evaporation lowers the temperature of the liquid and-vapor refrigerant mixture to where it is colder than the temperature of the enclosed space to be refrigerated.
The cold mixture is then routed through the coil or tubes in the evaporator. A fan circulates the warm air in the enclosed space across the coil or tubes carrying the cold refrigerant liquid and vapor mixture. That warm air evaporates the liquid part of the cold refrigerant mixture. At the same time, the circulating air is cooled and thus lowers the temperature of the enclosed space to the desired temperature. The evaporator is where the circulating refrigerant absorbs and removes heat which is subsequently rejected in the condenser and transferred elsewhere by the water or air used in the condenser.
To complete the refrigeration cycle, the refrigerant vapor from the evaporator is again a saturated vapor and is routed back into the compressor.
Over time, the evaporator may collect ice or water from ambient humidity. The ice is melted through defrosting cycle. An internal heat exchanger is either used to heat/cool the interior air directly or to heat water that is then circulated through radiators or underfloor heating circuit to either heat or cool the buildings.
Improvement of coefficient of performance (COP) by subcooling
Heat input can be improved if the refrigerant enters the evaporator with a lower vapor content. This can be achieved by cooling the liquid refrigerant after condensation. The gaseous refrigerant condenses on the heat exchange surface of the condenser. To achieve a heat flow from the gaseous flow center to the wall of the condenser, the temperature of the liquid refrigerant must be lower than the condensation temperature.
Additional subcooling can be achieved by heat exchange between relatively warm liquid refrigerant leaving the condenser and the cooler refrigerant vapor emerging from the evaporator. The enthalpy difference required for the subcooling leads to the superheating of the vapor drawn into the compressor. When the increase in cooling achieved by subcooling is greater that the compressor drive input required to overcome the additional pressure losses, such a heat exchange improves the coefficient of performance.[52]
One disadvantage of the subcooling of liquids is that the difference between the condensing temperature and the heat-sink temperature must be larger. This leads to a moderately high pressure difference between condensing and evaporating pressure, whereby the compressor energy increases.
Refrigerant choice
Pure refrigerants can be divided into organic substances (hydrocarbons (HCs), chlorofluorocarbons (CFCs), hydrochlorofluorocarbons (HCFCs), hydrofluorocarbons (HFCs), hydrofluoroolefins (HFOs), and HCFOs), and inorganic substances (ammonia (NH3), carbon dioxide (CO2), and water (H2O)[53]).[54] Their boiling points are usually below −25 °C.[55]
In the past 200 years, the standards and requirements for new refrigerants have changed. Nowadays low global warming potential (GWP) is required, in addition to all the previous requirements for safety, practicality, material compatibility, appropriate atmospheric life,[clarification needed] and compatibility with high-efficiency products. By 2022, devices using refrigerants with a very low GWP still have a small market share but are expected to play an increasing role due to enforced regulations,[56] as most countries have now ratified the Kigali Amendment to ban HFCs.[57] Isobutane (R600A) and propane (R290) are far less harmful to the environment than conventional hydrofluorocarbons (HFC) and are already being used in air-source heat pumps.[58] Propane may be the most suitable for high temperature heat pumps.[59] Ammonia (R717) and carbon dioxide (R744) also have a low GWP. (As of 2023) smaller CO2 heat pumps are not widely available and research and development of them continues.[60]
Until the 1990s, heat pumps, along with fridges and other related products used chlorofluorocarbons (CFCs) as refrigerants, which caused major damage to the ozone layer when released into the atmosphere. Use of these chemicals was banned or severely restricted by the Montreal Protocol of August 1987.[61]
Replacements, including R-134a and R-410A, are hydrofluorocarbons (HFC) with similar thermodynamic properties with insignificant ozone depletion potential (ODP) but had problematic GWP.[62] HFCs are powerful greenhouse gases which contribute to climate change.[63][64] Dimethyl ether (DME) also gained in popularity as a refrigerant in combination with R404a.[65] More recent refrigerants include difluoromethane (R32) with a lower GWP, but still over 600.
refrigerant | 20-year GWP | 100-year GWP |
---|---|---|
R-290 propane[66] | 0.072 | 0.02 |
R-600a isobutane | 3[67] | |
R-32[66] | 491 | 136 |
R-410a[68] | 4705 | 2285 |
R-134a[68] | 4060 | 1470 |
R-404a[68] | 7258 | 4808 |
Devices with R-290 refrigerant (propane) are expected to play a key role in the future.[59][69] The GWP of propane is about 500 times less than conventional HFC refrigerants and thus extremely low. The flammability of propane requires additional security measures. This issue can be targeted with a reduced charge.[70] By 2022, an increasing number of devices with R-290 were offered for domestic use, especially in Europe.
