Earth:Oligocene
Oligocene | |
---|---|
33.9 – 23.03 Ma | |
Chronology | |
Etymology | |
Name formality | Formal |
Name ratified | 1978 |
Usage information | |
Celestial body | Earth |
Regional usage | Global (ICS) |
Time scale(s) used | ICS Time Scale |
Definition | |
Chronological unit | Epoch |
Stratigraphic unit | Series |
Time span formality | Formal |
Lower boundary definition | LAD of Planktonic Foraminifers Hantkenina and Cribrohantkenina |
Lower boundary GSSP | Massignano quarry section, Massignano, Ancona, Italy [ ⚑ ] 43°31′58″N 13°36′04″E / 43.5328°N 13.6011°E |
GSSP ratified | 1992[3] |
Upper boundary definition |
|
Upper boundary GSSP | Lemme-Carrosio Section, Carrosio, Italy [ ⚑ ] 44°39′32″N 8°50′11″E / 44.6589°N 8.8364°E |
GSSP ratified | 1996 |
The Oligocene (IPA: /ˈɒlɪɡəsiːn, -ɡoʊ-/ OL-ə-gə-seen, -goh-)[4] is a geologic epoch of the Paleogene Period and extends from about 33.9 million to 23 million years before the present (33.9±0.1 to 23.03±0.05 Ma). As with other older geologic periods, the rock beds that define the epoch are well identified but the exact dates of the start and end of the epoch are slightly uncertain. The name Oligocene was coined in 1854 by the German paleontologist Heinrich Ernst Beyrich[5][6] from his studies of marine beds in Belgium and Germany.[7] The name comes from the Ancient Greek ὀλίγος (olígos, "few") and καινός (kainós, "new"),[8] and refers to the sparsity of extant forms of molluscs. The Oligocene is preceded by the Eocene Epoch and is followed by the Miocene Epoch. The Oligocene is the third and final epoch of the Paleogene Period.
The Oligocene is often considered an important time of transition, a link between the archaic world of the tropical Eocene and the more modern ecosystems of the Miocene.[9] Major changes during the Oligocene included a global expansion of grasslands, and a regression of tropical broad leaf forests to the equatorial belt.
The start of the Oligocene is marked by a notable extinction event called the Grande Coupure; it featured the replacement of European fauna with Asian fauna, except for the endemic rodent and marsupial families. By contrast, the Oligocene–Miocene boundary is not set at an easily identified worldwide event but rather at regional boundaries between the warmer late Oligocene and the relatively cooler Miocene.
Boundaries and subdivisions
The lower boundary of the Oligocene (its Global Boundary Stratotype Section and Point or GSSP) is placed at the last appearance of the foraminiferan genus Hantkenina in a quarry at Massignano, Italy. However, this GSSP has been criticized as excluding the uppermost part of the type Eocene Priabonian Stage and because it is slightly earlier than important climate shifts that form natural markers for the boundary, such as the global oxygen isotope shift marking the expansion of Antarctic glaciation (the Oi1 event).[10]
The upper boundary of the Oligocene is defined by its GSSP at Carrosio, Italy, which coincides with the first appearance of the foraminiferan Paragloborotalia kugleri and with the base of magnetic polarity chronozone C6Cn.2n.[11]
Oligocene faunal stages from youngest to oldest are:[3][12]
Chattian or late Oligocene | (28.1 – 23.03 mya) |
Rupelian or early Oligocene | (33.9 – 28.1 mya) |
Tectonics and paleogeography
During the Oligocene Epoch, the continents continued to drift toward their present positions.[13][14] Antarctica became more isolated as deep ocean channels were established between Antarctica and Australia and South America. Australia had been very slowly rifting away from West Antarctica since the Jurassic, but the exact timing of the establishment of ocean channels between the two continents remains uncertain. However, one estimate is that a deep channel was in place between the two continents by the end of the early Oligocene.[15] The timing of the formation of the Drake Passage between South America and Antarctica is also uncertain, with estimates ranging from 49 to 17 mya (early Eocene to Miocene),[16] but oceanic circulation through the Drake Passage may also have been in place by the end of the early Oligocene.[17][15] This may have been interrupted by a temporary constriction of the Drake Passage from sometime in the middle to late Oligocene (29 to 22 mya) to the middle Miocene (15 mya).[18]
The reorganization of the oceanic tectonic plates of the northeastern Pacific, which had begun in the Paleocene, culminated with the arrival of the Murray and Mendocino Fracture Zones at the North American subduction zone in the Oligocene. This initiated strike-slip movement along the San Andreas Fault and extensional tectonics in the Basin and Range province,[19] ended volcanism south of the Cascades, and produced clockwise rotation of many western North American terranes. The Rocky Mountains were at their peak. A new volcanic arc was established in western North America, far inland from the coast, reaching from central Mexico through the Mogollon-Datil volcanic field to the San Juan volcanic field, then through Utah and Nevada to the ancestral Northern Cascades. Huge ash deposits from these volcanoes created the White River and Arikaree Groups of the High Plains, with their excellent fossil beds.[20]
Between 31 and 26 mya, the Ethiopia-Yemen Continental Flood Basalts were emplaced by the East African large igneous province, which also initiated rifting along the Red Sea and Gulf of Aden.[21]
The Alps were rapidly rising in Europe as the African plate continued to push north into the Eurasian plate, isolating the remnants of the Tethys Sea.[13][22] Sea levels were lower in the Oligocene than in the early Eocene, exposing large coastal plains in Europe and the Gulf Coast and Atlantic Coast of North America. The Obik Sea, which had separated Europe from Asia, retreated early in the Oligocene, creating a persistent land connection between the continents.[13] The Paratethys Sea stretched from what is now the Balkan Peninsula across Central Asia to the Tian Shan region of what is now Xinjiang.[23] There appears to have been a land bridge in the early Oligocene between North America and Europe, since the faunas of the two regions are very similar.[24] However, towards the end of the Oligocene, there was a brief marine incursion in Europe.[25][26]
The rise of the Himalayas during the Oligocene remains poorly understood. One recent hypothesis is that a separate microcontinent collided with south Asia in the early Eocene, and India itself did not collide with south Asia until the end of the Oligocene.[27][28] The Tibetan Plateau may have reached nearly its present elevation by the late Oligocene.[29]
The Andes first became a major mountain chain in the Oligocene, as subduction became more direct into the coastline.[20][30]
Climate
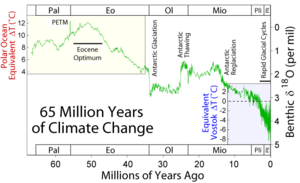
Climate during the Oligocene reflected a general cooling trend following the Early Eocene Climatic Optimum. This transformed the Earth's climate from a greenhouse to an icehouse climate.[32]
Eocene-Oligocene transition and Oi1 event
The Eocene-Oligocene transition was a major cooling event and reorganization of the biosphere,[33][34] being part of a broader trend of global cooling lasting from the Bartonian to the Rupelian.[35][36] The transition is marked by the Oi1 event, an oxygen isotope excursion occurring approximately 33.55 million years ago,[37] during which oxygen isotope ratios decreased by 1.3‰. About 0.3–0.4‰ of this is estimated to be due to major expansion of Antarctic ice sheets. The remaining 0.9 to 1.0‰ was due to about 5 to 6 °C (9 to 10 °F) of global cooling.[32] The transition likely took place in three closely spaced steps over the period from 33.8 to 33.5 mya. By the end of the transition, sea levels had dropped by 105 meters (344 ft), and ice sheets were 25% greater in extent than in the modern world.[38]
The effects of the transition can be seen in the geological record at many locations around the world. Ice volumes rose as temperature and sea levels dropped.[39] Playa lakes of the Tibetan Plateau disappeared at the transition, pointing to cooling and aridification of central Asia.[40] Pollen and spore counts in marine sediments of the Norwegian-Greenland Sea indicate a drop in winter temperatures at high latitudes of about 5 °C (9.0 °F) just prior to the Oi1 event.[41] Borehole dating from the Southeast Faroes drift indicates that deep-ocean circulation from the Arctic Ocean to the North Atlantic Ocean began in the early Oligocene.[42]
