Earth:Eocene
Eocene | |
---|---|
56.0 – 33.9 Ma | |
![]() Map of the Earth in the early Eocene (50 Ma) | |
Chronology | |
Etymology | |
Name formality | Formal |
Usage information | |
Celestial body | Earth |
Regional usage | Global (ICS) |
Time scale(s) used | ICS Time Scale |
Definition | |
Chronological unit | Epoch |
Stratigraphic unit | Series |
Time span formality | Formal |
Lower boundary definition | Strong negative anomaly in δ13C values at the PETM[3] |
Lower boundary GSSP | Dababiya section, Luxor, Egypt[3] [ ⚑ ] 25°30′00″N 32°31′52″E / 25.5000°N 32.5311°E |
GSSP ratified | 2003[3] |
Upper boundary definition | LAD of Planktonic Foraminifers Hantkenina and Cribrohantkenina |
Upper boundary GSSP | Massignano quarry section, Massignano, Ancona, Italy [ ⚑ ] 43°31′58″N 13°36′04″E / 43.5328°N 13.6011°E |
GSSP ratified | 1992[4] |
The Eocene (IPA: /ˈiːəsiːn, ˈiːoʊ-/ EE-ə-seen, EE-oh-[5][6]) Epoch is a geological epoch that lasted from about 56 to 33.9 million years ago (Ma). It is the second epoch of the Paleogene Period in the modern Cenozoic Era. The name Eocene comes from the Ancient Greek ἠώς (ēṓs, "dawn") and καινός (kainós, "new") and refers to the "dawn" of modern ('new') fauna that appeared during the epoch.[7][8]
The Eocene spans the time from the end of the Paleocene Epoch to the beginning of the Oligocene Epoch. The start of the Eocene is marked by a brief period in which the concentration of the carbon isotope 13C in the atmosphere was exceptionally low in comparison with the more common isotope 12C. The average temperature of Earth in the beginning of the Eocene was about 27 degrees Celsius.[9] The end is set at a major extinction event called the Grande Coupure (the "Great Break" in continuity) or the Eocene–Oligocene extinction event, which may be related to the impact of one or more large bolides in Siberia and in what is now Chesapeake Bay. As with other geologic periods, the strata that define the start and end of the epoch are well identified,[10] though their exact dates are slightly uncertain.
Etymology
The term "Eocene" is derived from Ancient Greek ἠώς eos meaning "dawn", and καινός kainos meaning "new" or "recent", as the epoch saw the dawn of recent, or modern, life.
Scottish geologist Charles Lyell (ignoring the Quaternary) divided the Tertiary Epoch into the Eocene, Miocene, Pliocene, and New Pliocene (Holocene) Periods in 1833.[11][n 1] British geologist John Phillips proposed the Cenozoic in 1840 in place of the Tertiary,[12] and Austrian paleontologist Moritz Hörnes introduced the Paleogene for the Eocene and Neogene for the Miocene and Pliocene in 1853.[13] After decades of inconsistent usage, the newly formed International Commission on Stratigraphy (ICS), in 1969, standardized stratigraphy based on the prevailing opinions in Europe: the Cenozoic Era subdivided into the Tertiary and Quaternary sub-eras, and the Tertiary subdivided into the Paleogene and Neogene periods.[14] In 1978, the Paleogene was officially defined as the Paleocene, Eocene, and Oligocene epochs; and the Neogene as the Miocene and Pliocene epochs.[15] In 1989, Tertiary and Quaternary were removed from the time scale due to the arbitrary nature of their boundary, but Quaternary was reinstated in 2009.[16]
Geology
Boundaries
The Eocene is a dynamic epoch that represents global climatic transitions between two climatic extremes, transitioning from the hot house to the cold house. The beginning of the Eocene is marked by the Paleocene–Eocene Thermal Maximum, a short period of intense warming and ocean acidification brought about by the release of carbon en masse into the atmosphere and ocean systems,[17] which led to a mass extinction of 30–50% of benthic foraminifera (single-celled species which are used as bioindicators of the health of a marine ecosystem)—one of the largest in the Cenozoic.[18][19] This event happened around 55.8 Ma, and was one of the most significant periods of global change during the Cenozoic.[17][20][21]
The middle Eocene was characterized by the shift towards a cooler climate at the end of the EECO, around 47.8 Ma, which was briefly interrupted by another warming event called the middle Eocene climatic optimum (MECO).[22] Lasting for about 400,000 years, the MECO was responsible for a globally uniform 4° to 6°C warming of both the surface and deep oceans, as inferred from foraminiferal stable oxygen isotope records. The resumption of a long-term gradual cooling trend resulted in a glacial maximum at the late Eocene/early Oligocene boundary.
The end of the Eocene was also marked by the Eocene–Oligocene extinction event, also known as the Grande Coupure.[23]
Stratigraphy
The Eocene is conventionally divided into early (56–47.8 Ma), middle (47.8–38 Ma), and late (38–33.9 Ma) subdivisions.[24] The corresponding rocks are referred to as lower, middle, and upper Eocene. The Ypresian Stage constitutes the lower, the Priabonian Stage the upper; and the Lutetian and Bartonian stages are united as the middle Eocene.[citation needed]
Palaeogeography and tectonics
During the Eocene, the continents continued to drift toward their present positions.
At the beginning of the period, Australia and Antarctica remained connected, and warm equatorial currents may have mixed with colder Antarctic waters, distributing the heat around the planet and keeping global temperatures high. When Australia split from the southern continent around 45 Ma, the warm equatorial currents were routed away from Antarctica. An isolated cold water channel developed between the two continents.[25] However, modeling results call into question the thermal isolation model for late Eocene cooling,[26] and decreasing carbon dioxide levels in the atmosphere may have been more important. Once the Antarctic region began to cool down, the ocean surrounding Antarctica began to freeze, sending cold water and icefloes north and reinforcing the cooling.[27]
The northern supercontinent of Laurasia began to fragment, as Europe, Greenland and North America drifted apart.[28]
In western North America, the Laramide Orogeny came to an end in the Eocene, and compression was replaced with crustal extension that ultimately gave rise to the Basin and Range Province.[29][30] The Kishenehn Basin, around 1.5 km in elevation during the Lutetian, was uplifted to an altitude of 2.5 km by the Priabonian.[31] Huge lakes formed in the high flat basins among uplifts,[32] resulting in the deposition of the Green River Formation lagerstätte.[33]
At about 35 Ma, an asteroid impact on the eastern coast of North America formed the Chesapeake Bay impact crater.[34][35]
The Tethys Ocean finally closed with the collision of Africa and Eurasia,[36] while the uplift of the Alps isolated its final remnant, the Mediterranean, and created another shallow sea with island archipelagos to the north.[37] Planktonic foraminifera in the northwestern Peri-Tethys are very similar to those of the Tethys in the middle Lutetian but become completely disparate in the Bartonian, indicating biogeographic separation.[38] Though the North Atlantic was opening,[39] a land connection appears to have remained between North America and Europe since the faunas of the two regions are very similar.[40]
Eurasia was separated in three different landmasses 50 Ma; Western Europe, Balkanatolia and Asia. About 40 Ma, Balkanatolia and Asia were connected, while Europe was connected 34 Ma.[41][42] The Fushun Basin contained large, suboxic lakes known as the paleo-Jijuntun Lakes.[43]
India collided with Asia, folding to initiate formation of the Himalaya s.[44] The incipient subcontinent collided with the Kohistan–Ladakh Arc around 50.2 Ma and with Karakoram around 40.4 Ma, with the final collision between Asia and India occurring ~40 Ma.[45][46]
Climate
The Eocene Epoch contained a wide variety of different climate conditions that includes the warmest climate in the Cenozoic Era, and arguably the warmest time interval since the Permian-Triassic mass extinction and Early Triassic, and ends in an icehouse climate.[47] The evolution of the Eocene climate began with warming after the end of the Paleocene–Eocene Thermal Maximum (PETM) at 56 Ma to a maximum during the Eocene Optimum at around 49 Ma. During this period of time, little to no ice was present on Earth with a smaller difference in temperature from the equator to the poles.[48] Because of this the maximum sea level was 150 meters higher than current levels.[49] Following the maximum was a descent into an icehouse climate from the Eocene Optimum to the Eocene–Oligocene transition at 34 Ma. During this decrease, ice began to reappear at the poles, and the Eocene–Oligocene transition is the period of time when the Antarctic ice sheet began to rapidly expand.[50]
Early Eocene
Greenhouse gases, in particular carbon dioxide and methane, played a significant role during the Eocene in controlling the surface temperature. The end of the PETM was met with very large sequestration of carbon dioxide into the forms of methane clathrate, coal, and crude oil at the bottom of the Arctic Ocean, that reduced the atmospheric carbon dioxide.[51] This event was similar in magnitude to the massive release of greenhouse gasses at the beginning of the PETM, and it is hypothesized that the sequestration was mainly due to organic carbon burial and weathering of silicates. For the early Eocene there is much discussion on how much carbon dioxide was in the atmosphere. This is due to numerous proxies representing different atmospheric carbon dioxide content. For example, diverse geochemical and paleontological proxies indicate that at the maximum of global warmth the atmospheric carbon dioxide values were at 700–900 ppm,[52] while model simulations suggest a concentration of 1,680 ppm fits best with deep sea, sea surface, and near-surface air temperatures of the time.[53] Other proxies such as pedogenic (soil building) carbonate and marine boron isotopes indicate large changes of carbon dioxide of over 2,000 ppm over periods of time of less than 1 million years.[54] This large influx of carbon dioxide could be attributed to volcanic out-gassing due to North Atlantic rifting or oxidation of methane stored in large reservoirs deposited from the PETM event in the sea floor or wetland environments.[52] For contrast, today the carbon dioxide levels are at 400 ppm or 0.04%.