At the same time, HFC refrigerants still dominate the market. Recent government mandates have seen the phase-out of R-22 refrigerant. Replacements such as R-32 and R-410A are being promoted as environmentally friendly but still have a high GWP.[71] A heat pump typically uses 3 kg of refrigerant. With R-32 this amount still has a 20-year impact equivalent to 7 tons of CO
2, which corresponds to two years of natural gas heating in an average household. Refrigerants with a high ODP have already been phased out.
Government incentives
Financial incentives aim to protect consumers from high fossil gas costs and to reduce greenhouse gas emissions,[72] and are currently available in more than 30 countries around the world, covering more than 70% of global heating demand in 2021.[2]
Australia
Food processors, brewers, petfood producers and other industrial energy users are exploring whether it is feasible to use renewable energy to produce industrial-grade heat. Process heating accounts for the largest share of onsite energy use in Australian manufacturing, with lower-temperature operations like food production particularly well-suited to transition to renewables.
To help producers understand how they could benefit from making the switch, the Australian Renewable Energy Agency (ARENA) provided funding to the Australian Alliance for Energy Productivity (A2EP) to undertake pre-feasibility studies at a range of sites around Australia, with the most promising locations advancing to full feasibility studies.[73]
In an effort to incentivize energy efficiency and reduce environmental impact, the Australian states of Victoria, New South Wales, and Queensland have implemented rebate programs targeting the upgrade of existing hot water systems. These programs specifically encourage the transition from traditional gas or electric systems to heat pump based systems.[74][75][76][77][78]
Canada
In 2022, the Canada Greener Homes Grant[79] provides up to $5000 for upgrades (including certain heat pumps), and $600 for energy efficiency evaluations.
China
Purchase subsidies in rural areas in the 2010s reduced burning coal for heating, which had been causing ill health.[80]
United Kingdom
As of 2022: heat pumps have no Value Added Tax (VAT) although in Northern Ireland they are taxed at the reduced rate of 5% instead of the usual level of VAT of 20% for most other products.[81] (As of 2022) the installation cost of a heat pump is more than a gas boiler, but with the "Boiler Upgrade Scheme"[82] government grant and assuming electricity/gas costs remain similar their lifetime costs would be similar.[83]
United States
The High-efficiency Electric Home Rebate Program was created in 2022 to award grants to State energy offices and Indian Tribes in order to establish state-wide high-efficiency electric-home rebates. Effective immediately, American households are eligible for a tax credit to cover the costs of buying and installing a heat pump, up to $2,000. Starting in 2023, low- and moderate-level income households will be eligible for a heat-pump rebate of up to $8,000.[84]
In 2022, more heat pumps were sold in the United States than natural gas furnaces.[85]
In November 2023 Biden's administration allocated 169 million dollars from the Inflation Reduction Act to speed production of heat pumps. It used the Defense Production Act for doing so, because according to the administration, energy that is better for climate, is better also for national security.[86]
Some US states and municipalities have previously offered incentives for air-source heat pumps:
- California
- In 2022, the California Public Utilities Commission allocated an additional $40 million from the 2023 gas Cap-and-Trade allowance auction proceeds to the existing $44.7 million budget of the Self-Generation Incentive Program (SGIP) Heat Pump Water Heater (HPWH) program, in which single-family residential customers can receive an incentive of up to $3,800 to install a HPWH. Half of the incentive funds are reserved for low-income utility customers, who are eligible for a maximum incentive of $4,885.[87]
- Maine
- The Efficiency Maine Trust offers residential heat-pump rebates of up to $1,200, as well as heat-pump rebates for low and moderate income Mainers of $2,000 for their first eligible heat pump and up to $400 for a second eligible heat pump.[88][89]
- Massachusetts
- Mass Save, a collaborative initiative between Massachusetts' natural gas and electric utilities and energy efficiency service providers, offers an air-source heat-pump rebate of up to $10,000, which covers the purchase price of the heat pump and installation costs.[90]
- Minnesota
- Minnesota Power offers an air-source heat-pump rebate of up to $1,200 if the pump is bought and installed by a Minnesota Power Participating Contractor.[91]
- South Carolina
- Dominion Energy South Carolina offers a $400–$500 rebate for purchasing and installing an ENERGY STAR certified heat pump or air-conditioning unit.[92]
See also
References
- ↑ "Heat Pump Systems". US Department of Energy. https://www.energy.gov/energysaver/heat-pump-systems.