The best terrestrial record of Oligocene climate comes from North America, where temperatures dropped by 7 to 11 °C (13 to 20 °F) in the earliest Oligocene. This change is seen from Alaska to the Gulf Coast. Upper Eocene paleosols reflect annual precipitation of over a meter of rain, but early Oligocene precipitation was less than half this.[43][44] In central North America, the cooling was by 8.2 ± 3.1 °C over a period of 400,000 years, though there is little indication of significant increase in aridity during this interval.[45] Ice-rafted debris in the Norwegian-Greenland Sea indicated that glaciers had appeared in Greenland by the start of the Oligocene.[46]
Continental ice sheets in Antarctica reached sea level during the transition.[47][48][49] Glacially rafted debris of early Oligocene age in the Weddell Sea and Kerguelen Plateau, in combination with Oi1 isotope shift, provides unambiguous evidence of a continental ice sheet on Antarctica by the early Oligocene.[50]
The causes of the Eocene-Oligocene transition are not yet fully understood.[51] The timing is wrong for this to be caused either by known impact events or by the volcanic activity on the Ethiopean Plateau.[52] Two other possible drivers of climate change, not mutually exclusive, have been proposed.[51] The first is thermal isolation of the continent of Antarctica by development of the Antarctic Circumpolar Current.[17][48][14] Deep sea cores from south of New Zealand suggest that cold deep-sea currents were present by the early Oligocene.[52] However, the timing of this event remains controversial.[53] The other possibility, for which there is considerable evidence, is a drop in atmospheric carbon dioxide levels (pCO2) during the transition.[51][54][35] The pCO2 is estimated to have dropped just before the transition, to 760 ppm at the peak of ice sheet growth, then rebounded slightly before resuming a more gradual fall.[55] Climate modeling suggests that glaciation of Antarctica took place only when pCO2 dropped below a critical threshold value.[56]
Middle Oligocene climate and the Oi2 event
Oligocene climate following the Eocene-Oligocene event is poorly known.[57] There were several pulses of glaciation in middle Oligocene, about the time of the Oi2 oxygen isotope shift. This led to the largest drop of sea level in past 100 million years, by about 75 meters (246 ft). This is reflected in a mid-Oligocene incision of continental shelves and unconformities in marine rocks around the world.[43]
Some evidence suggests that the climate remained warm at high latitudes[57][58] even as ice sheets experienced cyclical growth and retreat in response to orbital forcing and other climate drivers.[59] Other evidence indicates significant cooling at high latitudes.[47][60] Part of the difficulty may be that there were strong regional variations in the response to climate shifts. Evidence of a relatively warm Oligocene suggests an enigmatic climate state, neither hothouse nor icehouse.[61]
Late Oligocene warming
The late Oligocene (26.5 to 24 mya) likely saw a warming trend in spite of low pCO2 levels, though this appears to vary by region.[62] However, Antarctica remained heavily glaciated during this warming period.[63][64] The late Oligocene warming is discernible in pollen counts from the Tibetan Plateau, which also show that the South Asian Monsoon had already developed by the late Oligocene.[65] Around 25.8 Ma, the South Asian Monsoon underwent an episode of major intensification brought on by the uplift of the Tibetan Plateau.[66]
A deep 400,000-year glaciated Oligocene-Miocene boundary event is recorded at McMurdo Sound and King George Island.[67]
Biosphere

The early Eocene climate was very warm, with crocodilians and temperate plants thriving north of the Arctic Circle. The cooling trend that began in the middle Eocene continued into the Oligocene, bringing the poles well below freezing for the first time in the Phanerozoic. The cooling climate, together with the opening of some land bridges and the closing of others, led to a profound reorganization of the biosphere and loss of taxonomic diversity. Land animals and marine organisms reached a Phanerozoic low in diversity by the late Oligocene, and the temperate forests and jungles of the Eocene were replaced by forest and scrubland. The closing of the Tethys Seaway destroyed its tropical biota.[68]
Flora
The Oi1 event of the Eocene-Oligocene transition covered the continent of Antarctica with ice sheets, leaving Nothofagus and mosses and ferns clinging to life around the periphery of Antarctica in tundra conditions.[56]
Angiosperms continued their expansion throughout the world as tropical and sub-tropical forests were replaced by temperate deciduous forests. Open plains and deserts became more common and grasses expanded from their water-bank habitat in the Eocene moving out into open tracts.[69] The decline in pCO2 favored C4 photosynthesis,[70] which is found only in angiosperms and is particularly characteristic of grasses.[71] However, even at the end of the period, grass was not quite common enough for modern savannas.[69]
In North America, much of the dense forest was replaced by patchy scrubland with riparian forests.[43][44] Subtropical species dominated with cashews[72] and lychee trees present,[73] and temperate woody plants such as roses, beeches,[74] and pines[75] were common. The legumes spread,[76] while sedges[77] and ferns continued their ascent.[78]
In Europe, floral assemblages became increasingly affected by strengthening seasonality as it related to wildfire activity.[79]
The Ha Long megafossil flora from the Dong Ho Formation of Oligocene age shows that the Oligocene flora of what is now Vietnam was very similar to its present flora.[80]
Fauna

Most extant mammal families had appeared by the end of the Oligocene. These included primitive three-toed horses, rhinoceroses, camels, deer, and peccaries. Carnivores such as dogs, nimravids, bears, weasels, and raccoons began to replace the creodonts that had dominated the Paleocene in the Old World. Rodents and rabbits underwent tremendous diversification due to the increase in suitable habitats for ground-dwelling seed eaters, as habitats for squirrel-like nut- and fruit-eaters diminished. The primates, once present in Eurasia, were reduced in range to Africa and South America.[81] Many groups, such as equids,[82] entelodonts, rhinos, merycoidodonts, and camelids, became more able to run during this time, adapting to the plains that were spreading as the Eocene rainforests receded.[83] Brontotheres died out in the Earliest Oligocene, and creodonts died out outside Africa and the Middle East at the end of the period. Multituberculates, an ancient lineage of primitive mammals that originated back in the Jurassic, also became extinct in the Oligocene, aside from the gondwanatheres.[84]
The Eocene-Oligocene transition in Europe and Asia has been characterized as the Grande Coupure.[85] The lowering of sea levels closed the Turgai Strait across the Obik Sea, which had previously separated Asia from Europe. This allowed Asian mammals, such as rhinoceroses and ruminants, to enter Europe and drive endemic species to extinction.[81] Lesser faunal turnovers occurred simultaneously with the Oi2 event and towards the end of the Oligocene.[86] There was significant diversification of mammals in Eurasia, including the giant indricotheres, that grew up to 6 meters (20 ft) at the shoulder and weighed up to 20 tons. Paraceratherium was one of the largest land mammals ever to walk the Earth.[87] However, the indricotheres were an exception to a general tendency for Oligocene mammals to be much smaller than their Eocene counterparts.[69] The earliest deer, giraffes, pigs, and cattle appeared in the mid-Oligocene in Eurasia.[81] The first felid, Proailurus, originated in Asia during the late Oligocene and spread to Europe.[88]

