Biology:Marine invertebrates
Marine invertebrates are the invertebrates that live in marine habitats. Invertebrate is a blanket term that includes all animals apart from the vertebrate members of the chordate phylum. Invertebrates lack a vertebral column, and some have evolved a shell or a hard exoskeleton. As on land and in the air, marine invertebrates have a large variety of body plans, and have been categorised into over 30 phyla. They make up most of the macroscopic life in the oceans.
Evolution
Part of a series of overviews on |
Marine life |
---|
![]() |
The earliest animals were marine invertebrates, that is, vertebrates came later. Animals are multicellular eukaryotes,[note 1] and are distinguished from plants, algae, and fungi by lacking cell walls.[1] Marine invertebrates are animals that inhabit a marine environment apart from the vertebrate members of the chordate phylum; invertebrates lack a vertebral column. Some have evolved a shell or a hard exoskeleton.
The earliest animals may belong to the genus Dickinsonia,[2] 571 million to 539 million years ago.[3] Individual Dickinsonia typically resemble a bilaterally symmetrical ribbed oval. They kept growing until they were covered with sediment or otherwise killed,[4] and spent most of their lives with their bodies firmly anchored to the sediment.[5] Their taxonomic affinities are presently unknown, but their mode of growth is consistent with a bilaterian affinity.[6]
Apart from Dickinsonia, the earliest widely accepted animal fossils are the rather modern-looking cnidarians (the group that includes jellyfish, sea anemones and Hydra), possibly from around 580 Ma[7] The Ediacara biota, which flourished for the last 40 million years before the start of the Cambrian,[8] were the first animals more than a very few centimetres long. Like Dickinsonia, many were flat with a "quilted" appearance, and seemed so strange that there was a proposal to classify them as a separate kingdom, Vendozoa.[9] Others, however, have been interpreted as early molluscs (Kimberella[10][11]), echinoderms (Arkarua[12]), and arthropods (Spriggina,[13] Parvancorina[14]). There is still debate about the classification of these specimens, mainly because the diagnostic features which allow taxonomists to classify more recent organisms, such as similarities to living organisms, are generally absent in the Ediacarans. However, there seems little doubt that Kimberella was at least a triploblastic bilaterian animal, in other words, an animal significantly more complex than the cnidarians.[15]
The small shelly fauna are a very mixed collection of fossils found between the Late Ediacaran and Middle Cambrian periods. The earliest, Cloudina, shows signs of successful defense against predation and may indicate the start of an evolutionary arms race. Some tiny Early Cambrian shells almost certainly belonged to molluscs, while the owners of some "armor plates," Halkieria and Microdictyon, were eventually identified when more complete specimens were found in Cambrian lagerstätten that preserved soft-bodied animals.[16]
Dickinsonia may be the earliest animal. They appear in the fossil record 571 million to 541 million years ago.
Kimberella, an early mollusc important for understanding the Cambrian explosion. Invertebrates are grouped into different phyla (body plans).
Opabinia, an extinct stem group arthropod appeared in the Middle Cambrian[17]:124–136
In the 1970s there was already a debate about whether the emergence of the modern phyla was "explosive" or gradual but hidden by the shortage of Precambrian animal fossils.[16] A re-analysis of fossils from the Burgess Shale lagerstätte increased interest in the issue when it revealed animals, such as Opabinia, which did not fit into any known phylum. At the time these were interpreted as evidence that the modern phyla had evolved very rapidly in the Cambrian explosion and that the Burgess Shale's "weird wonders" showed that the Early Cambrian was a uniquely experimental period of animal evolution.[17] Later discoveries of similar animals and the development of new theoretical approaches led to the conclusion that many of the "weird wonders" were evolutionary "aunts" or "cousins" of modern groups[18]—for example that Opabinia was a member of the lobopods, a group which includes the ancestors of the arthropods, and that it may have been closely related to the modern tardigrades.[19] Nevertheless, there is still much debate about whether the Cambrian explosion was really explosive and, if so, how and why it happened and why it appears unique in the history of animals.[20]
Classification
Invertebrates are grouped into different phyla. Informally phyla can be thought of as a way of grouping organisms according to their body plan.[21][22]:33 A body plan refers to a blueprint which describes the shape or morphology of an organism, such as its symmetry, segmentation and the disposition of its appendages. The idea of body plans originated with vertebrates, which were grouped into one phylum. But the vertebrate body plan is only one of many, and invertebrates consist of many phyla or body plans. The history of the discovery of body plans can be seen as a movement from a worldview centred on vertebrates, to seeing the vertebrates as one body plan among many. Among the pioneering zoologists, Linnaeus identified two body plans outside the vertebrates; Cuvier identified three; and Haeckel had four, as well as the Protista with eight more, for a total of twelve. For comparison, the number of phyla recognised by modern zoologists has risen to 35.[22]