During the early Eocene, methane was another greenhouse gas that had a drastic effect on the climate. Methane has 30 times more of a warming effect than carbon dioxide on a 100-year scale (i.e., methane has a global warming potential of 29.8±11).[55] Most of the methane released to the atmosphere during this period of time would have been from wetlands, swamps, and forests.[56] The atmospheric methane concentration today is 0.000179% or 1.79 ppmv. As a result of the warmer climate and the sea level rise associated with the early Eocene, more wetlands, more forests, and more coal deposits would have been available for methane release. If we compare the early Eocene production of methane to current levels of atmospheric methane, the early Eocene would have produced triple the amount of methane. The warm temperatures during the early Eocene could have increased methane production rates, and methane that is released into the atmosphere would in turn warm the troposphere, cool the stratosphere, and produce water vapor and carbon dioxide through oxidation. Biogenic production of methane produces carbon dioxide and water vapor along with the methane, as well as yielding infrared radiation. The breakdown of methane in an atmosphere containing oxygen produces carbon monoxide, water vapor and infrared radiation. The carbon monoxide is not stable, so it eventually becomes carbon dioxide and in doing so releases yet more infrared radiation. Water vapor traps more infrared than does carbon dioxide. At about the beginning of the Eocene Epoch (55.8–33.9 Ma) the amount of oxygen in the Earth's atmosphere more or less doubled.[57]
During the warming in the early Eocene between 55 and 52 Ma, there were a series of short-term changes of carbon isotope composition in the ocean.[58][59] These isotope changes occurred due to the release of carbon from the ocean into the atmosphere that led to a temperature increase of 4–8 °C (7.2–14.4 °F) at the surface of the ocean. Recent analysis of and research into these hyperthermals in the early Eocene has led to hypotheses that the hyperthermals are based on orbital parameters, in particular eccentricity and obliquity. The hyperthermals in the early Eocene, notably the Palaeocene–Eocene Thermal Maximum (PETM), the Eocene Thermal Maximum 2 (ETM2), and the Eocene Thermal Maximum 3 (ETM3), were analyzed and found that orbital control may have had a role in triggering the ETM2 and ETM3.[60][61][62] An enhancement of the biological pump proved effective at sequestering excess carbon during the recovery phases of these hyperthermals.[63] These hyperthermals led to increased perturbations in planktonic and benthic foraminifera,[64][65] with a higher rate of fluvial sedimentation as a consequence of the warmer temperatures.[66] Unlike the PETM, the lesser hyperthermals of the Early Eocene had negligible consequences for terrestrial mammals.[67] These Early Eocene hyperthermals produced a sustained period of extremely hot climate known as the Early Eocene Climatic Optimum (EECO).[68] During the early and middle EECO, the superabundance of the euryhaline dinocyst Homotryblium in New Zealand indicates elevated ocean salinity in the region.[69]
Equable climate problem
One of the unique features of the Eocene's climate as mentioned before was the equable and homogeneous climate that existed in the early parts of the Eocene. A multitude of proxies support the presence of a warmer equable climate being present during this period of time. A few of these proxies include the presence of fossils native to warm climates, such as crocodiles, located in the higher latitudes,[70][71] the presence in the high latitudes of frost-intolerant flora such as palm trees which cannot survive during sustained freezes,[71][72] and fossils of snakes found in the tropics that would require much higher average temperatures to sustain them.[71] TEX86 BAYSPAR measurements indicate extremely high sea surface temperatures of 40 °C (104 °F) to 45 °C (113 °F) at low latitudes,[73] although clumped isotope analyses point to a maximum low latitude sea surface temperature of 36.3 °C (97.3 °F) ± 1.9 °C (35.4 °F) during the EECO.[74] Relative to present-day values, bottom water temperatures are 10 °C (18 °F) higher according to isotope proxies.[72] With these bottom water temperatures, temperatures in areas where deep water forms near the poles are unable to be much cooler than the bottom water temperatures.[citation needed]
An issue arises, however, when trying to model the Eocene and reproduce the results that are found with the proxy data.[75] Using all different ranges of greenhouse gasses that occurred during the early Eocene, models were unable to produce the warming that was found at the poles and the reduced seasonality that occurs with winters at the poles being substantially warmer. The models, while accurately predicting the tropics, tend to produce significantly cooler temperatures of up to 20 °C (36 °F) colder than the actual determined temperature at the poles.[72] This error has been classified as the "equable climate problem". To solve this problem, the solution would involve finding a process to warm the poles without warming the tropics. Some hypotheses and tests which attempt to find the process are listed below.[citation needed]
Large lakes
Due to the nature of water as opposed to land, less temperature variability would be present if a large body of water is also present. In an attempt to try to mitigate the cooling polar temperatures, large lakes were proposed to mitigate seasonal climate changes.[76] To replicate this case, a lake was inserted into North America and a climate model was run using varying carbon dioxide levels. The model runs concluded that while the lake did reduce the seasonality of the region greater than just an increase in carbon dioxide, the addition of a large lake was unable to reduce the seasonality to the levels shown by the floral and faunal data.[citation needed]
Ocean heat transport
The transport of heat from the tropics to the poles, much like how ocean heat transport functions in modern times, was considered a possibility for the increased temperature and reduced seasonality for the poles.[77] With the increased sea surface temperatures and the increased temperature of the deep ocean water during the early Eocene, one common hypothesis was that due to these increases there would be a greater transport of heat from the tropics to the poles. Simulating these differences, the models produced lower heat transport due to the lower temperature gradients and were unsuccessful in producing an equable climate from only ocean heat transport.[citation needed]
Orbital parameters
While typically seen as a control on ice growth and seasonality, the orbital parameters were theorized as a possible control on continental temperatures and seasonality.[78] Simulating the Eocene by using an ice free planet, eccentricity, obliquity, and precession were modified in different model runs to determine all the possible different scenarios that could occur and their effects on temperature. One particular case led to warmer winters and cooler summer by up to 30% in the North American continent, and it reduced the seasonal variation of temperature by up to 75%. While orbital parameters did not produce the warming at the poles, the parameters did show a great effect on seasonality and needed to be considered.[citation needed]
Polar stratospheric clouds
Another method considered for producing the warm polar temperatures were polar stratospheric clouds.[79] Polar stratospheric clouds are clouds that occur in the lower stratosphere at very low temperatures. Polar stratospheric clouds have a great impact on radiative forcing. Due to their minimal albedo properties and their optical thickness, polar stratospheric clouds act similar to a greenhouse gas and traps outgoing longwave radiation. Different types of polar stratospheric clouds occur in the atmosphere: polar stratospheric clouds that are created due to interactions with nitric or sulfuric acid and water (Type I) or polar stratospheric clouds that are created with only water ice (Type II).[citation needed]
Methane is an important factor in the creation of the primary Type II polar stratospheric clouds that were created in the early Eocene.[56] Since water vapor is the only supporting substance used in Type II polar stratospheric clouds, the presence of water vapor in the lower stratosphere is necessary where in most situations the presence of water vapor in the lower stratosphere is rare. When methane is oxidized, a significant amount of water vapor is released. Another requirement for polar stratospheric clouds is cold temperatures to ensure condensation and cloud production. Polar stratospheric cloud production, since it requires the cold temperatures, is usually limited to nighttime and winter conditions. With this combination of wetter and colder conditions in the lower stratosphere, polar stratospheric clouds could have formed over wide areas in Polar Regions.[citation needed]
To test the polar stratospheric clouds effects on the Eocene climate, models were run comparing the effects of polar stratospheric clouds at the poles to an increase in atmospheric carbon dioxide.[79] The polar stratospheric clouds had a warming effect on the poles, increasing temperatures by up to 20 °C in the winter months. A multitude of feedbacks also occurred in the models due to the polar stratospheric clouds' presence. Any ice growth was slowed immensely and would lead to any present ice melting. Only the poles were affected with the change in temperature and the tropics were unaffected, which with an increase in atmospheric carbon dioxide would also cause the tropics to increase in temperature. Due to the warming of the troposphere from the increased greenhouse effect of the polar stratospheric clouds, the stratosphere would cool and would potentially increase the amount of polar stratospheric clouds.