There was only limited migration between Asia and North America.[81] The cooling of central North America at the Eocene-Oligocene transition resulted in a large turnover of gastropods, amphibians, and reptiles. Mammals were much less affected.[45] Crocodilians and pond turtles replaced by dry land tortoises. Molluscs shifted to more drought-tolerant forms.[43] The White River Fauna of central North America inhabited a semiarid prairie home and included entelodonts like Archaeotherium, camelids (such as Poebrotherium), running rhinoceratoids, three-toed equids (such as Mesohippus), nimravids, protoceratids, and early canids like Hesperocyon.[89] Merycoidodonts, an endemic American group, were very diverse during this time.[90]
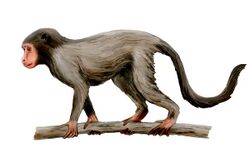
Australia and South America became geographically isolated and developed their own distinctive endemic fauna. These included the New World and Old World monkeys. The South American continent was home to animals such as pyrotheres and astrapotheres, as well as litopterns and notoungulates. Sebecosuchians, terror birds, and carnivorous metatheres, like the borhyaenids remained the dominant predators.[91]
Africa was also relatively isolated and retained its endemic fauna. These included mastodonts, hyraxes, arsinoitheres, and other archaic forms.[81] Egypt in the Oligocene was an environment of lush forested deltas.[92] Nevertheless, the Early Oligocene saw a major reduction in the diversity of many Afro-Arabian mammal clades, including hyaenodonts, primates, and hystricognath and anomaluroid rodents.[93]
During the Oligocene, the Tethyan marine biodiversity hotspot collapsed as the Tethys Ocean contracted. The seas around Southeast Asia and Australia became the new dominant hotspot of marine biodiversity.[94] At sea, 97% of marine snail species, 89% of clams, and 50% of echinoderms of the Gulf Coast did not survive past the earliest Oligocene. New species evolved, but the overall diversity diminished. Cold-water mollusks migrated around the Pacific Rim from Alaska and Siberia.[81] The marine animals of Oligocene oceans resembled today's fauna, such as the bivalves. Calcareous cirratulids appeared in the Oligocene.[95]
The Oligocene saw the emergence of parrotfishes, as the centre of marine biodiversity shifted from the Central Tethys eastward into the Indo-Pacific.[96] The fossil record of marine mammals is a little spotty during this time, and not as well known as the Eocene or Miocene, but some fossils have been found. The baleen whales and toothed whales had just appeared, and their ancestors, the archaeocete cetaceans began to decrease in diversity due to their lack of echolocation, which was very useful as the water became colder and cloudier. Other factors to their decline could include climate changes and competition with today's modern cetaceans and the requiem sharks, which also appeared in this epoch. Early desmostylians, like Behemotops, are known from the Oligocene. Pinnipeds appeared near the end of the epoch from an otter-like ancestor.[97]
Oceans
The Oligocene sees the beginnings of modern ocean circulation, with tectonic shifts causing the opening and closing of ocean gateways. Cooling of the oceans had already commenced by the Eocene/Oligocene boundary,[98] and they continued to cool as the Oligocene progressed. The formation of permanent Antarctic ice sheets during the early Oligocene and possible glacial activity in the Arctic may have influenced this oceanic cooling, though the extent of this influence is still a matter of some significant dispute.
Effects of oceanic gateways on circulation
The opening and closing of ocean gateways: the opening of the Drake Passage; the opening of the Tasmanian Gateway and the closing of the Tethys seaway; along with the final formation of the Greenland–Iceland–Faroes Ridge; played vital parts in reshaping oceanic currents during the Oligocene. As the continents shifted to a more modern configuration, so too did ocean circulation.[99]
Drake Passage
The Drake Passage is located between South America and Antarctica. Once the Tasmanian Gateway between Australia and Antarctica opened, all that kept Antarctica from being completely isolated by the Southern Ocean was its connection to South America. As the South American continent moved north, the Drake Passage opened and enabled the formation of the Antarctic Circumpolar Current (ACC), which would have kept the cold waters of Antarctica circulating around that continent and strengthened the formation of Antarctic Bottom Water (ABW).[99][100] With the cold water concentrated around Antarctica, sea surface temperatures and, consequently, continental temperatures would have dropped. The onset of Antarctic glaciation occurred during the early Oligocene,[101] and the effect of the Drake Passage opening on this glaciation has been the subject of much research. However, some controversy still exists as to the exact timing of the passage opening, whether it occurred at the start of the Oligocene or nearer the end. Even so, many theories agree that at the Eocene/Oligocene (E/O) boundary, a yet shallow flow existed between South America and Antarctica, permitting the start of an Antarctic Circumpolar Current.[102]
Stemming from the issue of when the opening of the Drake Passage took place, is the dispute over how great of an influence the opening of the Drake Passage had on the global climate. While early researchers concluded that the advent of the ACC was highly important, perhaps even the trigger, for Antarctic glaciation[99] and subsequent global cooling, other studies have suggested that the δ18O signature is too strong for glaciation to be the main trigger for cooling.[102] Through study of Pacific Ocean sediments, other researchers have shown that the transition from warm Eocene ocean temperatures to cool Oligocene ocean temperatures took only 300,000 years,[98] which strongly implies that feedbacks and factors other than the ACC were integral to the rapid cooling.[98]
The latest hypothesized time for the opening of the Drake Passage is during the early Miocene.[98] Despite the shallow flow between South America and Antarctica, there was not enough of a deep water opening to allow for significant flow to create a true Antarctic Circumpolar Current. If the opening occurred as late as hypothesized, then the Antarctic Circumpolar Current could not have had much of an effect on early Oligocene cooling, as it would not have existed.
The earliest hypothesized time for the opening of the Drake Passage is around 30 Ma.[98] One of the possible issues with this timing was the continental debris cluttering up the seaway between the two plates in question. This debris, along with what is known as the Shackleton Fracture Zone, has been shown in a recent study to be fairly young, only about 8 million years old.[100] The study concludes that the Drake Passage would be free to allow significant deep water flow by around 31 Ma. This would have facilitated an earlier onset of the Antarctic Circumpolar Current. There is some evidence that it occurred much earlier, during the early Eocene.[103]
Opening of the Tasman Gateway
The other major oceanic gateway opening during this time was the Tasman, or Tasmanian, depending on the paper, gateway between Australia and Antarctica. The time frame for this opening is less disputed than the Drake Passage and is largely considered to have occurred around 34 Ma. As the gateway widened, the Antarctic Circumpolar Current strengthened.
Tethys Seaway closing
The Tethys Seaway was not a gateway, but rather a sea in its own right. Its closing during the Oligocene had significant impact on both ocean circulation and climate. The collisions of the African plate with the European plate and of the Indian subcontinent with the Asian plate, cut off the Tethys Seaway that had provided a low-latitude ocean circulation.[104] The closure of Tethys built some new mountains (the Zagros range) and drew down more carbon dioxide from the atmosphere, contributing to global cooling.[105]
Greenland–Iceland–Faroes
The gradual separation of the clump of continental crust and the deepening of the tectonic ridge in the North Atlantic that would become Greenland, Iceland, and the Faroe Islands helped to increase the deep water flow in that area.[101] More information about the evolution of North Atlantic Deep Water will be given a few sections down.