Historically body plans were thought of as having evolved in rapidly during the Cambrian explosion,[23] but a more nuanced understanding of animal evolution suggests a gradual development of body plans throughout the early Palaeozoic and beyond.[24] More generally a phylum can be defined in two ways: as described above, as a group of organisms with a certain degree of morphological or developmental similarity (the phenetic definition), or a group of organisms with a certain degree of evolutionary relatedness (the phylogenetic definition).[24]
As on land and in the air, invertebrates make up a great majority of all macroscopic life, as the vertebrates makes up a subphylum of one of over 30 known animal phyla, making the term almost meaningless for taxonomic purpose. Invertebrate sea life includes the following groups, some of which are phyla:
- Acoela, among the most primitive bilateral animals;
- Annelida, (polychaetes and sea leeches);
- Brachiopoda, marine animals that have hard "valves" (shells) on the upper and lower surfaces ;
- Bryozoa, also known as moss animals or sea mats;
- Chaetognatha, commonly known as arrow worms, are a phylum of predatory marine worms that are a major component of plankton;
- Cephalochordata represented in the modern oceans by the lancelets (also known as Amphioxus);
- Cnidaria, such as jellyfish, sea anemones, and corals;
- Crustacea, including lobsters, crabs, shrimp, crayfish, barnacles, hermit crabs, mantis shrimps, and copepods;
- Ctenophora, also known as comb jellies, the largest animals that swim by means of cilia;
- Echinodermata, including sea stars, brittle stars, sea urchins, sand dollars, sea cucumbers, crinoids, and sea daisies;
- Echiura, also known as spoon worms;
- Gnathostomulids, slender to thread-like worms, with a transparent body that inhabit sand and mud beneath shallow coastal waters;
- Gastrotricha, often called hairy backs, found mostly interstitially in between sediment particles;
- Hemichordata, includes acorn worms, solitary worm-shaped organisms;
- Kamptozoa, goblet-shaped sessile aquatic animals, with relatively long stalks and a "crown" of solid tentacles, also called Entoprocta;
- Kinorhyncha, segmented, limbless animals, widespread in mud or sand at all depths, also called mud dragons;
- Loricifera, very small to microscopic marine sediment-dwelling animals only discovered in 1983;
- Mollusca, including shellfish, squid, octopus, whelks, Nautilus, cuttlefish, nudibranchs, scallops, sea snails, Aplacophora, Caudofoveata, Monoplacophora, Polyplacophora, and Scaphopoda;
- Myzostomida, a taxonomic group of small marine worms which are parasitic on crinoids or "sea lilies";
- Nemertinea, also known as "ribbon worms" or "proboscis worms";
- Orthonectida, a small phylum of poorly known parasites of marine invertebrates that are among the simplest of multi-cellular organisms;
- Phoronida, a phylum of marine animals that filter-feed with a lophophore (a "crown" of tentacles), and build upright tubes of chitin to support and protect their soft bodies;
- Placozoa, small, flattened, multicellular animals around 1 millimetre across and the simplest in structure. They have no regular outline, although the lower surface is somewhat concave, and the upper surface is always flattened;
- Porifera (sponges), multicellular organisms that have bodies full of pores and channels allowing water to circulate through them;
- Priapulida, or penis worms, are a phylum of marine worms that live marine mud. They are named for their extensible spiny proboscis, which, in some species, may have a shape like that of a human penis;
- Pycnogonida, also called sea spiders, are unrelated to spiders, or even to arachnids which they resemble;
- Sipunculida, also called peanut worms, is a group containing 144–320 species (estimates vary) of bilaterally symmetrical, unsegmented marine worms;
- Tunicata, also known as sea squirts or sea pork, are filter feeders attached to rocks or similarly suitable surfaces on the ocean floor;
- Some flatworms of the classes Turbellaria and Monogenea;
- Xenoturbella, a genus of bilaterian animals that contains only two marine worm-like species;
- Xiphosura, includes a large number of extinct lineages and only four recent species in the family Limulidae, which include the horseshoe crabs.
Arthropods total about 1,113,000 described extant species, molluscs about 85,000 and chordates about 52,000.[25]
Marine sponges
Sponges are animals of the phylum Porifera (Modern Latin for bearing pores[26]). They are multicellular organisms that have bodies full of pores and channels allowing water to circulate through them, consisting of jelly-like mesohyl sandwiched between two thin layers of cells. They have unspecialized cells that can transform into other types and that often migrate between the main cell layers and the mesohyl in the process. Sponges do not have nervous, digestive or circulatory systems. Instead, most rely on maintaining a constant water flow through their bodies to obtain food and oxygen and to remove wastes.