- ↑ 2.0 2.1 2.2 2.3 2.4 2.5 2.6 Technology Report: The Future of Heat Pumps (Report). November 2022. https://www.iea.org/reports/the-future-of-heat-pumps. Retrieved 2023-01-06. License: CC BY 4.0.
- ↑ IPCC AR6 WG3 Ch11 2022, Sec. 11.3.4.1.
- ↑ IPCC SR15 Ch2 2018, p. 142.
- ↑ Everitt, Neil (2023-09-11). "Study proves heat pump efficiency at low temperatures" (in en-GB). https://www.coolingpost.com/world-news/study-proves-heat-pump-efficiency-at-low-temperatures/.
- ↑ "How to find the Coefficient of Performance of a Heat Pump" (in en-US). https://unacademy.com/content/jee/study-material/physics/how-to-find-the-coefficient-of-performance-of-a-heat-pump/.
- ↑ Deetjen, Thomas A.; Walsh, Liam; Vaishnav, Parth (2021-07-28). "US residential heat pumps: the private economic potential and its emissions, health, and grid impacts" (in en). Environmental Research Letters 16 (8): 084024. doi:10.1088/1748-9326/ac10dc. ISSN 1748-9326. Bibcode: 2021ERL....16h4024D.
- ↑ 8.0 8.1 "Heat Pumps – Analysis" (in en-GB). https://www.iea.org/reports/the-future-of-heat-pumps.
- ↑ 9.0 9.1 G. F. C. Rogers and Y. R. Mayhew (1957), Engineering Thermodynamics, Work and Heat Transfer, Section 13.1, Longmans, Green & Company Limited.
- ↑ 10.0 10.1 10.2 "History of Heat Pumping Technologies in Switzerland – Texts". https://www.aramis.admin.ch/Texte/?ProjectID=45262.
- ↑ Banks, David L. (2008-05-06). An Introduction to Thermogeology: Ground Source Heating and Cooling. Wiley-Blackwell. ISBN 978-1-4051-7061-1. http://www.researchandmarkets.com/reports/2174113/an_introduction_to_thermogeology_ground_source.pdf. Retrieved 2014-03-05.
- ↑ Wirth, E. (1955) (in German), Aus der Entwicklungsgeschichte der Wärmepumpe, Schweizerische Bauzeitung, 73, pp. 647–650, https://www.e-periodica.ch/digbib/view?pid=sbz-002:1955:73#3306, retrieved 2021-11-20
- ↑ 13.0 13.1 Electricity supply in the United Kingdom : a chronology – from the beginnings of the industry to 31 December 1985. The Electricity Council.. 1987. ISBN 978-0851881058. OCLC 17343802.
- ↑ Banks, David (August 2012). An Introduction to Thermogeology: Ground Source Heating and Cooling. John Wiley & Sons. pp. 123.
- ↑ Lawrence, Karen. "Air source heat pumps explained" (in en). https://www.which.co.uk/reviews/ground-and-air-source-heat-pumps/article/air-source-heat-pumps-explained-al5MC4f773Zq.
- ↑ "Wärmepumpen mit Prüf- / Effizienznachweis (heat pumps with efficiency validation)" (in German). BAFA (Federal Office for Economic Affairs and Export Control in Germany). https://www.bafa.de/SharedDocs/Downloads/DE/Energie/ee_waermepumpen_anlagenliste_bis_2020.html.
- ↑ "Heat pumps do work in the cold – Americans just don't know it yet" (in en-us). 2022-05-09. https://grist.org/housing/heat-pumps-do-work-in-the-cold-americans-just-dont-know-it-yet/.
- ↑ Energy Saving Trust (2019-02-13). "Could a water source heat pump work for you?" (in en-GB). https://energysavingtrust.org.uk/could-water-source-heat-pump-work-you/.
- ↑ Baraniuk, Chris (2023-05-29). "The 'exploding' demand for giant heat pumps". https://www.bbc.com/news/business-65321487.