Ocean cooling
Evidence for ocean-wide cooling during the Oligocene exists mostly in isotopic proxies. Patterns of extinction[106] and patterns of species migration[107] can also be studied to gain insight into ocean conditions. For a while, it was thought that the glaciation of Antarctica may have significantly contributed to the cooling of the ocean, however, recent evidence tends to deny this.[100][108]
Deep water

Isotopic evidence suggests that during the early Oligocene, the main source of deep water was the North Pacific and the Southern Ocean. As the Greenland-Iceland-Faroe Ridge sank and thereby connected the Norwegian–Greenland sea with the Atlantic Ocean, the deep water of the North Atlantic began to come into play as well. Computer models suggest that once this occurred, a more modern in appearance thermo-haline circulation started.[104]
Evidence for the early Oligocene onset of chilled North Atlantic deep water lies in the beginnings of sediment drift deposition in the North Atlantic, such as the Feni and Southeast Faroe drifts.[101]
The chilling of the South Ocean deep water began in earnest once the Tasmanian Gateway and the Drake Passage opened fully.[100] Regardless of the time at which the opening of the Drake Passage occurred, the effect on the cooling of the Southern Ocean would have been the same.
Impact events
Recorded extraterrestrial impacts:
- Haughton impact crater, Nunavut, Canada (23 Ma, crater 24 km (15 mi) diameter; now considered questionable as an Oligocene event; later analyses have concluded the crater dates to 39 Ma, placing the event in the Eocene.)[109][110]
Supervolcanic explosions
- La Garita Caldera (28–26 million years ago)[111]
- Wah Wah Springs Caldera (30 million years ago)
See also
- List of fossil sites (with link directory)
- Turgai Sea
References
- ↑ Zachos, J. C.; Kump, L. R. (2005). "Carbon cycle feedbacks and the initiation of Antarctic glaciation in the earliest Oligocene". Global and Planetary Change 47 (1): 51–66. doi:10.1016/j.gloplacha.2005.01.001. Bibcode: 2005GPC....47...51Z.
- ↑ "International Chronostratigraphic Chart". International Commission on Stratigraphy. https://stratigraphy.org/icschart/ChronostratChart2020-01.pdf.
- ↑ 3.0 3.1 Silva, Isabella; Jenkins, D. (September 1993). "Decision on the Eocene-Oligocene boundary stratotype". Episodes 16 (3): 379–382. doi:10.18814/epiiugs/1993/v16i3/002. https://stratigraphy.org/gssps/files/rupelian.pdf. Retrieved 13 December 2020.
- ↑ "Oligocene Definition & Meaning". Dictionary.com. https://www.dictionary.com/browse/oligocene. Retrieved 2022-05-13.
- ↑ Beyrich (November 1854). "Über die Stellung der hessische Tertiärbildungen". Verhandlungen Köngliche Preussischen Akademie Wissenschaft Berlin [Proceedings of the Royal Prussian Academy of Sciences at Berlin]: 640–666. https://babel.hathitrust.org/cgi/pt?id=hvd.32044092888627;view=1up;seq=660. From p. 664: "Der neue Name Oligocän mag sich zwischenstellen zwischen das ältere Eocän und das jüngere Miocän." (The new name Oligocene may be interposed between the older Eocene and the younger Miocene.)
- ↑ Wilmarth, Mary Grace (1925). Bulletin 769: The Geologic Time Classification of the United States Geological Survey Compared With Other Classifications, accompanied by the original definitions of era, period and epoch terms. Washington, D.C., U.S.: U.S. Government Printing Office. p. 53. https://books.google.com/books?id=my7x_PBkpm4C&pg=PA53.
- ↑ Prothero 2005, p. 472.
- ↑ "Oligocene". Oligocene. http://www.etymonline.com/index.php?term=Oligocene&allowed_in_frame=0.
- ↑ Haines, Tim; Walking with Beasts: A Prehistoric Safari, (New York: Dorling Kindersley Publishing, Inc., 1999)
- ↑ Prothero 2005, pp. 472–473.
- ↑ Steininger, Fritz F.; Aubry, M.P.; Berggren, W.A.; Biolzi, M.; M.Borsetti, A.; Cartlidge, Julie E.; Cati, F.; Corfield, R. et al. (1 March 1997). "The Global Stratotype Section and Point (GSSP) for the base of the Neogene". Episodes 20 (1): 23–28. doi:10.18814/epiiugs/1997/v20i1/005.
- ↑ Coccioni, Rodolfo; Montanari, Alessandro; Nice, David; Brinkhuis, Henk; Deino, Alain; Frontalini, Fabrizio; Liter, Fabrizio; Maiorano, Patricia et al. (1 March 2018). "The Global Stratotype Section and Point (GSSP) for the base of the Chattian stage (Paleogene System, Oligocene Series) at Monte Cagnero, Italy". Episodes 41 (1): 17–32. doi:10.18814/epiiugs/2018/v41i1/018003.
- ↑ 13.0 13.1 13.2 Prothero 2005, pp. 476–477.
- ↑ 14.0 14.1 Torsvik, Trond H.; Cocks, L. Robin M. (2017). Earth history and palaeogeography. Cambridge, United Kingdom: Cambridge University Press. pp. 241–245. ISBN 9781107105324.
- ↑ 15.0 15.1 Torsvik & Cocks 2017, pp. 251–252.
- ↑ Scher, H. D.; Martin, E. E. (21 April 2006). "Timing and Climatic Consequences of the Opening of Drake Passage". Science 312 (5772): 428–430. doi:10.1126/science.1120044. PMID 16627741. Bibcode: 2006Sci...312..428S.
- ↑ 17.0 17.1 Prothero 2005, pp. 474, 476.
- ↑ Lagabrielle, Yves; Goddéris, Yves; Donnadieu, Yannick; Malavieille, Jacques; Suarez, Manuel (30 March 2009). "The tectonic history of Drake Passage and its possible impacts on global climate". Earth and Planetary Science Letters 279 (3–4): 197–211. doi:10.1016/j.epsl.2008.12.037. Bibcode: 2009E&PSL.279..197L. https://hal.archives-ouvertes.fr/hal-00413525.
- ↑ Torsvik & Cocks 2017, p. 245.
- ↑ 20.0 20.1 Prothero 2005, p. 477.
- ↑ Torsvik & Cocks 2017, pp. 241, 249.
- ↑ Torsvik & Cocks 2017, pp. 241–245.
- ↑ Li, Qian; Li, Long; Zhang, Yuanyuan; Guo, Zhaojie (20 April 2020). "Oligocene incursion of the Paratethys seawater to the Junggar Basin, NW China: insight from multiple isotopic analysis of carbonate". Scientific Reports 10 (1): 6601. doi:10.1038/s41598-020-63609-0. PMID 32313139. Bibcode: 2020NatSR..10.6601L.