While the polar stratospheric clouds could explain the reduction of the equator to pole temperature gradient and the increased temperatures at the poles during the early Eocene, there are a few drawbacks to maintaining polar stratospheric clouds for an extended period of time. Separate model runs were used to determine the sustainability of the polar stratospheric clouds.[80] It was determined that in order to maintain the lower stratospheric water vapor, methane would need to be continually released and sustained. In addition, the amounts of ice and condensation nuclei would need to be high in order for the polar stratospheric cloud to sustain itself and eventually expand.[citation needed]
Middle Eocene
The Eocene is not only known for containing the warmest period during the Cenozoic; it also marked the decline into an icehouse climate and the rapid expansion of the Antarctic ice sheet. The transition from a warming climate into a cooling climate began at around 49 Ma. Isotopes of carbon and oxygen indicate a shift to a global cooling climate.[81] The cause of the cooling has been attributed to a significant decrease of >2,000 ppm in atmospheric carbon dioxide concentrations.[52] One proposed cause of the reduction in carbon dioxide during the warming to cooling transition was the azolla event. With the equable climate during the early Eocene, warm temperatures in the arctic allowed for the growth of azolla, which is a floating aquatic fern, on the Arctic Ocean. The significantly high amounts of carbon dioxide also acted to facilitate azolla blooms across the Arctic Ocean. Compared to current carbon dioxide levels, these azolla grew rapidly in the enhanced carbon dioxide levels found in the early Eocene.[81] The isolation of the Arctic Ocean, evidenced by euxinia that occurred at this time,[82] led to stagnant waters and as the azolla sank to the sea floor, they became part of the sediments on the seabed and effectively sequestered the carbon by locking it out of the atmosphere for good. The ability for the azolla to sequester carbon is exceptional, and the enhanced burial of azolla could have had a significant effect on the world atmospheric carbon content and may have been the event to begin the transition into an ice house climate.[81] The azolla event could have led to a draw down of atmospheric carbon dioxide of up to 470 ppm. Assuming the carbon dioxide concentrations were at 900 ppmv prior to the Azolla Event they would have dropped to 430 ppmv, or 30 ppmv more than they are today, after the Azolla Event.[81] This cooling trend at the end of the EECO has also been proposed to have been caused by increased siliceous plankton productivity and marine carbon burial, which also helped draw carbon dioxide out of the atmosphere.[52] Cooling after this event, part of a trend known as the Middle-Late Eocene Cooling (MLEC),[83] continued due to continual decrease in atmospheric carbon dioxide from organic productivity and weathering from mountain building.[84] Many regions of the world became more arid and cold over the course of the stage, such as the Fushun Basin.[43] In East Asia, lake level changes were in sync with global sea level changes over the course of the MLEC.[85]
Global cooling continued until there was a major reversal from cooling to warming in the Bartonian. This warming event, signifying a sudden and temporary reversal of the cooling conditions, is known as the Middle Eocene Climatic Optimum (MECO).[86][87] At around 41.5 Ma, stable isotopic analysis of samples from Southern Ocean drilling sites indicated a warming event for 600,000 years.[84] A similar shift in carbon isotopes is known from the Northern Hemisphere in the Scaglia Limestones of Italy.[86] Oxygen isotope analysis showed a large negative change in the proportion of heavier oxygen isotopes to lighter oxygen isotopes, which indicates an increase in global temperatures.[88] The warming is considered to be primarily due to carbon dioxide increases, because carbon isotope signatures rule out major methane release during this short-term warming.[84] A sharp increase in atmospheric carbon dioxide was observed with a maximum of 4,000 ppm: the highest amount of atmospheric carbon dioxide detected during the Eocene.[89] Other studies suggest a more modest rise in carbon dioxide levels.[90] The increase in atmospheric carbon dioxide has also been hypothesised to have been driven by increased seafloor spreading rates and metamorphic decarbonation reactions between Australia and Antarctica and increased amounts of volcanism in the region. One possible cause of atmospheric carbon dioxide increase could have been a sudden increase due to metamorphic release due to continental drift and collision of India with Asia and the resulting formation of the Himalayas; however, data on the exact timing of metamorphic release of atmospheric carbon dioxide is not well resolved in the data.[84] Recent studies have mentioned, however, that the removal of the ocean between Asia and India could have released significant amounts of carbon dioxide.[89] Another hypothesis still implicates a diminished negative feedback of silicate weathering as a result of continental rocks having become less weatherable during the warm Early and Middle Eocene, allowing volcanically released carbon dioxide to persist in the atmosphere for longer.[91] Yet another explanation hypothesises that MECO warming was caused by the simultaneous occurrence of minima in both the 400 kyr and 2.4 Myr eccentricity cycles.[92] During the MECO, sea surface temperatures in the Tethys Ocean jumped to 32–36 °C,[93] and Tethyan seawater became more dysoxic.[94] A decline in carbonate accumulation at ocean depths of greater than three kilometres took place synchronously with the peak of the MECO, signifying ocean acidification took place in the deep ocean.[95] On top of that, MECO warming caused an increase in the respiration rates of pelagic heterotrophs, leading to a decreased proportion of primary productivity making its way down to the seafloor and causing a corresponding decline in populations of benthic foraminifera.[96] An abrupt decrease in lakewater salinity in western North America occurred during this warming interval.[97] This warming is short lived, as benthic oxygen isotope records indicate a return to cooling at ~40 Ma.[98]
Late Eocene
At the end of the MECO, the MLEC resumed.[83] Cooling and the carbon dioxide drawdown continued through the late Eocene and into the Eocene–Oligocene transition around 34 Ma.[99] The post-MECO cooling brought with it a major aridification trend in Asia,[100] enhanced by retreating seas.[101] The cooling during the initial stages of the opening of the Drake Passage ~38.5 Ma was not global, as evidenced by an absence of cooling in the North Atlantic.[102] During the cooling period, benthic oxygen isotopes show the possibility of ice creation and ice increase during this later cooling.[52] The end of the Eocene and beginning of the Oligocene is marked with the massive expansion of area of the Antarctic ice sheet that was a major step into the icehouse climate.[103] Multiple proxies, such as oxygen isotopes and alkenones, indicate that at the Eocene–Oligocene transition, the atmospheric carbon dioxide concentration had decreased to around 750–800 ppm, approximately twice that of present levels.[98][103] Along with the decrease of atmospheric carbon dioxide reducing the global temperature, orbital factors in ice creation can be seen with 100,000-year and 400,000-year fluctuations in benthic oxygen isotope records.[104] Another major contribution to the expansion of the ice sheet was the creation of the Antarctic Circumpolar Current.[105] The creation of the Antarctic circumpolar current would isolate the cold water around the Antarctic, which would reduce heat transport to the Antarctic[106] along with creating ocean gyres that result in the upwelling of colder bottom waters.[105] The issue with this hypothesis of the consideration of this being a factor for the Eocene-Oligocene transition is the timing of the creation of the circulation is uncertain.[107] For Drake Passage, sediments indicate the opening occurred ~41 Ma while tectonics indicate that this occurred ~32 Ma.[citation needed] Solar activity did not change significantly during the greenhouse-icehouse transition across the Eocene-Oligocene boundary.[108]
Flora
During the early-middle Eocene, forests covered most of the Earth including the poles. Tropical forests extended across much of modern Africa, South America, Central America, India, South-east Asia and China. Paratropical forests grew over North America, Europe and Russia, with broad-leafed evergreen and broad-leafed deciduous forests at higher latitudes.[109]
Polar forests were quite extensive. Fossils and even preserved remains of trees such as swamp cypress and dawn redwood from the Eocene have been found on Ellesmere Island in the Arctic. Even at that time, Ellesmere Island was only a few degrees in latitude further south than it is today. Fossils of subtropical and even tropical trees and plants from the Eocene also have been found in Greenland and Alaska. Tropical rainforests grew as far north as northern North America and Europe.[citation needed]
Palm trees were growing as far north as Alaska and northern Europe during the early Eocene, although they became less abundant as the climate cooled.[110] Dawn redwoods were far more extensive as well.[111]
The earliest definitive Eucalyptus fossils were dated from 51.9 Ma, and were found in the Laguna del Hunco deposit in Chubut province in Argentina .[112]
Cooling began mid-period, and by the end of the Eocene continental interiors had begun to dry, with forests thinning considerably in some areas. The newly evolved grasses were still confined to river banks and lake shores, and had not yet expanded into plains and savannas.[citation needed]
The cooling also brought seasonal changes. Deciduous trees, better able to cope with large temperature changes, began to overtake evergreen tropical species.[113] By the end of the period, deciduous forests covered large parts of the northern continents, including North America, Eurasia and the Arctic, and rainforests held on only in equatorial South America, Africa, India and Australia .[citation needed]
Antarctica began the Eocene fringed with a warm temperate to sub-tropical rainforest. Pollen found in Prydz Bay from the Eocene suggest taiga forest existed there.[114] It became much colder as the period progressed; the heat-loving tropical flora was wiped out, and by the beginning of the Oligocene, the continent hosted deciduous forests and vast stretches of tundra.[citation needed]
Fauna
During the Eocene, plants and marine faunas became quite modern. Many modern bird orders first appeared in the Eocene. The Eocene oceans were warm and teeming with fish and other sea life.
Mammals
The oldest known fossils of most of the modern mammal orders appear within a brief period during the early Eocene. At the beginning of the Eocene, several new mammal groups arrived in North America. These modern mammals, like artiodactyls, perissodactyls, and primates, had features like long, thin legs, feet, and hands capable of grasping, as well as differentiated teeth adapted for chewing. Dwarf forms reigned. All the members of the new mammal orders were small, under 10 kg; based on comparisons of tooth size, Eocene mammals were only 60% of the size of the primitive Palaeocene mammals that preceded them. They were also smaller than the mammals that followed them. It is assumed that the hot Eocene temperatures favored smaller animals that were better able to manage the heat.[citation needed]
Both groups of modern ungulates (hoofed animals) became prevalent because of a major radiation between Europe and North America, along with carnivorous ungulates like Mesonyx. Early forms of many other modern mammalian orders appeared, including horses (most notably the Eohippus), bats, proboscidians (elephants), primates, and rodents. Older primitive forms of mammals declined in variety and importance. Important Eocene land fauna fossil remains have been found in western North America, Europe, Patagonia, Egypt, and southeast Asia. Marine fauna are best known from South Asia and the southeast United States.[citation needed]
Established megafauna of the Eocene include the Uintatherium, Arsinoitherium, and brontotheres, in which the former two, unlike the latter, did not belong to ungulates but groups that became extinct shortly after their establishments.
Large terrestrial mammalian predators began to take form as the terrestrial carnivores like the Hyaenodon and Daphoenus (the earliest lineage of a once-successful predatory family known as bear dogs). Entelodonts meanwhile established themselves as some of the largest omnivores. The first nimravids, including Dinictis, established themselves as amongst the first feliforms to appear. Their groups became highly successful and continued to live past the Eocene.
Basilosaurus is a very well-known Eocene whale, but whales as a group had become very diverse during the Eocene, which is when the major transitions from being terrestrial to fully aquatic in cetaceans occurred. The first sirenians were evolving at this time, and would eventually evolve into the extant manatees and dugongs.