- ↑ Ristau, Oliver (2022-07-24). "Energy transition, the Danish way". https://www.dw.com/en/danish-port-city-to-play-key-role-in-europes-wind-energy-plans/a-62464055.
- ↑ Padavic-Callaghan, Karmela (6 December 2022). "Heat pump uses a loudspeaker and wet strips of paper to cool air" (in en-US). https://www.newscientist.com/article/2349973-heat-pump-uses-a-loudspeaker-and-wet-strips-of-paper-to-cool-air/.
- ↑ Everitt, Neil (2023-08-14). "Scientists claim solid-state heat pump breakthrough" (in en-GB). https://www.coolingpost.com/world-news/scientists-claim-solid-state-heat-pump-breakthrough/.
- ↑ "Heat Pump Systems". U.S. Department of Energy. http://energy.gov/energysaver/heat-pump-systems.
- ↑ "Renewable Heat Incentive – Domestic RHI – paid over 7 years". http://www.gshp.org.uk/RHI_Domestic.html.
- ↑ "Heat Pump Efficiency | Heat Pump SEER Ratings" (in en). https://www.carrier.com/residential/en/us/products/heat-pumps/heat-pump-efficiency/.
- ↑ "How it Works — Heat Pump Water Heaters (HPWHs)" (in en). https://www.energystar.gov/products/water_heaters/high_efficiency_electric_storage_water_heaters/how_it_works.
- ↑ "Heat-pump hot water systems" (in en). https://www.sustainability.vic.gov.au/energy-efficiency-and-reducing-emissions/save-energy-in-the-home/water-heating/choose-the-right-hot-water-system/heat-pump-water-heaters.
- ↑ Baraniuk, Chris (2023-05-29). "The 'exploding' demand for giant heat pumps" (in en-GB). BBC News. https://www.bbc.com/news/business-65321487.
- ↑ "District Heating - Energy System" (in en-GB). https://www.iea.org/energy-system/buildings/district-heating.
- ↑ 30.0 30.1 David, Andrei (2017). "Heat Roadmap Europe: Large-Scale Electric Heat Pumps in District Heating Systems". Energies 10 (4): 578. doi:10.3390/en10040578.
- ↑ Lund, Henrik (2014). "4th Generation District Heating (4GDH): Integrating smart thermal grids into future sustainable energy systems". Energy 68: 1–11. doi:10.1016/j.energy.2014.02.089.
- ↑ Sayegh, M. A. (2018). "Heat pump placement, connection and operational modes in European district heating". Energy and Buildings 166: 122–144. doi:10.1016/j.enbuild.2018.02.006. http://bura.brunel.ac.uk/handle/2438/15834. Retrieved 2019-07-10.
- ↑ Buffa, Simone (2019), "5th generation district heating and cooling systems: A review of existing cases in Europe" (in de), Renewable and Sustainable Energy Reviews 104: 504–522, doi:10.1016/j.rser.2018.12.059
- ↑ IEA HPT TCP Annex 35
- ↑ IEA HPT TCP Annex 35 Publications
- ↑ "Application of Industrial Heat Pumps. Annex 35 two-page summary." (in en). https://heatpumpingtechnologies.org/publications/application-of-industrial-heat-pumps-annex-35-two-page-summary/.
- ↑ "Norwegian Researchers Develop World's Hottest Heat Pump" (in en-US). 2021-08-05. https://ammonia21.com/norwegian-researchers-develop-worlds-hottest-heat-pump/.
- ↑ "Heat pumps are key to helping industry turn electric" (in en-GB). https://www.wbcsd.org/3w9qh.
- ↑ 39.0 39.1 39.2 39.3 39.4 39.5 "Heating and cooling with a heat pump: Efficiency terminology". Natural Resources Canada. 8 September 2022. https://natural-resources.canada.ca/energy-efficiency/energy-star-canada/about/energy-star-announcements/publications/heating-and-cooling-heat-pump/6817#b6.
- ↑ "Ecodesign requirements for space heaters". https://eur-lex.europa.eu/legal-content/EN/TXT/?uri=CELEX%3A32013R0813.
- ↑ 41.0 41.1 41.2 41.3 The Canadian Renewable Energy Network 'Commercial Earth Energy Systems', Figure 29 . . Retrieved December 8, 2009.