- ↑ Denk, Thomas; Grímsson, Friðgeir; Zetter, Reinhard; Símonarson, Leifur A. (2011). "The Biogeographic History of Iceland – the North Atlantic Land Bridge Revisited". Late Cainozoic Floras of Iceland. Topics in Geobiology. 35. pp. 647–668. doi:10.1007/978-94-007-0372-8_12. ISBN 978-94-007-0371-1.
- ↑ Rousse, Stephane; Duringer, Philippe; Stapf, Karl R. G. (July 2012). "An exceptional rocky shore preserved during Oligocene (Late Rupelian) transgression in the Upper Rhine Graben (Mainz Basin, Germany): OLIGOCENE ROCKY SHORE". Geological Journal 47 (4): 388–408. doi:10.1002/gj.1349.
- ↑ Filek, Thomas; Hofmayer, Felix; Feichtinger, Iris; Berning, Björn; Pollerspöck, Jürgen; Zwicker, Jennifer; Smrzka, Daniel; Peckmann, Jörn et al. (July 2021). "Environmental conditions during the late Oligocene transgression in the North Alpine Foreland Basin (Eferding Formation, Egerian) – A multidisciplinary approach". Palaeogeography, Palaeoclimatology, Palaeoecology 580: 110527. doi:10.1016/j.palaeo.2021.110527. Bibcode: 2021PPP...58010527F.
- ↑ van Hinsbergen, D. J. J.; Lippert, P. C.; Dupont-Nivet, G.; McQuarrie, N.; Doubrovine, P. V.; Spakman, W.; Torsvik, T. H. (15 May 2012). "Greater India Basin hypothesis and a two-stage Cenozoic collision between India and Asia". Proceedings of the National Academy of Sciences 109 (20): 7659–7664. doi:10.1073/pnas.1117262109. PMID 22547792. Bibcode: 2012PNAS..109.7659V.
- ↑ Torsvik & Cocks 2017, p. 241.
- ↑ DeCelles, Peter G.; Quade, Jay; Kapp, Paul; Fan, Majie; Dettman, David L.; Ding, Lin (January 2007). "High and dry in central Tibet during the Late Oligocene". Earth and Planetary Science Letters 253 (3–4): 389–401. doi:10.1016/j.epsl.2006.11.001. Bibcode: 2007E&PSL.253..389D.
- ↑ Orme, Antony R. (2007). "The Tectonic Framework of South America". Physical Geography of South America. Oxford University Press. pp. 12–17. ISBN 978-0-19-531341-3. https://archive.org/details/physicalgeograph00vebl.
- ↑ Zachos, J.; Pagani, M.; Sloan, L.; Thomas, E.; Billups, K. (2001). "Trends, rhythms, and aberrations in global climate 65 Ma to present". Science 292 (5517): 686–693. doi:10.1126/science.1059412. PMID 11326091. Bibcode: 2001Sci...292..686Z. http://doc.rero.ch/record/13508/files/PAL_E304.pdf.
- ↑ 32.0 32.1 Prothero 2005, p. 473.
- ↑ Berggren, William A.; Prothero, Donald R. (1992). "Eocene-Oligocene climatic and biotic evolution: an overview". Eocene-Oligocene Climatic and Biotic Evolution. Princeton University Press. p. 1. doi:10.1515/9781400862924.1. ISBN 9781400862924.
- ↑ Coxall, H.K.; Pearson, P.N. (2007). "The Eocene–Oligocene Transition". Deep-Time Perspectives on Climate Change: Marrying the Signal from Computer Models and Biological Proxies. The Micropalaeontological Society, Special Publications. London: The Geological Society. pp. 351–387.
- ↑ 35.0 35.1 Lauretano, Vittoria; Kennedy-Asser, Alan T.; Korasidis, Vera A.; Wallace, Malcolm W.; Valdes, Paul J.; Lunt, Daniel J.; Pancost, Richard T.; Naafs, B. David A. (2 August 2021). "Eocene to Oligocene terrestrial Southern Hemisphere cooling caused by declining pCO2". Nature Geoscience 14 (9): 659–664. doi:10.1038/s41561-021-00788-z. Bibcode: 2021NatGe..14..659L. https://www.nature.com/articles/s41561-021-00788-z. Retrieved 8 December 2022.
- ↑ Gutiérrez, Néstor M.; Pino, Juan Pablo; Le Roux, Jacobus P.; Petroza, Viviana; Orayzun, José Luis; Hinojosa, Luis Felipe (15 August 2019). "An Oligocene microthermal forest dominated by Nothofagus in Sierra Baguales, Chilean Patagonia: Response to global cooling and tectonic events". Palaeogeography, Palaeoclimatology, Palaeoecology 528: 1–13. doi:10.1016/j.palaeo.2019.04.006. Bibcode: 2019PPP...528....1G. https://www.sciencedirect.com/science/article/pii/S0031018218303961. Retrieved 4 December 2022.
- ↑ Jovane, Luigi; Florindo, Fabio; Sprovieri, Mario; Pälike, Heiko (27 July 2006). "Astronomic calibration of the late Eocene/early Oligocene Massignano section (central Italy)". Geochemistry, Geophysics, Geosystems 7 (7): 1–10. doi:10.1029/2005GC001195. Bibcode: 2006GGG.....7.7012J. https://agupubs.onlinelibrary.wiley.com/doi/10.1029/2005GC001195. Retrieved 6 December 2022.
- ↑ Katz, Miriam E.; Miller, Kenneth G.; Wright, James D.; Wade, Bridget S.; Browning, James V.; Cramer, Benjamin S.; Rosenthal, Yair (May 2008). "Stepwise transition from the Eocene greenhouse to the Oligocene icehouse". Nature Geoscience 1 (5): 329–334. doi:10.1038/ngeo179. Bibcode: 2008NatGe...1..329K.
- ↑ Miller, K. G.; Browning, J. V.; Aubry, M.-P.; Wade, B. S.; Katz, M. E.; Kulpecz, A. A.; Wright, J. D. (1 January 2008). "Eocene-Oligocene global climate and sea-level changes: St. Stephens Quarry, Alabama". Geological Society of America Bulletin 120 (1–2): 34–53. doi:10.1130/B26105.1. Bibcode: 2008GSAB..120...34M.
- ↑ Dupont-Nivet, Guillaume; Krijgsman, Wout; Langereis, Cor G.; Abels, Hemmo A.; Dai, Shuang; Fang, Xiaomin (February 2007). "Tibetan plateau aridification linked to global cooling at the Eocene–Oligocene transition". Nature 445 (7128): 635–638. doi:10.1038/nature05516. PMID 17287807.
- ↑ Eldrett, James S.; Greenwood, David R.; Harding, Ian C.; Huber, Matthew (June 2009). "Increased seasonality through the Eocene to Oligocene transition in northern high latitudes". Nature 459 (7249): 969–973. doi:10.1038/nature08069. PMID 19536261. Bibcode: 2009Natur.459..969E.
- ↑ Davies, Richard; Cartwright, Joseph; Pike, Jennifer; Line, Charles (April 2001). "Early Oligocene initiation of North Atlantic Deep Water formation". Nature 410 (6831): 917–920. doi:10.1038/35073551. PMID 11309613. Bibcode: 2001Natur.410..917D.
- ↑ 43.0 43.1 43.2 43.3 Prothero 2005, p. 475.
- ↑ 44.0 44.1 Retallack, G.J. (1983). "Late Eocene and Oligocene paleosols from Badlands National Park, South Dakota". Geological Society of America Special Paper 193. ISBN 9780813721934.