It is thought that millions of years after the Cretaceous-Paleogene extinction event, brain sizes of mammals now started to increase, "likely driven by a need for greater cognition in increasingly complex environments".[115][116][clarification needed]
Birds
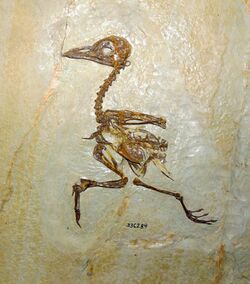
Eocene birds include some enigmatic groups with resemblances to modern forms, some of which continued from the Paleocene. Bird taxa of the Eocene include carnivorous psittaciforms, such as Messelasturidae, Halcyornithidae, large flightless forms such as Gastornis and Eleutherornis, long legged falcon Masillaraptor, ancient galliforms such as Gallinuloides, putative rail relatives of the family Songziidae, various pseudotooth birds such as Gigantornis, the ibis relative Rhynchaeites, primitive swifts of the genus Aegialornis, and primitive penguins such as Archaeospheniscus and Inkayacu.[citation needed]
Reptiles
Reptile fossils from this time, such as fossils of pythons and turtles, are abundant.[117]
Insects and arachnids
Several rich fossil insect faunas are known from the Eocene, notably the Baltic amber found mainly along the south coast of the Baltic Sea,[118] amber from the Paris Basin, France, the Fur Formation, Denmark , and the Bembridge Marls from the Isle of Wight, England. Insects found in Eocene deposits mostly belong to genera that exist today, though their range has often shifted since the Eocene. For instance the bibionid genus Plecia is common in fossil faunas from presently temperate areas, but only lives in the tropics and subtropics today.[citation needed]
Gallery
See also
- Bolca in Italy
- List of fossil sites (with link directory)
- London Clay
- Messel pit in Germany
- Wadi El Hitan in Egypt
Notes
- ↑ In Lyell's time, epochs were divided into periods. In modern geology, periods are divided into epochs.
References
- ↑ Zachos, J. C.; Kump, L. R. (2005). "Carbon cycle feedbacks and the initiation of Antarctic glaciation in the earliest Oligocene". Global and Planetary Change 47 (1): 51–66. doi:10.1016/j.gloplacha.2005.01.001. Bibcode: 2005GPC....47...51Z.
- ↑ "International Chronostratigraphic Chart". International Commission on Stratigraphy. https://stratigraphy.org/icschart/ChronostratChart2020-01.pdf.
- ↑ 3.0 3.1 3.2 Aubry, Marie-Pierre; Ouda, Khaled; Dupuis, Christian; William A. Berggren; John A. Van Couvering; Working Group on the Paleocene/Eocene Boundary (2007). "The Global Standard Stratotype-section and Point (GSSP) for the base of the Eocene Series in the Dababiya section (Egypt)". Episodes 30 (4): 271–286. doi:10.18814/epiiugs/2007/v30i4/003. http://www.stratigraphy.org/GSSP/file11.pdf.
- ↑ Silva, Isabella; Jenkins, D. (September 1993). "Decision on the Eocene-Oligocene boundary stratotype". Episodes 16 (3): 379–382. doi:10.18814/epiiugs/1993/v16i3/002. https://stratigraphy.org/gssps/files/rupelian.pdf. Retrieved 13 December 2020.
- ↑ Jones, Daniel (2003), Peter Roach; James Hartmann; Jane Setter, eds., English Pronouncing Dictionary, Cambridge: Cambridge University Press, ISBN 3-12-539683-2
- ↑ "Eocene". Merriam-Webster Dictionary. https://www.merriam-webster.com/dictionary/Eocene.
- ↑ See:
- Letter from William Whewell to Charles Lyell dated 31 January 1831 in: Todhunter, Isaac, ed (1876). William Whewell, D. D., Master of Trinity College, Cambridge: An account of his writings with selections from his literary and scientific correspondence. 2. London, England: Macmillan and Co.. p. 111. https://books.google.com/books?id=XaMKAAAAIAAJ&pg=PA111.
- Lyell, Charles (1833). Principles of Geology, …. 3. London, England: John Murray. p. 55. https://babel.hathitrust.org/cgi/pt?id=hvd.32044103125555;view=1up;seq=105. From p. 55: "The period next antecedent we shall call Eocene, from ήως, aurora, and χαινος, recens, because the extremely small proportion of living species contained in these strata, indicates what may be considered the first commencement, or dawn, of the existing state of the animate creation."
- ↑ "Eocene". Eocene. http://www.etymonline.com/index.php?term=Eocene&allowed_in_frame=0.
- ↑ https://www.pnas.org/doi/10.1073/pnas.1809600115#:~:text=During%20the%20Eocene%2C%20the%20warmest,(ppmv)%20(23).
- ↑ The extinction of the Hantkeninidae, a planktonic family of foraminifera became generally accepted as marking the Eocene-Oligocene boundary; in 1998 Massignano in Umbria, central Italy, was designated the Global Boundary Stratotype Section and Point (GSSP).
- ↑ Lyell, C. (1833). Principles of Geology. 3. Geological Society of London. p. 378. https://archive.org/details/in.ernet.dli.2015.45031.
- ↑ Phillips, J. (1840). "Palæozoic series". Penny Cyclopaedia of the Society for the Diffusion of Useful Knowledge. 17. London, England: Charles Knight and Co.. pp. 153–154. https://babel.hathitrust.org/cgi/pt?id=hvd.hn4zr7;view=1up;seq=162.
- ↑ Hörnes, M. (1853). "Mittheilungen an Professor Bronn gerichtet" (in de). Neues Jahrbuch für Mineralogie, Geognosie, Geologie und Petrefaktenkunde: 806–810. https://babel.hathitrust.org/cgi/pt?id=hvd.32044106271273;view=1up;seq=828.
- ↑ George, T. N.; Harland, W. B. (1969). "Recommendations on stratigraphical usage". Proceedings of the Geological Society of London 156 (1,656): 139–166.
- ↑ Odin, G. S.; Curry, D.; Hunziker, J. Z. (1978). "Radiometric dates from NW European glauconites and the Palaeogene time-scale". Journal of the Geological Society 135 (5): 481–497. doi:10.1144/gsjgs.135.5.0481. Bibcode: 1978JGSoc.135..481O.
- ↑ Knox, R. W. O.'B.; Pearson, P. N.; Barry, T. L. (2012). "Examining the case for the use of the Tertiary as a formal period or informal unit". Proceedings of the Geologists' Association 123 (3): 390–393. doi:10.1016/j.pgeola.2012.05.004. Bibcode: 2012PrGA..123..390K. http://discovery.ucl.ac.uk/1398932/2/1398932.pdf.
- ↑ 17.0 17.1 Turner, S. K.; Hull, P. M.; Ridgwell, A. (2017). "A probabilistic assessment of the rapidity of PETM onset". Nature Communications 8 (353): 353. doi:10.1038/s41467-017-00292-2. PMID 28842564. Bibcode: 2017NatCo...8..353K.
- ↑ Zhang, Q.; Willems, H.; Ding, L.; Xu, X. (2019). "Response of larger benthic foraminifera to the Paleocene–Eocene thermal maximum and the position of the Paleocene/Eocene boundary in the Tethyan shallow benthic zones: Evidence from south Tibet". Geological Society of America Bulletin 131 (1–2): 84–98. doi:10.1130/B31813.1. Bibcode: 2019GSAB..131...84Z.
- ↑ Kennet, J. P.; Stott, L. D. (1995). "Terminal Paleocene Mass Extinction in the Deep Sea: Association with Global Warming". Effects of Past Global Change on Life: Studies in Geophysics. National Academy of Sciences. https://www.ncbi.nlm.nih.gov/books/NBK231944/.
- ↑ Winguth, C.; Thomas, E. (2012). "Global decline in ocean ventilation, oxygenation, and productivity during the Paleocene–Eocene Thermal Maximum: Implications for the benthic extinction". Geology 40 (3): 263–266. doi:10.1130/G32529.1. Bibcode: 2012Geo....40..263W. https://www.researchgate.net/publication/228335391.
- ↑ Schmidt, G. A.; Shindell, D. T. (2003). "Atmospheric composition, radiative forcing, and climate change as a consequence of a massive methane release from gas hydrates". Paleoceanography and Paleoclimatology 18 (1): n/a. doi:10.1029/2002PA000757. Bibcode: 2003PalOc..18.1004S.
- ↑ Bijl, Peter K.; Houben, Alexander J. P.; Schouten, Stefan; Bohaty, Steven M.; Sluijs, Appy; Reichart, Gert-Jan; Sinninghe Damsté, Jaap S.; Brinkhuis, Henk (2010-11-05). "Transient Middle Eocene Atmospheric CO 2 and Temperature Variations" (in en). Science 330 (6005): 819–821. doi:10.1126/science.1193654. ISSN 0036-8075. PMID 21051636. Bibcode: 2010Sci...330..819B. https://www.science.org/doi/10.1126/science.1193654.
- ↑ Hooker, J.J.; Collinson, M.E.; Sille, N.P. (2004). "Eocene-Oligocene mammalian faunal turnover in the Hampshire Basin, UK: calibration to the global time scale and the major cooling event". Journal of the Geological Society 161 (2): 161–172. doi:10.1144/0016-764903-091. Bibcode: 2004JGSoc.161..161H. http://doc.rero.ch/record/13418/files/PAL_E228.pdf.
- ↑ Rafferty, John P., ed (2013). "Eocene Epoch". Britannica. https://www.britannica.com/science/Eocene-Epoch.
- ↑ Bijl, P. K.; Bendle, J. A. P.; Bohaty, S. M.; Pross, J.; Schouten, S.; Tauxe, L.; Stickley, C. E.; McKay, R. M. et al. (2013-06-11). "Eocene cooling linked to early flow across the Tasmanian Gateway". Proceedings of the National Academy of Sciences of the United States of America 110 (24): 9645–9650. doi:10.1073/pnas.1220872110. PMID 23720311. Bibcode: 2013PNAS..110.9645B.
- ↑ Huber, Matthew; Brinkhuis, Henk; Stickley, Catherine E.; Döös, Kristofer; Sluijs, Appy; Warnaar, Jeroen; Schellenberg, Stephen A.; Williams, Graham L. (December 2004). "Eocene circulation of the Southern Ocean: Was Antarctica kept warm by subtropical waters?: Did the East Australian Current Warm Antarctica?". Paleoceanography and Paleoclimatology 19 (4). doi:10.1029/2004PA001014. https://docs.lib.purdue.edu/cgi/viewcontent.cgi?article=1198&context=easpubs.