- ↑ Technical Institute of Physics and Chemistry, Chinese Academy of Sciences 'State of the Art of Air-source Heat Pump for Cold Region', Figure 5 . . Retrieved April 19, 2008.
- ↑ 43.0 43.1 SINTEF Energy Research 'Integrated CO2 Heat Pump Systems for Space Heating and DHW in low-energy and passive houses', J. Steen, Table 3.1, Table 3.3 . . Retrieved April 19, 2008.
- ↑ "How Wind Can Help Us Breathe Easier" (in en). https://www.energy.gov/eere/wind/articles/how-wind-can-help-us-breathe-easier.
- ↑ "Global Electricity Review 2023" (in en-US). 2023-04-11. https://ember-climate.org/insights/research/global-electricity-review-2023/.
- ↑ 46.0 46.1 Quaschning 2022
- ↑ "The UK is sabotaging its own plan to decarbonize heating". 27 May 2021. https://www.engadget.com/air-source-heat-pumps-uk-120044198.html.
- ↑ Deetjen, Thomas A; Walsh, Liam; Vaishnav, Parth (2021-07-28). "US residential heat pumps: the private economic potential and its emissions, health, and grid impacts". Environmental Research Letters 16 (8): 084024. doi:10.1088/1748-9326/ac10dc. Bibcode: 2021ERL....16h4024D.
- ↑ "Can the UK rely on hydrogen to save its gas boilers?". 2021-05-21. https://inews.co.uk/news/can-the-uk-rely-on-hydrogen-to-save-its-gas-boilers-1014119.
- ↑ IEA (2022), Global Hydrogen Review 2022, IEA, Paris https://www.iea.org/reports/global-hydrogen-review-2022 , License: CC BY 4.0
- ↑ Saturated vapors and saturated liquids are vapors and liquids at their saturation temperature and saturation pressure. A superheated vapor is at a temperature higher than the saturation temperature corresponding to its pressure.
- ↑ Ludwig von Cube, Hans (1981). Heat Pump Technology. Butterworths. pp. 22–23. ISBN 0-408-00497-5. https://books.google.com/books?id=YNH8BAAAQBAJ&dq=heat+pump+principle&pg=PP1. Retrieved 2023-01-02.
- ↑ Chamoun, Marwan; Rulliere, Romuald; Haberschill, Philippe; Berail, Jean Francois (2012-06-01). "Dynamic model of an industrial heat pump using water as refrigerant". International Journal of Refrigeration 35 (4): 1080–1091. doi:10.1016/j.ijrefrig.2011.12.007. ISSN 0140-7007. https://www.sciencedirect.com/science/article/pii/S0140700711003082.
- ↑ Wu, Di (2021). "Vapor compression heat pumps with pure Low-GWP refrigerants". Renewable and Sustainable Energy Reviews 138: 110571. doi:10.1016/j.rser.2020.110571. ISSN 1364-0321. https://doi.org/10.1016/j.rser.2020.110571. Retrieved 2022-11-17.
- ↑ "Everything you need to know about the wild world of heat pumps" (in en). https://www.technologyreview.com/2023/02/14/1068582/everything-you-need-to-know-about-heat-pumps/.
- ↑ Miara, Marek (2019-10-22). "Heat Pumps with Climate-Friendly Refrigerant Developed for Indoor Installation". Fraunhofer ISE. https://www.ise.fraunhofer.de/en/press-media/press-releases/2019/heat-pumps-with-climate-friendly-refrigerant-developed-for-indoor-installation.html.
- ↑ Rabe, Barry G. (2022-09-23). "Pivoting from global climate laggard to leader: Kigali and American HFC policy" (in en-US). https://www.brookings.edu/blog/fixgov/2022/09/23/pivoting-from-global-climate-laggard-to-leader-kigali-and-american-hfc-policy/.
- ↑ Itteilag, Richard L. (2012-08-09) (in en). Green Electricity and Global Warming. AuthorHouse. pp. 77. ISBN 9781477217405. https://books.google.com/books?id=LpStalb-tF4C&q=R600A+isobutane+ozone+environment&pg=PA77. Retrieved 2020-11-01.
- ↑ 59.0 59.1 "Propane-powered heat pumps are greener". The Economist. 2023-09-06. ISSN 0013-0613. https://www.economist.com/science-and-technology/2023/09/06/propane-powered-heat-pumps-are-greener.
- ↑ "Smart CO2 Heat Pump". https://www.dti.dk/co2-heat-pump-20-200-kw/44672.