- ↑ 45.0 45.1 Zanazzi, Alessandro; Kohn, Matthew J.; MacFadden, Bruce J.; Terry, Dennis O. (February 2007). "Large temperature drop across the Eocene–Oligocene transition in central North America". Nature 445 (7128): 639–642. doi:10.1038/nature05551. PMID 17287808.
- ↑ Eldrett, James S.; Harding, Ian C.; Wilson, Paul A.; Butler, Emily; Roberts, Andrew P. (March 2007). "Continental ice in Greenland during the Eocene and Oligocene". Nature 446 (7132): 176–179. doi:10.1038/nature05591. PMID 17287724. Bibcode: 2007Natur.446..176E.
- ↑ 47.0 47.1 Coxall & Pearson 2007, pp. 351–387.
- ↑ 48.0 48.1 Berggren & Prothero 1992, p. 1.
- ↑ O’Brien, Charlotte L.; Huber, Matthew; Thomas, Ellen; Pagani, Mark; Super, James R.; Elder, Leanne E.; Hull, Pincelli M. (13 October 2020). "The enigma of Oligocene climate and global surface temperature evolution". Proceedings of the National Academy of Sciences 117 (41): 25302–25309. doi:10.1073/pnas.2003914117. PMID 32989142. Bibcode: 2020PNAS..11725302O.
- ↑ Berggren & Prothero 1992, pp. 5–6.
- ↑ 51.0 51.1 51.2 O’Brien et al. 2020, p. 25302.
- ↑ 52.0 52.1 Prothero 2005, p. 474.
- ↑ Lyle, Mitchell; Gibbs, Samantha; Moore, Theodore C.; Rea, David K. (2007). "Late Oligocene initiation of the Antarctic Circumpolar Current: Evidence from the South Pacific". Geology 35 (8): 691. doi:10.1130/G23806A.1. Bibcode: 2007Geo....35..691L.
- ↑ Francis, J.E.; Marenssi, S.; Levy, R.; Hambrey, M.; Thorn, V.C.; Mohr, B.; Brinkhuis, H.; Warnaar, J. et al. (2008). "Chapter 8 From Greenhouse to Icehouse – The Eocene/Oligocene in Antarctica". Developments in Earth and Environmental Sciences 8: 309–368. doi:10.1016/S1571-9197(08)00008-6. ISBN 9780444528476.
- ↑ Pearson, Paul N.; Foster, Gavin L.; Wade, Bridget S. (October 2009). "Atmospheric carbon dioxide through the Eocene–Oligocene climate transition". Nature 461 (7267): 1110–1113. doi:10.1038/nature08447. PMID 19749741. Bibcode: 2009Natur.461.1110P.
- ↑ 56.0 56.1 Francis et al. 2008.
- ↑ 57.0 57.1 O’Brien et al. 2020, pp. 25302–25309.
- ↑ Lear, C. H. (14 January 2000). "Cenozoic Deep-Sea Temperatures and Global Ice Volumes from Mg/Ca in Benthic Foraminiferal Calcite". Science 287 (5451): 269–272. doi:10.1126/science.287.5451.269. PMID 10634774. Bibcode: 2000Sci...287..269L.
- ↑ Pälike, Heiko; Norris, Richard D.; Herrle, Jens O.; Wilson, Paul A.; Coxall, Helen K.; Lear, Caroline H.; Shackleton, Nicholas J.; Tripati, Aradhna K. et al. (22 December 2006). "The Heartbeat of the Oligocene Climate System". Science 314 (5807): 1894–1898. doi:10.1126/science.1133822. PMID 17185595. Bibcode: 2006Sci...314.1894P. https://eprints.soton.ac.uk/42935/2/Paelike_etal_Science2006_supplMat.pdf.
- ↑ Liu, Z.; Pagani, M.; Zinniker, D.; DeConto, R.; Huber, M.; Brinkhuis, H.; Shah, S. R.; Leckie, R. M. et al. (27 February 2009). "Global Cooling During the Eocene-Oligocene Climate Transition". Science 323 (5918): 1187–1190. doi:10.1126/science.1166368. PMID 19251622. Bibcode: 2009Sci...323.1187L. http://doc.rero.ch/record/210392/files/PAL_E4399.pdf.
- ↑ O’Brien et al. 2020, pp. 25303.
- ↑ O’Brien et al. 2020, pp. 25302-25303.
- ↑ Pekar, Stephen F.; DeConto, Robert M.; Harwood, David M. (February 2006). "Resolving a late Oligocene conundrum: Deep-sea warming and Antarctic glaciation". Palaeogeography, Palaeoclimatology, Palaeoecology 231 (1–2): 29–40. doi:10.1016/j.palaeo.2005.07.024. Bibcode: 2006PPP...231...29P. http://doc.rero.ch/record/13512/files/PAL_E309.pdf.
- ↑ Hauptvogel, D. W.; Pekar, S. F.; Pincay, V. (April 2017). "Evidence for a heavily glaciated Antarctica during the late Oligocene "warming" (27.8–24.5 Ma): Stable isotope records from ODP Site 690: LATE OLIGOCENE STABLE ISOTOPE RECORD". Paleoceanography 32 (4): 384–396. doi:10.1002/2016PA002972.
- ↑ Wu, Fuli; Miao, Yunfa; Meng, Qingquan; Fang, Xiaomin; Sun, Jimin (January 2019). "Late Oligocene Tibetan Plateau Warming and Humidity: Evidence From a Sporopollen Record". Geochemistry, Geophysics, Geosystems 20 (1): 434–441. doi:10.1029/2018GC007775. Bibcode: 2019GGG....20..434W.
- ↑ Jin, Chun-Sheng; Xu, Deke; Li, Mingsong; Hu, Pengxiang; Jiang, Zhaoxia; Liu, Jianxing; Miao, Yunfa; Wu, Fuli et al. (11 April 2023). "Tectonic and orbital forcing of the South Asian monsoon in central Tibet during the late Oligocene" (in en). Proceedings of the National Academy of Sciences of the United States of America 120 (15). doi:10.1073/pnas.2214558120. ISSN 0027-8424. PMID 37011203. PMC 10104490. https://pnas.org/doi/10.1073/pnas.2214558120. Retrieved 25 December 2023.
- ↑ Wilson, G.S.; Pekar, S.F.; Naish, T.R.; Passchier, S.; DeConto, R. (2008). "Chapter 9 The Oligocene–Miocene Boundary – Antarctic Climate Response to Orbital Forcing". Developments in Earth and Environmental Sciences 8: 369–400. doi:10.1016/S1571-9197(08)00009-8. ISBN 9780444528476.
- ↑ Prothero 2005, pp. 473–477.
- ↑ 69.0 69.1 69.2 Torsvik & Cocks 2017, p. 255.
- ↑ Christin, Pascal-Antoine; Besnard, Guillaume; Samaritani, Emanuela; Duvall, Melvin R.; Hodkinson, Trevor R.; Savolainen, Vincent; Salamin, Nicolas (January 2008). "Oligocene CO2 Decline Promoted C4 Photosynthesis in Grasses". Current Biology 18 (1): 37–43. doi:10.1016/j.cub.2007.11.058. PMID 18160293.
- ↑ "A portrait of the C4 photosynthetic family on the 50th anniversary of its discovery: species number, evolutionary lineages, and Hall of Fame". Journal of Experimental Botany 67 (14): 4039–56. July 2016. doi:10.1093/jxb/erw156. PMID 27053721.