- ↑ Francis, J.E.; Marenssi, S.; Levy, R.; Hambrey, M.; Thorn, V.C.; Mohr, B.; Brinkhuis, H.; Warnaar, J. et al. (2008). "Chapter 8 From Greenhouse to Icehouse – The Eocene/Oligocene in Antarctica". Developments in Earth and Environmental Sciences 8: 309–368. doi:10.1016/S1571-9197(08)00008-6. ISBN 9780444528476.
- ↑ Torsvik, Trond H.; Cocks, L. Robin M. (2017). Earth history and palaeogeography. Cambridge, United Kingdom: Cambridge University Press. pp. 242, 251. ISBN 9781107105324.
- ↑ English, Joseph M.; Johnston, Stephen T. (September 2004). "The Laramide Orogeny: What Were the Driving Forces?". International Geology Review 46 (9): 833–838. doi:10.2747/0020-6814.46.9.833. Bibcode: 2004IGRv...46..833E.
- ↑ Bird, Peter (October 1998). "Kinematic history of the Laramide orogeny in latitudes 35°-49°N, western United States". Tectonics 17 (5): 780–801. doi:10.1029/98TC02698. Bibcode: 1998Tecto..17..780B.
- ↑ Fan, Majie; Constenius, Kurt N.; Phillips, Rachel F.; Dettman, David L. (17 March 2021). "Late Paleogene paleotopographic evolution of the northern Cordilleran orogenic front: Implications for demise of the orogen" (in en). Geological Society of America Bulletin 133 (11–12): 2549–2566. doi:10.1130/B35919.1. ISSN 0016-7606. https://pubs.geoscienceworld.org/gsa/gsabulletin/article/595423/Late-Paleogene-paleotopographic-evolution-of-the. Retrieved 11 September 2023.
- ↑ Bradley, W. H. (1930). "The varves and climate of the Green River epoch". U.S. Geological Survey Professional Paper. Professional Paper 158-E. doi:10.3133/pp158E.
- ↑ Grande, Lance (2001). "An Updated Review of the Fish Faunas from the Green River Formation, the World's Most Productive Freshwater Lagerstätten". Eocene Biodiversity. Topics in Geobiology. 18. pp. 1–38. doi:10.1007/978-1-4615-1271-4_1. ISBN 978-1-4613-5471-0.
- ↑ Gohn, G. S.; Koeberl, C.; Miller, K. G.; Reimold, W. U.; Browning, J. V.; Cockell, C. S.; Horton, J. W.; Kenkmann, T. et al. (2008-06-27). "Deep Drilling into the Chesapeake Bay Impact Structure". Science 320 (5884): 1740–1745. doi:10.1126/science.1158708. PMID 18583604. Bibcode: 2008Sci...320.1740G.
- ↑ Poag, C. Wylie (2004). The Chesapeake Bay Crater : Geology and Geophysics of a Late Eocene Submarine Impact Structure. Berlin, Heidelberg: Springer Berlin Heidelberg. ISBN 9783642189005.
- ↑ Frizon de Lamotte, Dominique; Raulin, Camille; Mouchot, Nicolas; Wrobel-Daveau, Jean-Christophe; Blanpied, Christian; Ringenbach, Jean-Claude (17 May 2011). "The southernmost margin of the Tethys realm during the Mesozoic and Cenozoic: Initial geometry and timing of the inversion processes" (in en). Tectonics 30 (3): 1–22. doi:10.1029/2010TC002691. ISSN 0278-7407. https://agupubs.onlinelibrary.wiley.com/doi/10.1029/2010TC002691. Retrieved 15 December 2023.
- ↑ Torsvik & Cocks 2017, pp. 242-245.
- ↑ Benyamovskiy, Vladimir Naumovich (January 2012). "A high resolution lutetian-Bartonian planktonic foraminiferal zonation in the Crimean-Caucasus region of the northeastern Peri-Tethys". Austrian Journal of Earth Sciences 105 (1): 117–128. https://www.researchgate.net/publication/286698149. Retrieved 24 September 2023.
- ↑ Torsvik & Cocks 2017, pp. 251.
- ↑ Denk, Thomas; Grímsson, Friðgeir; Zetter, Reinhard; Símonarson, Leifur A. (2011). "The Biogeographic History of Iceland – the North Atlantic Land Bridge Revisited". Late Cainozoic Floras of Iceland. Topics in Geobiology. 35. pp. 647–668. doi:10.1007/978-94-007-0372-8_12. ISBN 978-94-007-0371-1.
- ↑ Licht, Alexis; Métais, Grégoire; Coster, Pauline; İbilioğlu, Deniz; Ocakoğlu, Faruk; Westerweel, Jan; Mueller, Megan; Campbell, Clay et al. (2022-03-01). "Balkanatolia: The insular mammalian biogeographic province that partly paved the way to the Grande Coupure" (in en). Earth-Science Reviews 226: 103929. doi:10.1016/j.earscirev.2022.103929. ISSN 0012-8252. Bibcode: 2022ESRv..22603929L. https://www.sciencedirect.com/science/article/pii/S0012825222000137.
- ↑ CNRS (2022-03-01). "Balkanatolia: Forgotten Continent Discovered by Team of Paleontologists and Geologists" (in en-us). https://scitechdaily.com/balkanatolia-forgotten-continent-discovered-by-team-of-paleontologists-and-geologists/.
- ↑ 43.0 43.1 Xu, Sheng-Chuan; Liu, Zhao-Jun; Zhang, Pu; Boak, Jeremy M.; Liu, Rong; Meng, Qing-Tao (1 October 2016). "Characterization of depositional conditions for lacustrine oil shales in the Eocene Jijuntun Formation, Fushun Basin, NE China" (in en). International Journal of Coal Geology 167: 10–30. doi:10.1016/j.coal.2016.09.004. https://linkinghub.elsevier.com/retrieve/pii/S0166516216305262. Retrieved 24 September 2023.
- ↑ Ding, Huixia; Zhang, Zeming; Dong, Xin; Tian, Zuolin; Xiang, Hua; Mu, Hongchen; Gou, Zhengbin; Shui, Xinfang et al. (February 2016). "Early Eocene ( c . 50 Ma) collision of the Indian and Asian continents: Constraints from the North Himalayan metamorphic rocks, southeastern Tibet". Earth and Planetary Science Letters 435: 64–73. doi:10.1016/j.epsl.2015.12.006. Bibcode: 2016E&PSL.435...64D.
- ↑ Bouilhol, Pierre; Jagoutz, Oliver; Hanchar, John M.; Dudas, Francis O. (15 March 2013). "Dating the India–Eurasia collision through arc magmatic records". Earth and Planetary Science Letters 366: 163–175. doi:10.1016/j.epsl.2013.01.023. Bibcode: 2013E&PSL.366..163B. https://www.sciencedirect.com/science/article/abs/pii/S0012821X1300040X. Retrieved 25 December 2022.
- ↑ Rennie, Victoria C. F.; Paris, Guillaume; Sessions, Alex L.; Abramovich, Sigal; Turchyn, Alexandra V.; Adkins, Jess F. (13 August 2018). "Cenozoic record of δ34S in foraminiferal calcite implies an early Eocene shift to deep-ocean sulfide burial". Nature Geoscience 11 (10): 761–765. doi:10.1038/s41561-018-0200-y. Bibcode: 2018NatGe..11..761R. https://www.nature.com/articles/s41561-018-0200-y?error=cookies_not_supported&code=183907e9-3ff1-46b3-b05c-ebd9b695add3. Retrieved 26 December 2022.
- ↑ Cramwinckel, Margot J.; Huber, Matthew; Kocken, Ilja J.; Agnini, Claudia; Bijl, Peter K.; Bohaty, Steven M.; Frieling, Joost; Goldner, Aaron et al. (2 July 2018). "Synchronous tropical and polar temperature evolution in the Eocene". Nature 559 (7714): 382–386. doi:10.1038/s41586-018-0272-2. PMID 29967546. Bibcode: 2018Natur.559..382C. https://www.nature.com/articles/s41586-018-0272-2. Retrieved 21 September 2022.
- ↑ West, Christopher K.; Greenwood, David R.; Reichgelt, Tammo; Lowe, Alexander J.; Vachon, Janelle M.; Basinger, James F. (4 August 2020). "Paleobotanical proxies for early Eocene climates and ecosystems in northern North America from middle to high latitudes". Climate of the Past 16 (4): 1387–1410. doi:10.5194/cp-16-1387-2020. Bibcode: 2020CliPa..16.1387W. https://cp.copernicus.org/articles/16/1387/2020/. Retrieved 8 January 2023.
- ↑ Li, Fengyuan; Li, Shuqiang (2018-10-01). "Paleocene–Eocene and Plio–Pleistocene sea-level changes as "species pumps" in Southeast Asia: Evidence from Althepus spiders" (in en). Molecular Phylogenetics and Evolution 127: 545–555. doi:10.1016/j.ympev.2018.05.014. ISSN 1055-7903. PMID 29778723. https://www.sciencedirect.com/science/article/pii/S1055790317306802.
- ↑ Galeotti, Simone; Deconto, Robert; Naish, Timothy; Stocchi, Paolo; Florindo, Fabio; Pagani, Mark; Barrett, Peter; Bohaty, Steven M. et al. (10 March 2016). "Antarctic Ice Sheet variability across the Eocene-Oligocene boundary climate transition". Science 352 (6281): 76–80. doi:10.1126/science.aab0669. PMID 27034370. Bibcode: 2016Sci...352...76G. https://www.science.org/doi/10.1126/science.aab0669. Retrieved 17 March 2023.
- ↑ Bowen, J. G.; Zachos, J. C. (2010). "Rapid carbon sequestration at the termination of the Palaeocene-Eocene Thermal Maximum". Nature Geoscience 3 (12): 866–869. doi:10.1038/ngeo1014. Bibcode: 2010NatGe...3..866B.