- ↑ "Handbook for the Montreal Protocol on Substances that Deplete the Ozone Layer – 7th Edition". United Nations Environment Programme – Ozone Secretariat. 2007. http://ozone.unep.org/Publications/MP_Handbook/Section_1.2_Control_measures/Annex_A_-_Group_I.shtml.
- ↑ "Refrigerants – Environmental Properties". http://www.engineeringtoolbox.com/Refrigerants-Environment-Properties-d_1220.html.
- ↑ R-410A.
- ↑ Ecometrica.com (27 June 2012). "Calculation of green house gas potential of R-410A". http://ecometrica.com/article/calculating-the-global-warming-potential-of-refrigerant-gas-mixes.
- ↑ "R404 and DME Refrigerant blend as a new solution to limit global warming potential". March 14, 2012. http://www.mecanica.pub.ro/frigo-eco/R404A_DME.pdf.
- ↑ 66.0 66.1 IPCC_AR6_WG1_Ch7 2021, 7SM-26
- ↑ LearnMetrics (2023-05-12). "List of Low GWP Refrigerants: 69 Refrigerants Below 500 GWP" (in en-GB). https://learnmetrics.com/low-gwp-refrigerants/.
- ↑ 68.0 68.1 68.2 "Global warming potential (GWP) of HFC refrigerants" (in en). https://iifiir.org/en/encyclopedia-of-refrigeration/global-warming-potential-gwp-of-hfc-refrigerants.
- ↑ Everitt, Neil (2023-09-15). "Qvantum plant has 1 million heat pump capacity" (in en-GB). https://www.coolingpost.com/world-news/qvantum-plant-has-1-million-heat-pump-capacity/.
- ↑ Miara, Marek (22 October 2019). "Heat Pumps with Climate-Friendly Refrigerant Developed for Indoor Installation". Fraunhofer ISE. https://www.ise.fraunhofer.de/en/press-media/press-releases/2019/heat-pumps-with-climate-friendly-refrigerant-developed-for-indoor-installation.html.
- ↑ US Environmental Protection Agency, OAR (14 November 2014). "Phaseout of Ozone-Depleting Substances (ODS)" (in en). https://www.epa.gov/ods-phaseout.
- ↑ "Heat Pumps". https://www.iea.org/energy-system/buildings/heat-pumps.
- ↑ "Electrifying industrial processes with heat pumps.". 22 March 2022. https://arena.gov.au/blog/electrifying-industrial-processes-with-heat-pumps/.
- ↑ Department of Energy, Environment and Climate Action, Victoria Government (Australia) (2023-10-11). "Hot water systems for businesses" (in en-AU). Victoria Government. https://www.energy.vic.gov.au/businesses/victorian-energy-upgrades-businesses/hot-water-systems.
- ↑ Department of Energy, Environment and Climate Action (Australia), Victoria Government (2023-09-23). "Hot water systems for households" (in en-AU). Victoria Government. https://www.energy.vic.gov.au/households/victorian-energy-upgrades-for-households/hot-water-systems.
- ↑ New South Wales Climate and Energy Action, New South Wales Government (Australia) (2023-12-08). "Upgrade your hot water system" (in en-AU). NSW Government. https://www.energy.nsw.gov.au/households/rebates-grants-and-schemes/household-energy-saving-upgrades/upgrade-your-hot-water.
- ↑ Australian Government, Queensland (2023-10-05). "Queensland Business Energy Saving and Transformation Rebates" (in en-AU). Queensland Government. https://www.business.qld.gov.au/running-business/energy-business/energy-efficiency-rebate.
- ↑ Time To Save (2023-11-21). "Hot Water Rebates in Australia: A Detailed Guide For Businesses" (in en-AU). Timetosave. https://www.timetosave.com.au/hot-water-rebates-in-australia/.
- ↑ "Canada Greener Homes Grant". 17 March 2021. https://www.nrcan.gc.ca/energy-efficiency/homes/canada-greener-homes-grant/23441.
- ↑ "Coal fired boiler replacement in Beijing rural area". https://hpa.ehpa.org/coal-fired-boiler-replacement-in-beijing-rural-area-2/.
- ↑ "HMCR rates for goods and services". 11 July 2022. https://www.gov.uk/guidance/rates-of-vat-on-different-goods-and-services#power-utilities-energy-and-energy-saving-heating.