- ↑ Méndez-Cárdenas, Juliana P.; Cevallos-Ferriz, Sergio R.S.; Calvillo-Canadell, Laura; Rodríguez-Yam, Gabriel A.; Borja, Amparo M.; Martínez-Cabrera, Hugo I. (August 2014). "Loxopterygium wood in Coayuca de Andrade, Oligocene of Puebla, Mexico". Review of Palaeobotany and Palynology 207: 38–43. doi:10.1016/j.revpalbo.2014.04.004. Bibcode: 2014RPaPa.207...38M.
- ↑ Buerki, Sven; Forest, Félix; Stadler, Tanja; Alvarez, Nadir (July 2013). "The abrupt climate change at the Eocene–Oligocene boundary and the emergence of South-East Asia triggered the spread of sapindaceous lineages". Annals of Botany 112 (1): 151–160. doi:10.1093/aob/mct106. PMID 23723259.
- ↑ Denk, Thomas; Grimm, Guido W. (December 2009). "The biogeographic history of beech trees". Review of Palaeobotany and Palynology 158 (1–2): 83–100. doi:10.1016/j.revpalbo.2009.08.007. Bibcode: 2009RPaPa.158...83D. https://www.sciencedirect.com/science/article/abs/pii/S0034666709001353. Retrieved 15 December 2023.
- ↑ Torsvik & Cocks 2017, p. 254.
- ↑ Herendeen, Patrick S.; Dilcher, David L. (March 1990). "Fossil mimosoid legumes from the Eocene and Oligocene of southeastern North America". Review of Palaeobotany and Palynology 62 (3–4): 339–361. doi:10.1016/0034-6667(90)90094-Y. Bibcode: 1990RPaPa..62..339H.
- ↑ Escudero, Marcial; Hipp, Andrew L.; Waterway, Marcia J.; Valente, Luis M. (June 2012). "Diversification rates and chromosome evolution in the most diverse angiosperm genus of the temperate zone (Carex, Cyperaceae)". Molecular Phylogenetics and Evolution 63 (3): 650–655. doi:10.1016/j.ympev.2012.02.005. PMID 22366369.
- ↑ Devore, M.L.; Pigg, K.B. (22 March 2010). "Floristic composition and comparison of middle Eocene to late Eocene and Oligocene floras in North America". Bulletin of Geosciences: 111–134. doi:10.3140/bull.geosci.1135.
- ↑ Uhl, Dieter; Spiekermann, Rafael; Wuttke, Michael; Poschmann, Markus J.; Jasper, André (1 February 2022). "Wildfires during the Paleogene (late Eocene–late Oligocene) of the Neuwied Basin (W-Germany)". Review of Palaeobotany and Palynology 297: 104565. doi:10.1016/j.revpalbo.2021.104565. ISSN 0034-6667. https://www.sciencedirect.com/science/article/pii/S0034666721001895. Retrieved 15 December 2023.
- ↑ Huang, Jian; Spicer, Robert A.; Li, Shu-Feng; Liu, Jia; Do, Truong Van; Nguyen, Hung Ba; Zhou, Zhe-Kun; Su, Tao (1 May 2022). "Long-term floristic and climatic stability of northern Indochina: Evidence from the Oligocene Ha Long flora, Vietnam". Palaeogeography, Palaeoclimatology, Palaeoecology 593: 110930. doi:10.1016/j.palaeo.2022.110930. Bibcode: 2022PPP...59310930H. https://www.sciencedirect.com/science/article/abs/pii/S0031018222001006. Retrieved 13 February 2023.
- ↑ 81.0 81.1 81.2 81.3 81.4 81.5 Prothero 2005, p. 476.
- ↑ Floyd, Andrea E. (2007). "Evolution of the equine digit and its relevance to the modern horse". Equine podiatry. Philadelphia, Pa.: Elsevier Saunders. ISBN 9781416064596.
- ↑ Prothero, Donald R. (2002). "Cloven hooves". Horns, tusks, and flippers : the evolution of hoofed mammals. Baltimore: Johns Hopkins University Press. p. 19. ISBN 9780801871351. https://books.google.com/books?id=kWpQX-sfsLgC&q=running. Retrieved 10 August 2021.
- ↑ Prothero, Donald R. (1985). "North American mammalian diversity and Eocene–Oligocene extinctions". Paleobiology 11 (4): 389–405. doi:10.1017/S0094837300011696. Bibcode: 1985Pbio...11..389P.
- ↑ Mennecart, Bastien; Aiglstorfer, Manuela; Li, Yikun; Li, Chunxiao; Wang, ShiQi (6 September 2021). "Ruminants reveal Eocene Asiatic palaeobiogeographical provinces as the origin of diachronous mammalian Oligocene dispersals into Europe" (in en). Scientific Reports 11 (1): 17710. doi:10.1038/s41598-021-96221-x. ISSN 2045-2322. PMC 8421421. https://www.nature.com/articles/s41598-021-96221-x. Retrieved 25 December 2023.
- ↑ Barberà, X.; Cabrera, L.; Marzo, M.; Parés, J.M.; Agustı́, J. (April 2001). "A complete terrestrial Oligocene magnetobiostratigraphy from the Ebro Basin, Spain". Earth and Planetary Science Letters 187 (1–2): 1–16. doi:10.1016/S0012-821X(01)00270-9. Bibcode: 2001E&PSL.187....1B. http://doc.rero.ch/record/16006/files/PAL_E3760.pdf.
- ↑ Prothero, D. (2013). Rhinoceros Giants: The Palaeobiology of Indricotheres. Indiana: Indiana University Press. pp. 87–106. ISBN 978-0-253-00819-0. https://www.google.com/books/edition/Rhinoceros_Giants/fbyn88ExO9IC?hl=en&gbpv=1&pg=PA87.
- ↑ Mott, Maryann (January 11, 2006). "Cats Climb New Family Tree". National Geographic News. http://news.nationalgeographic.com/news/2006/01/0111_060111_cat_evolution.html.
- ↑ Benton, Rachel C.; Terry, Dennis O. Jr.; Evanoff, Emmett; McDonald, Hugh Gregory (2015). The White River Badlands: Geology and Paleontology. Bloomington: Indiana University Press. ISBN 9780253016089. https://books.google.com/books?id=ZcFtCQAAQBAJ. Retrieved 10 August 2021.
- ↑ Saarinen, Juha; Mantzouka, Dimitra; Sakala, Jakub (2020). "Aridity, Cooling, Open Vegetation, and the Evolution of Plants and Animals During the Cenozoic". Nature through Time. Springer Textbooks in Earth Sciences, Geography and Environment. pp. 83–107. doi:10.1007/978-3-030-35058-1_3. ISBN 978-3-030-35057-4.
- ↑ Flynn, John J; Wyss, André R; Croft, Darin A; Charrier, Reynaldo (June 2003). "The Tinguiririca Fauna, Chile: biochronology, paleoecology, biogeography, and a new earliest Oligocene South American Land Mammal 'Age'". Palaeogeography, Palaeoclimatology, Palaeoecology 195 (3–4): 229–259. doi:10.1016/S0031-0182(03)00360-2. Bibcode: 2003PPP...195..229F.
- ↑ Benton, M. J. (2019). Cowen's history of life (Sixth ed.). Hoboken, NJ: John Wiley & Sons Ltd. p. 306. ISBN 9781119482215.