- ↑ 52.0 52.1 52.2 52.3 52.4 Pearson, P. N.; Palmer, M. R. (2000). "Atmospheric carbon dioxide concentrations over the past 60 million years". Nature 406 (6797): 695–699. doi:10.1038/35021000. PMID 10963587. Bibcode: 2000Natur.406..695P.
- ↑ Goudsmit-Harzevoort, Barbara; Lansu, Angelique; Baatsen, Michiel L. J.; von der Heydt, Anna S.; de Winter, Niels J.; Zhang, Yurui; Abe-Ouchi, Ayako; de Boer, Agatha et al. (17 February 2023). "The Relationship Between the Global Mean Deep-Sea and Surface Temperature During the Early Eocene" (in en). Paleoceanography and Paleoclimatology 38 (3): 1–18. doi:10.1029/2022PA004532. ISSN 2572-4517. https://agupubs.onlinelibrary.wiley.com/doi/10.1029/2022PA004532. Retrieved 24 September 2023.
- ↑ Royer, Dana L.; Wing, Scott L.; Beerling, David J.; Jolley, David W.; Koch, Paul L.; Hickey1, Leo J.; Berner, Robert A. (22 June 2001). "Paleobotanical Evidence for Near Present-Day Levels of Atmospheric CO2 During Part of the Tertiary". Science 292 (5525): 2310–2313. doi:10.1126/science.292.5525.2310. PMID 11423657. Bibcode: 2001Sci...292.2310R.
- ↑ Forster, P.; Storelvmo, T.; Armour, K.; Collins, W. (2021). "Chapter 7: The Earth's energy budget, climate feedbacks, and climate sensitivity". IPCC AR6 WG1 2021. https://www.ipcc.ch/report/ar6/wg1/downloads/report/IPCC_AR6_WGI_Chapter_07.pdf.
- ↑ 56.0 56.1 Sloan, L. C.; Walker, C. G.; Moore, T. C. Jr.; Rea, D. K.; Zachos, J. C. (1992). "Possible methane-induced polar warming in the early Eocene". Nature 357 (6376): 1129–1131. doi:10.1038/357320a0. PMID 11536496. Bibcode: 1992Natur.357..320S.
- ↑ O'Neil, Dennis (2012). "The First Primates". http://anthro.palomar.edu/earlyprimates/early_2.htm.
- ↑ Galeotti, S.; Krishnan, Srinath; Pagani, Mark; Lanci, Luca; Gaudio, Alberto; Zachos, James C.; Monechi, Simonetta; Morelli, Guia et al. (2010). "Orbital chronology of Early Eocene hyperthermals from the Contessa Road section, central Italy". Earth and Planetary Science Letters 290 (1–2): 192–200. doi:10.1016/j.epsl.2009.12.021. Bibcode: 2010E&PSL.290..192G.
- ↑ Slotnick, Benjamin S.; Cickens, Gerald R.; Nicolo, Micah J.; Hollis, Christopher J.; Crampton, James S.; Zachos, James C.; Sluijs, Appy (11 May 2012). "Large-Amplitude Variations in Carbon Cycling and Terrestrial Weathering during the Latest Paleocene and Earliest Eocene: The Record at Mead Stream, New Zealand". The Journal of Geology 120 (5): 487–505. doi:10.1086/666743. https://www.journals.uchicago.edu/doi/abs/10.1086/666743. Retrieved 23 June 2023.
- ↑ Zachos, James C.; McCarren, Heather; Murphy, Brandon; Röhl, Ursula; Westerhold, Thomas (15 October 2010). "Tempo and scale of late Paleocene and early Eocene carbon isotope cycles: Implications for the origin of hyperthermals". Earth and Planetary Science Letters 299 (1–2): 242–249. doi:10.1016/j.epsl.2010.09.004. https://www.sciencedirect.com/science/article/abs/pii/S0012821X10005650. Retrieved 23 June 2023.
- ↑ Turner, Sandra Kirtland; Sexton, Philip D.; Charles, Christopher D.; Norris, Richard D. (7 September 2014). "Persistence of carbon release events through the peak of early Eocene global warmth". Nature Geoscience 7 (1): 748–751. doi:10.1038/ngeo2240. https://www.nature.com/articles/ngeo2240. Retrieved 22 June 2023.
- ↑ Sexton, Philip F.; Norris, Richard D.; Wilson, Paul A.; Pälike, Heiko; Westerhold, Thomas; Röhl, Ursula; Bolton, Clara T.; Gibbs, Samantha (16 March 2011). "Eocene global warming events driven by ventilation of oceanic dissolved organic carbon". Nature 471 (7338): 349–352. doi:10.1038/nature09826. PMID 21412336. https://www.nature.com/articles/nature09826. Retrieved 22 June 2023.
- ↑ Yasukawa, Kazutaka; Nakamura, Kentaro; Fujinaga, Koijiro; Ikehara, Minoru; Kato, Yasuhiro (12 September 2017). "Earth system feedback statistically extracted from the Indian Ocean deep-sea sediments recording Eocene hyperthermals". Scientific Reports 7 (1): 11304. doi:10.1038/s41598-017-11470-z. PMID 28900142.
- ↑ Khanolkar, Sonal; Saraswati, Pratul Kumar (1 July 2015). "Ecological Response of Shallow-Marine Foraminifera to Early Eocene Warming in Equatorial India". Journal of Foraminiferal Research 45 (3): 293–304. doi:10.2113/gsjfr.45.3.293. https://pubs.geoscienceworld.org/cushmanfoundation/jfr/article-abstract/45/3/293/295085/ECOLOGICAL-RESPONSE-OF-SHALLOW-MARINE-FORAMINIFERA?redirectedFrom=fulltext. Retrieved 23 June 2023.
- ↑ Stassen, Peter; Steurbaut, Etienne; Morsi, Abdel-Mohsen; Schulte, Peter; Speijer, Robert (1 May 2021). "Biotic impact of Eocene thermal maximum 2 in a shelf setting (Dababiya, Egypt)". Austrian Journal of Earth Sciences 109: 154–160. https://lirias.kuleuven.be/278683?limo=0. Retrieved 23 June 2023.
- ↑ Reinhardt, Lutz; Von Gosen, Werner; Lückge, Andreas; Blumenberg, Martin; Galloway, Jennifer M.; West, Christopher K.; Sudermann, Markus; Dolezych, Martina (7 January 2022). "Geochemical indications for the Paleocene-Eocene Thermal Maximum (PETM) and Eocene Thermal Maximum 2 (ETM-2) hyperthermals in terrestrial sediments of the Canadian Arctic". Geosphere 18 (1): 327–349. doi:10.1130/GES02398.1. https://pubs.geoscienceworld.org/gsa/geosphere/article/18/1/327/610709/Geochemical-indications-for-the-Paleocene-Eocene. Retrieved 23 June 2023.
- ↑ Abels, Hemmo A.; Clyde, William C.; Gingerich, Philip D.; Hilgen, Frederik J.; Fricke, Henry C.; Bowen, Gabriel J.; Lourens, Lucas J. (1 April 2012). "Terrestrial carbon isotope excursions and biotic change during Palaeogene hyperthermals". Nature Geoscience 5 (5): 326–329. doi:10.1038/ngeo1427. https://www.nature.com/articles/ngeo1427. Retrieved 22 June 2023.
- ↑ Slotnick, B. S.; Dickens, G. R.; Hollis, C. J.; Crampton, J. S.; Strong, C. Percy; Phillips, A. (17 Sep 2015). "The onset of the Early Eocene Climatic Optimum at Branch Stream, Clarence River valley, New Zealand". New Zealand Journal of Geology and Geophysics 58 (3): 262–280. doi:10.1080/00288306.2015.1063514.
- ↑ Crouch, E. M.; Shepherd, C. L.; Morgans, H. E. G.; Naafs, B. D. A.; Dallanave, E.; Phillips, A.; Hollis, C. J.; Pancost, R. D. (1 January 2020). "Climatic and environmental changes across the early Eocene climatic optimum at mid-Waipara River, Canterbury Basin, New Zealand". Earth-Science Reviews 200: 102961. doi:10.1016/j.earscirev.2019.102961. ISSN 0012-8252. https://www.sciencedirect.com/science/article/pii/S0012825219302892. Retrieved 11 September 2023.
- ↑ Sloan, L. C.; Rea, D. K. (1995). "Atmospheric carbon dioxide and early Eocene climate: a general circulation modeling sensitivity study". Palaeogeography, Palaeoclimatology, Palaeoecology 119 (3–4): 275–292. doi:10.1016/0031-0182(95)00012-7.
- ↑ 71.0 71.1 71.2 Huber, M. (2009). "Snakes tell a torrid tale". Nature 457 (7230): 669–671. doi:10.1038/457669a. PMID 19194439.
- ↑ 72.0 72.1 72.2 Huber, M.; Caballero, R. (2011). "The early Eocene equable climate problem revisited". Climate of the Past 7 (2): 603–633. doi:10.5194/cp-7-603-2011. Bibcode: 2011CliPa...7..603H. https://docs.lib.purdue.edu/cgi/viewcontent.cgi?article=1184&context=easpubs.
- ↑ Grossman, Ethan L.; Joachimski, Michael M. (27 May 2022). "Ocean temperatures through the Phanerozoic reassessed". Scientific Reports 12 (1): 8938. doi:10.1038/s41598-022-11493-1. PMID 35624298. Bibcode: 2022NatSR..12.8938G.
- ↑ Evans, David; Sagoo, Navjit; Renema, Willem; Cotton, Laura J.; Müller, Wolfgang; Todd, Jonathan A.; Saraswati, Pratul Kumar; Stassen, Peter et al. (22 January 2018). "Eocene greenhouse climate revealed by coupled clumped isotope-Mg/Ca thermometry". Proceedings of the National Academy of Sciences of the United States of America 115 (6): 1174–1179. doi:10.1073/pnas.1714744115. PMID 29358374. Bibcode: 2018PNAS..115.1174E.