- ↑ "Apply for the Boiler Upgrade Scheme". https://www.gov.uk/apply-boiler-upgrade-scheme.
- ↑ "BBC Radio 4 – Sliced Bread, Air Source Heat Pumps" (in en-GB). https://www.bbc.co.uk/programmes/m0016pvn.
- ↑ Shao, Elena. "H. R. 5376 – Inflation Reduction Act of 2022". U.S. Congress. https://www.congress.gov/bill/117th-congress/house-bill/5376/text.
- ↑ "As Heat Pumps Go Mainstream, a Big Question: Can They Handle Real Cold?". The New York Times. February 22, 2023. https://www.nytimes.com/interactive/2023/02/22/climate/heat-pumps-extreme-cold.html.
- ↑ Frazin, Rachel (17 November 2023). "Biden administration uses wartime authority to bolster energy efficient manufacturing". The Hill. https://thehill.com/policy/energy-environment/4315744-biden-admin-wartime-authority-bolster-energy-efficient-manufacturing/.
- ↑ "CPUC Provides Additional Incentives and Framework for Electric Heat Pump Water Heater Program". California Public Utilities Commission. https://www.cpuc.ca.gov/news-and-updates/all-news/cpuc-provides-additional-incentives-and-framework-for-electric-heat-pump-water-heater-program.
- ↑ "Residential Heat Pump Rebates". The Efficiency Maine Trust. https://www.efficiencymaine.com/at-home/ductless-heat-pumps/.
- ↑ "Heat Pump Rebates for Low and Moderate Income Mainers". The Efficiency Maine Trust. https://www.efficiencymaine.com/income-eligible-heat-pump/.
- ↑ "Air Source Heat Pump Rebates". https://www.masssave.com/saving/residential-rebates/air-source-heat-pumps.
- ↑ "ASHP Rebates". https://www.mnpower.com/ProgramsRebates/ASHPRebates.
- ↑ "Rebates for Heating & Cooling System Replacements". https://www.dominionenergy.com/south-carolina/save-energy/heating-and-cooling-rebates/replacing-new-equipment.
Sources
IPCC reports
- IPCC (2021). Masson-Delmotte, V.; Zhai, P.; Pirani, A. et al.. eds (in en). Climate Change 2021: The Physical Science Basis. Contribution of Working Group I to the Sixth Assessment Report of the Intergovernmental Panel on Climate Change. Cambridge University Press (In Press). https://www.ipcc.ch/report/ar6/wg1/downloads/report/IPCC_AR6_WGI_Full_Report.pdf.
- Forster, P.; Storelvmo, T.; Armour, K.; Collins, W. (2021). "Chapter 7: The Earth's energy budget, climate feedbacks, and climate sensitivity Supplementary Material". IPCC AR6 WG1 2021. https://www.ipcc.ch/report/ar6/wg1/downloads/report/IPCC_AR6_WGI_Chapter07_SM.pdf.
- Global Warming of 1.5°C. An IPCC Special Report on the impacts of global warming of 1.5°C above pre-industrial levels and related global greenhouse gas emission pathways, in the context of strengthening the global response to the threat of climate change, sustainable development, and efforts to eradicate poverty. Intergovernmental Panel on Climate Change. 2018. https://www.ipcc.ch/site/assets/uploads/sites/2/2019/06/SR15_Full_Report_High_Res.pdf. https://www.ipcc.ch/sr15/.
- IPCC (2022). Shula, P. R.; Skea, J.; Slade, R. et al.. eds. Climate Change 2022: Mitigation of Climate Change. Contribution of Working Group III to the Sixth Assessment Report of the Intergovernmental Panel on Climate Change. Cambridge, UK and New York, New York, USA: Cambridge University Press (In Press). https://report.ipcc.ch/ar6wg3/pdf/IPCC_AR6_WGIII_FinalDraft_FullReport.pdf. Retrieved 2022-05-10.
Other
- Quaschning, Volker. "Specific Carbon Dioxide Emissions of Various Fuels". https://www.volker-quaschning.de/datserv/CO2-spez/index_e.php.
External links
- Video explaining very simply how heat pumps work
- IEA Technology Collaboration Programme on Heat Pumping Technologies
- Carbon Brief guest post: How heat pump sales are starting to take off around the world
![]() | Original source: https://en.wikipedia.org/wiki/Heat pump.
Read more |