- ↑ de Vries, Dorien; Heritage, Steven; Borths, Matthew R.; Sallam, Hesham M.; Seiffert, Erik R. (7 October 2021). "Widespread loss of mammalian lineage and dietary diversity in the early Oligocene of Afro-Arabia" (in en). Communications Biology 4 (1): 1–9. doi:10.1038/s42003-021-02707-9. ISSN 2399-3642. https://www.nature.com/articles/s42003-021-02707-9. Retrieved 25 December 2023.
- ↑ Cowman, P. F.; Bellwood, D. R. (10 October 2011). "Coral reefs as drivers of cladogenesis: expanding coral reefs, cryptic extinction events, and the development of biodiversity hotspots". Journal of Evolutionary Biology 24 (12): 2543–2562. doi:10.1111/j.1420-9101.2011.02391.x. PMID 21985176.
- ↑ Vinn, O. (2009). "The ultrastructure of calcareous cirratulid (Polychaeta, Annelida) tubes". Estonian Journal of Earth Sciences 58 (2): 153–156. doi:10.3176/earth.2009.2.06. http://www.kirj.ee/public/Estonian_Journal_of_Earth_Sciences/2009/issue_2/earth-2009-2-153-156.pdf. Retrieved 2012-09-16.
- ↑ Siqueira, Alexandre C.; Bellwood, David R.; Cowman, Peter F. (4 June 2019). "Historical biogeography of herbivorous coral reef fishes: The formation of an Atlantic fauna". Journal of Biogeography 46 (7): 1611–1624. doi:10.1111/jbi.13631. https://onlinelibrary.wiley.com/doi/abs/10.1111/jbi.13631. Retrieved 9 May 2023.
- ↑ Handwerk, Brian (2009-03-22). "Seal with "Arms" Discovered". National Geographic News. http://news.nationalgeographic.com/news/2009/04/090422-seal-evolution-missing-link.html.
- ↑ 98.0 98.1 98.2 98.3 98.4 Lyle, Mitchell; Barron, J.; Bralower, T.; Huber, M.; Olivarez Lyle, A.; Ravelo, A. C.; Rea, D. K.; Wilson, P. A. (April 2008). "Pacific Ocean and Cenozoic evolution of climate". Reviews of Geophysics 46 (2): RG2002. doi:10.1029/2005RG000190. Bibcode: 2008RvGeo..46.2002L. https://deepblue.lib.umich.edu/bitstream/2027.42/95039/1/rog1637.pdf.
- ↑ 99.0 99.1 99.2 Prothero, D. (May 2005). "TERTIARY TO PRESENT | Oligocene". Tertiary to Present | Oligocene. 472–478. doi:10.1016/B0-12-369396-9/00056-3. ISBN 978-0-12-369396-9.
- ↑ 100.0 100.1 100.2 100.3 Mackensen, Andreas (Dec 2004). "Changing Southern Ocean palaeocirculation and effects on global climate". Antarctic Science 16 (4): 369–389. doi:10.1017/S0954102004002202. Bibcode: 2004AntSc..16..369M.
- ↑ 101.0 101.1 101.2 Via, Rachael; Thomas, D. (June 2006). "Evolution of Antarctic thermohaline circulation: Early Oligocene onset of deep-water production in the North Atlantic". Geology 34 (6): 441–444. doi:10.1130/G22545.1. Bibcode: 2006Geo....34..441V.
- ↑ 102.0 102.1 Katz, M; Cramer, B.; Toggweiler, J.; Esmay, G.; Liu, C.; Miller, K.; Rosenthal, Y.; Wade, B. et al. (May 2011). "Impact of Antarctic Circumpolar Current development on late Paleogene ocean structure". Science 332 (6033): 1076–1079. doi:10.1126/science.1202122. PMID 21617074. Bibcode: 2011Sci...332.1076K.
- ↑ van de Lagemaat, S.H.A. et al. (April 2021). "Subduction initiation in the Scotia Sea region and opening of the Drake Passage: When and why?". Earth-Science Reviews 215: 103351. doi:10.1016/j.earscirev.2021.103551. Bibcode: 2021ESRv..21503551V.
- ↑ 104.0 104.1 von der Heydt, Anna; Dijkstra, Henk A. (May 2008). "The effect of gateways on ocean circulation patterns in the Cenozoic". Global and Planetary Change. 1–2 62 (1–2): 132–146. doi:10.1016/j.gloplacha.2007.11.006. Bibcode: 2008GPC....62..132V.
- ↑ Allen, Mark; Armstrong, Howard (July 2008). "Arabia-Eurasia cooling and the forcing of mid-Cenozoic global cooling". Palaeogeology, Palaeoclimatology, Palaeoecology. 1–2 265 (1–2): 52–58. doi:10.1016/j.palaeo.2008.04.021. http://dro.dur.ac.uk/14557/1/14557.pdf.
- ↑ Green, William; Hunt, G.; Wing, S.; DiMichele, W. (2011). "Does extinction wield an axe or pruning shears? How interactions between phylogeny and ecology affect patterns of extinction". Paleobiology 37 (1): 72–91. doi:10.1666/09078.1. Bibcode: 2011Pbio...37...72G.
- ↑ Bosellini, Francesca; Perrin, Christine (February 2008). "Estimating Mediterranean Oligocene–Miocene sea surface temperatures: An approach based on coral taxonomic richness". Palaeogeography, Palaeoclimatology, Palaeoecology. 1–2 258 (1–2): 71–88. doi:10.1016/j.palaeo.2007.10.028. Bibcode: 2008PPP...258...71B.
- ↑ Hay, William; Flogel, S.; Soding, E. (September 2004). "Is initiation of glaciation on Antarctica related to a change in the structure of the ocean?". Global and Planetary Change. 1–3 45 (1–3): 23–33. doi:10.1016/j.gloplacha.2004.09.005. Bibcode: 2005GPC....45...23H.
- ↑ "Haughton". Earth Impact Database. Planetary and Space Science Centre University of New Brunswick Fredericton. http://www.passc.net/EarthImpactDatabase/New%20website_05-2018/Haughton.html.
- ↑ Sherlock, S.C. et al. (2005). "Re-evaluating the age of the Haughton impact event". Meteoritics & Planetary Science 40 (12): 1777–1787. doi:10.1111/j.1945-5100.2005.tb00146.x. Bibcode: 2005M&PS...40.1777S.
- ↑ Breining, Greg (2007). "Most-Super Volcanoes". Super Volcano: The Ticking Time Bomb Beneath Yellowstone National Park. St. Paul, MN: Voyageur Press. pp. 256 pg. ISBN 978-0-7603-2925-2. https://archive.org/details/supervolcanotick0000brei/page/256.
- Ogg, Jim (June 2004). "Overview of Global Boundary Stratotype Sections and Points (GSSP's)". International Commission on Stratigraphy. Accessed April 30, 2006.
External links
![]() |
Wikisource has the text of the 1911 Encyclopædia Britannica article Oligocene System. |
- Palaeos: Oligocene
- UCMP Berkeley Oligocene Page
- Prehistoric Pictures, in the Public Domain
- Oligocene Leaf Fossils
- Olicgocene Fish Fossils
- PaleoMap Project: Oligocene
- Oligocene Microfossils: 300+ images of Foraminifera
![]() | Original source: https://en.wikipedia.org/wiki/Oligocene.
Read more |