- ↑ Sloan, L. C.; Barron, E. J. (1990). ""Equable" climates during Earth history?". Geology 18 (6): 489–492. doi:10.1130/0091-7613(1990)018<0489:ecdeh>2.3.co;2. Bibcode: 1990Geo....18..489C.
- ↑ Sloan, L. C. (1994). "Equable climates during the early Eocene: Significance of regional paleogeography for North American climate". Geology 22 (10): 881–884. doi:10.1130/0091-7613(1994)022<0881:ecdtee>2.3.co;2. Bibcode: 1994Geo....22..881C.
- ↑ Huber, M.; Sloan, L. C. (2001). "Heat transport, deeps waters, and thermal gradients: Coupled simulation of an Eocene Greenhouse Climate". Geophysical Research Letters 28 (18): 3481–3484. doi:10.1029/2001GL012943. Bibcode: 2001GeoRL..28.3481H.
- ↑ Sloan, L. C.; Morrill, C. (1998). "Orbital forcing and Eocene continental temperatures". Palaeogeography, Palaeoclimatology, Palaeoecology 144 (1–2): 21–35. doi:10.1016/s0031-0182(98)00091-1. Bibcode: 1998PPP...144...21S.
- ↑ 79.0 79.1 Sloan, L. C.; Pollard, D. (1998). "Polar stratospheric clouds: A high latitude warming mechanism in an ancient greenhouse world". Geophysical Research Letters 25 (18): 3517–3520. doi:10.1029/98gl02492. Bibcode: 1998GeoRL..25.3517S.
- ↑ Kirk-Davidoff, D. B.; Lamarque, J. F. (2008). "Maintenance of polar stratospheric clouds in a moist stratosphere". Climate of the Past 4 (1): 69–78. doi:10.5194/cp-4-69-2008. Bibcode: 2008CliPa...4...69K.
- ↑ 81.0 81.1 81.2 81.3 Speelman, E. N.; Van Kempen, M. M. L.; Barke, J.; Brinkhuis, H.; Reichart, G. J.; Smolders, A. J. P.; Roelofs, J. G. M.; Sangiorgi, F. et al. (27 March 2009). "The Eocene Arctic Azolla bloom: environmental conditions, productivity, and carbon drawdown". Geobiology 7 (2): 155–170. doi:10.1111/j.1472-4669.2009.00195.x. PMID 19323694. Bibcode: 2009Gbio....7..155S.
- ↑ Ogawa, Yusuke; Takahashi, Kozo; Yamanaka, Toshiro; Onodera, Jonaotaro (30 July 2009). "Significance of euxinic condition in the middle Eocene paleo-Arctic basin: A geochemical study on the IODP Arctic Coring Expedition 302 sediments". Earth and Planetary Science Letters 285 (1–2): 190–197. doi:10.1016/j.epsl.2009.06.011. Bibcode: 2009E&PSL.285..190O. https://www.sciencedirect.com/science/article/abs/pii/S0012821X09003446. Retrieved 6 April 2023.
- ↑ 83.0 83.1 Scotese, Christopher Robert; Song, Haijun; Mills, Benjamin J.W.; van der Meer, Douwe G. (April 2021). "Phanerozoic paleotemperatures: The earth's changing climate during the last 540 million years" (in en). Earth-Science Reviews 215: 103503. doi:10.1016/j.earscirev.2021.103503. https://linkinghub.elsevier.com/retrieve/pii/S0012825221000027. Retrieved 24 September 2023.
- ↑ 84.0 84.1 84.2 84.3 Bohaty, S. M.; Zachos, J. C. (2003). "Significant Southern Ocean warming event in the late middle Eocene". Geology 31 (11): 1017–1020. doi:10.1130/g19800.1. Bibcode: 2003Geo....31.1017B.
- ↑ Ma, Yiquan; Fan, Majie; Li, Mingsong; Ogg, James G.; Zhang, Chen; Feng, Jun; Zhou, Chunhua; Liu, Xiaofeng et al. (15 January 2023). "East Asian lake hydrology modulated by global sea-level variations in the Eocene warmhouse". Earth and Planetary Science Letters 602: 117925. doi:10.1016/j.epsl.2022.117925. ISSN 0012-821X. https://www.sciencedirect.com/science/article/pii/S0012821X22005611. Retrieved 24 September 2023.
- ↑ 86.0 86.1 Jovane, Luigi; Florindo, Fabio; Coccioni, Rodolfo; Marsili, Andrea; Monechi, Simonetta; Roberts, Andrew P.; Sprovieri, Mario (1 March 2007). "The middle Eocene climatic optimum event in the Contessa Highway section, Umbrian Apennines, Italy". Geological Society of America Bulletin 119 (3–4): 413–427. doi:10.1130/B25917.1. Bibcode: 2007GSAB..119..413J. https://pubs.geoscienceworld.org/gsa/gsabulletin/article-abstract/119/3-4/413/125390/The-middle-Eocene-climatic-optimum-event-in-the. Retrieved 18 May 2023.
- ↑ Shi, Juye; Jin, Zhijun; Liu, Quanyou; Zhang, Rui; Huang, Zhenkai (March 2019). "Cyclostratigraphy and astronomical tuning of the middle eocene terrestrial successions in the Bohai Bay Basin, Eastern China". Global and Planetary Change 174: 115–126. doi:10.1016/j.gloplacha.2019.01.001. Bibcode: 2019GPC...174..115S. https://www.sciencedirect.com/science/article/abs/pii/S0921818118300729. Retrieved 3 January 2023.
- ↑ Edgar, Kirsty M.; Wilson, P. A.; Sexton, P. F.; Gibbs, S. J.; Roberts, Andrew P.; Norris, R. D. (20 November 2010). "New biostratigraphic, magnetostratigraphic and isotopic insights into the Middle Eocene Climatic Optimum in low latitudes". Palaeogeography, Palaeoclimatology, Palaeoecology 297 (3–4): 670–682. doi:10.1016/j.palaeo.2010.09.016. Bibcode: 2010PPP...297..670E. https://www.sciencedirect.com/science/article/abs/pii/S0031018210005869. Retrieved 18 May 2023.
- ↑ 89.0 89.1 Pearson, P. N. (2010). "Increased Atmospheric CO2 During the Middle Eocene". Science 330 (6005): 763–764. doi:10.1126/science.1197894. PMID 21051620. Bibcode: 2010Sci...330..763P.
- ↑ Henehan, Michael J.; Edgar, Kirsty M.; Foster, Gavin L.; Penman, Donald E.; Hull, Pincelli M.; Greenop, Rosanna; Anagnostou, Eleni; Pearson, Paul N. (9 March 2020). "Revisiting the Middle Eocene Climatic Optimum "Carbon Cycle Conundrum" With New Estimates of Atmospheric pCO2 From Boron Isotopes". Paleoceanography and Paleoclimatology 35 (6). doi:10.1029/2019PA003713. Bibcode: 2020PaPa...35.3713H. https://agupubs.onlinelibrary.wiley.com/doi/full/10.1029/2019PA003713. Retrieved 18 May 2023.
- ↑ Van der Ploeg, Robin; Selby, David; Cramwinckel, Margot J.; Li, Yang; Bohaty, Steven M.; Middelburg, Jack J.; Sluijs, Appy (23 July 2018). "Middle Eocene greenhouse warming facilitated by diminished weathering feedback". Nature Communications 9 (1): 2877. doi:10.1038/s41467-018-05104-9. PMID 30038400. Bibcode: 2018NatCo...9.2877V.
- ↑ Giorgioni, Martino; Jovane, Luigi; Rego, Eric S.; Rodelli, Daniel; Frontalini, Fabrizio; Coccioni, Rodolfo; Catanzariti, Rita; Özcan, Ercan (27 June 2019). "Carbon cycle instability and orbital forcing during the Middle Eocene Climatic Optimum". Scientific Reports 9 (1): 9357. doi:10.1038/s41598-019-45763-2. PMID 31249387. Bibcode: 2019NatSR...9.9357G.
- ↑ Cramwinckel, Margot J.; Van der Ploeg, Robin; Van Helmond, Niels A. G. M.; Waarlo, Niels; Agnini, Claudia; Bijl, Peter K.; Van der Boon, Annique; Brinkhuis, Henk et al. (1 September 2022). "Deoxygenation and organic carbon sequestration in the Tethyan realm associated with the middle Eocene climatic optimum". Geological Society of America Bulletin 135 (5–6): 1280–1296. doi:10.1130/B36280.1. https://pubs.geoscienceworld.org/gsa/gsabulletin/article/135/5-6/1280/616563/Deoxygenation-and-organic-carbon-sequestration-in. Retrieved 18 May 2023.
- ↑ Spofforth, D. J. A.; Agnini, C.; Pälike, H.; Rio, D.; Fornaciari, E.; Giusberi, L.; Luciani, V.; Lanci, L. et al. (24 August 2010). "Organic carbon burial following the middle Eocene climatic optimum in the central western Tethys". Paleoceanography and Paleoclimatology 25 (3): 1–11. doi:10.1029/2009PA001738. Bibcode: 2010PalOc..25.3210S. https://agupubs.onlinelibrary.wiley.com/doi/full/10.1029/2009PA001738. Retrieved 18 May 2023.
- ↑ Bohaty, Steven M.; Zachos, James C.; Florindo, Fabio; Delaney, Margaret L. (9 May 2009). "Coupled greenhouse warming and deep-sea acidification in the middle Eocene". Paleoceanography and Paleoclimatology 24 (2): 1–16. doi:10.1029/2008PA001676. Bibcode: 2009PalOc..24.2207B. https://agupubs.onlinelibrary.wiley.com/doi/full/10.1029/2008PA001676. Retrieved 20 May 2023.
- ↑ Boscolo Galazzo, Flavia; Thomas, Ellen; Giusberti, Luca (1 January 2015). "Benthic foraminiferal response to the Middle Eocene Climatic Optimum (MECO) in the South-Eastern Atlantic (ODP Site 1263)" (in en). Palaeogeography, Palaeoclimatology, Palaeoecology 417: 432–444. doi:10.1016/j.palaeo.2014.10.004. https://www.sciencedirect.com/science/article/abs/pii/S003101821400491X. Retrieved 19 November 2023.
- ↑ Mulch, Andreas; Chamberlain, C. P.; Cosca, Michael A.; Teyssier, Christian; Methner, Katharina; Hren, Michael T.; Graham, Stephan A. (April 2015). "Rapid change in high-elevation precipitation patterns of western North America during the Middle Eocene Climatic Optimum (MECO)". American Journal of Science 315 (4): 317–336. doi:10.2475/04.2015.02. Bibcode: 2015AmJS..315..317M. https://www.ajsonline.org/content/315/4/317.short. Retrieved 18 May 2023.
- ↑ 98.0 98.1 Pagani, M.; Zachos, J. C.; Freeman, Katherine H.; Tipple, Brett; Bohaty, Stephen (2005). "Marked Decline in Atmospheric Carbon Dioxide Concentrations During the Paleogene". Science 309 (5734): 600–603. doi:10.1126/science.1110063. PMID 15961630. Bibcode: 2005Sci...309..600P.
- ↑ Cappelli, C.; Bown, P. R.; Westerhold, T.; Bohaty, S. M.; De Riu, M.; Loba, V.; Yamamoto, Y.; Agnini, C. (15 November 2019). "The Early to Middle Eocene Transition: An Integrated Calcareous Nannofossil and Stable Isotope Record From the Northwest Atlantic Ocean (Integrated Ocean Drilling Program Site U1410)". Paleoceanography and Paleoclimatology 34 (12): 1913–1930. doi:10.1029/2019PA003686. Bibcode: 2019PaPa...34.1913C. https://agupubs.onlinelibrary.wiley.com/doi/10.1029/2019PA003686. Retrieved 17 March 2023.
- ↑ Bosboom, Roderic E.; Abels, Hemmo A.; Hoorn, Carina; Van den Berg, Bas C. J.; Guo, Zhaojie; Dupont-Nivet, Guillaume (1 March 2014). "Aridification in continental Asia after the Middle Eocene Climatic Optimum (MECO)". Earth and Planetary Science Letters 389: 34–42. doi:10.1016/j.epsl.2013.12.014. Bibcode: 2014E&PSL.389...34B. https://www.sciencedirect.com/science/article/abs/pii/S0012821X13007206. Retrieved 18 May 2023.
- ↑ Bosboom, Roderic; Dupont-Nivet, Guillaume; Grothe, Arjen; Brinkhuis, Henk; Villa, Giuliana; Mandic, Oleg; Stoica, Marius; Kouwenhoven, Tanja et al. (1 June 2014). "Timing, cause and impact of the late Eocene stepwise sea retreat from the Tarim Basin (west China)". Palaeogeography, Palaeoclimatology, Palaeoecology 403: 101–118. doi:10.1016/j.palaeo.2014.03.035. ISSN 0031-0182. https://www.sciencedirect.com/science/article/pii/S0031018214001709. Retrieved 26 December 2023.
- ↑ Borrelli, Chiara; Cramer, Benjamin S.; Katz, Miriam E. (27 March 2014). "Bipolar Atlantic deepwater circulation in the middle-late Eocene: Effects of Southern Ocean gateway openings". Paleoceanography and Paleoclimatology 29 (4): 308–327. doi:10.1002/2012PA002444. Bibcode: 2014PalOc..29..308B. https://agupubs.onlinelibrary.wiley.com/doi/10.1002/2012PA002444. Retrieved 7 April 2023.
- ↑ 103.0 103.1 Lear, C. H.; Bailey, T. R.; Pearson, P.N.; Coxall, H. K.; Rosenthal, Y. (2008). "Cooling and ice growth across the Eocene-Oligocene transition". Geology 36 (3): 251–254. doi:10.1130/g24584a.1. Bibcode: 2008Geo....36..251L.
- ↑ Diester-Haass, L.; Zahn, R. (1996). "Eocene-Oligocene transition in the Southern Ocean: History of water mass circulation and biological productivity". Geology 24 (2): 163–166. doi:10.1130/0091-7613(1996)024<0163:eotits>2.3.co;2. Bibcode: 1996Geo....24..163D.
- ↑ 105.0 105.1 Barker, P. F.; Thomas, E. (2004). "Origin, signature and palaeoclimatic influence of the Antarctic Circumpolar Current". Earth-Science Reviews 66 (1–2): 143–162. doi:10.1016/j.earscirev.2003.10.003. Bibcode: 2004ESRv...66..143B.
- ↑ Huber, M.; Nof, D. (2006). "The ocean circulation in the southern hemisphere and its climatic impacts in the Eocene". Palaeogeography, Palaeoclimatology, Palaeoecology 231 (1–2): 9–28. doi:10.1016/j.palaeo.2005.07.037.
- ↑ Barker, P. F.; Filippelli, Gabriel M.; Florindo, Fabio; Martin, Ellen E.; Scher, Howard D. (2007). "Onset and Role of the Antarctic Circumpolar Current". Topical Studies in Oceanography 54 (21–22): 2388–2398. doi:10.1016/j.dsr2.2007.07.028. Bibcode: 2007DSRII..54.2388B. http://www.earth-prints.org/bitstream/2122/2184/1/1214.pdf.
- ↑ Shi, Juye; Jin, Zhijun; Liu, Quanyou; Fan, Tailiang; Gao, Zhiqian (1 October 2021). "Sunspot cycles recorded in Eocene lacustrine fine-grained sedimentary rocks in the Bohai Bay Basin, eastern China". Global and Planetary Change 205: 103614. doi:10.1016/j.gloplacha.2021.103614. ISSN 0921-8181. https://www.sciencedirect.com/science/article/pii/S0921818121001995. Retrieved 26 December 2023.
- ↑ Briggs, John (1995). Global Biogeography. Elsevier. p116 Fig 40. ISBN 0-444-88297-9.
- ↑ Wing, Scott L.; Greenwood, David R. (28 August 1993). "Fossils and fossil climate: the case for equable continental interiors in the Eocene". Philosophical Transactions of the Royal Society of London. Series B: Biological Sciences 341 (1297): 243–252. doi:10.1098/rstb.1993.0109.
- ↑ Jahren, A. Hope (28 August 1993). "Fossils and fossil climate: the case for equable continental interiors in the Eocene". Philosophical Transactions of the Royal Society of London. Series B: Biological Sciences 341 (1297): 243–252. doi:10.1098/rstb.1993.0109.
- ↑ Gandolfo, MA; Hermsen, EJ; Zamaloa, MC; Nixon, KC; González, CC (2011). "Oldest Known Eucalyptus Macrofossils Are from South America". PLOS ONE 6 (6): e21084. doi:10.1371/journal.pone.0021084. PMID 21738605. Bibcode: 2011PLoSO...621084G.
- ↑ Briggs 1995, p. 118.
- ↑ Bender, Michael (2013). Paleoclimate. Princeton University Press. pp. 108.
- ↑ "Mammals' bodies outpaced their brains right after the dinosaurs died". Science News. 31 March 2022. https://www.sciencenews.org/article/mammal-big-bodies-small-brains-dinosaur-extinction-fossils.
- ↑ Bertrand, Ornella C.; Shelley, Sarah L.; Williamson, Thomas E.; Wible, John R.; Chester, Stephen G. B.; Flynn, John J.; Holbrook, Luke T.; Lyson, Tyler R. et al. (April 2022). "Brawn before brains in placental mammals after the end-Cretaceous extinction" (in en). Science 376 (6588): 80–85. doi:10.1126/science.abl5584. ISSN 0036-8075. PMID 35357913. Bibcode: 2022Sci...376...80B. https://www.science.org/doi/10.1126/science.abl5584. Retrieved 19 November 2023.
- ↑ Georgalis, Georgios L.; Abdel Gawad, Mohamed K.; Hassan, Safiya M.; El-Barkooky, Ahmed N.; Hamdan, Mohamed A. (2020-05-22). "Oldest co-occurrence of Varanus and Python from Africa—first record of squamates from the early Miocene of Moghra Formation, Western Desert, Egypt". PeerJ 8: e9092. doi:10.7717/peerj.9092. ISSN 2167-8359. PMID 32509449.
- ↑ Wolfe, Alexander P.; Tappert, Ralf; Muehlenbachs, Karlis; Boudreau, Marc; McKellar, Ryan C.; Basinger, James F.; Garrett, Amber (1 July 2009). "A new proposal concerning the botanical origin of Baltic amber". Proceedings of the Royal Society B 276 (1972): 3403–3412. doi:10.1098/rspb.2009.0806. PMID 19570786.
Further reading
- Ogg, Jim; June, 2004, Overview of Global Boundary Stratotype Sections and Points (GSSP's) Global Stratotype Sections and Points Accessed April 30, 2006.
- Stanley, Steven M. Earth System History. New York City : W.H. Freeman and Company, 1999. ISBN:0-7167-2882-6
External links
- PaleoMap Project
- Paleos Eocene page
- PBS Deep Time: Eocene
- Eocene and Oligocene Fossils
- The UPenn Fossil Forest Project, focusing on the Eocene polar forests in Ellesmere Island, Canada
- Basilosaurus Primitive Eocene Whales
- Basilosaurus - The plesiosaur that wasn't....
- Detailed maps of Tertiary Western North America
- Map of Eocene Earth
- Eocene Microfossils: 60+ images of Foraminifera
- Eocene Epoch. (2011). In Encyclopædia Britannica. Retrieved from Eocene Epoch | geochronology
![]() | Original source: https://en.wikipedia.org/wiki/Eocene.
Read more |