Sponges are similar to other animals in that they are multicellular, heterotrophic, lack cell walls and produce sperm cells. Unlike other animals, they lack true tissues and organs, and have no body symmetry. The shapes of their bodies are adapted for maximal efficiency of water flow through the central cavity, where it deposits nutrients, and leaves through a hole called the osculum. Many sponges have internal skeletons of spongin and/or spicules of calcium carbonate or silicon dioxide. All sponges are sessile aquatic animals. Although there are freshwater species, the great majority are marine (salt water) species, ranging from tidal zones to depths exceeding 8,800 m (5.5 mi).
While most of the approximately 5,000–10,000 known species feed on bacteria and other food particles in the water, some host photosynthesizing micro-organisms as endosymbionts and these alliances often produce more food and oxygen than they consume. A few species of sponge that live in food-poor environments have become carnivores that prey mainly on small crustaceans.[27]
Venus' flower basket at a depth of 2572 meters
Linnaeus mistakenly identified sponges as plants in the order Algae.[29] For a long time thereafter sponges were assigned to a separate subkingdom, Parazoa (meaning beside the animals).[30] They are now classified as a paraphyletic phylum from which the higher animals have evolved.[31]
Marine cnidarians
Cnidarians (Greek for nettle) are distinguished by the presence of stinging cells, specialized cells that they use mainly for capturing prey. Cnidarians include corals, sea anemones, jellyfish and hydrozoans. They form a phylum containing over 10,000[32] species of animals found exclusively in aquatic (mainly marine) environments. Their bodies consist of mesoglea, a non-living jelly-like substance, sandwiched between two layers of epithelium that are mostly one cell thick. They have two basic body forms: swimming medusae and sessile polyps, both of which are radially symmetrical with mouths surrounded by tentacles that bear cnidocytes. Both forms have a single orifice and body cavity that are used for digestion and respiration.
Fossil cnidarians have been found in rocks formed about 580 million years ago. Fossils of cnidarians that do not build mineralized structures are rare. Scientists currently think cnidarians, ctenophores and bilaterians are more closely related to calcareous sponges than these are to other sponges, and that anthozoans are the evolutionary "aunts" or "sisters" of other cnidarians, and the most closely related to bilaterians.
Cnidarians are the simplest animals in which the cells are organised into tissues.[33] The starlet sea anemone is used as a model organism in research.[34] It is easy to care for in the laboratory and a protocol has been developed which can yield large numbers of embryos on a daily basis.[35] There is a remarkable degree of similarity in the gene sequence conservation and complexity between the sea anemone and vertebrates.[35] In particular, genes concerned in the formation of the head in vertebrates are also present in the anemone.[36][37]
Their tentacles sting and paralyse small fish
Close up of polyps on the surface of a coral, waving their tentacles.
If an island sinks below the sea, coral growth can keep pace with the rising water and form an atoll
Lion's mane jellyfish, largest known jellyfish[38]
The bioluminescence sea walnut has a transient anus[39] which forms only when it needs to defecate
Turritopsis dohrnii achieves biological immortality by transferring its cells back to childhood[40][41]
Marine worms
Worms (Old English for serpent) typically have long cylindrical tube-like bodies and no limbs. Marine worms vary in size from microscopic to over 1 metre (3.3 ft) in length for some marine polychaete worms (bristle worms)[42] and up to 58 metres (190 ft) for the marine nemertean worm (bootlace worm).[43] Some marine worms occupy a small variety of parasitic niches, living inside the bodies of other animals, while others live more freely in the marine environment or by burrowing underground.
Different groups of marine worms are related only distantly, so they are found in several different phyla such as the Annelida (segmented worms), Chaetognatha (arrow worms), Hemichordata, and Phoronida (horseshoe worms). Many of these worms have specialized tentacles used for exchanging oxygen and carbon dioxide and also may be used for reproduction. Some marine worms are tube worms, such as the giant tube worm which lives in waters near underwater volcanoes and can withstand temperatures up to 90 degrees Celsius.
Platyhelminthes (flatworms) form another worm phylum which includes a class Cestoda of parasitic tapeworms. The marine tapeworm Polygonoporus giganticus, found in the gut of sperm whales, can grow to over 30 m (100 ft).[44][45]
Nematodes (roundworms) constitute a further worm phylum with tubular digestive systems and an opening at both ends.[46][47] Over 25,000 nematode species have been described,[48][49] of which more than half are parasitic. It has been estimated another million remain undescribed.[50] They are ubiquitous in marine, freshwater and terrestrial environments, where they often outnumber other animals in both individual and species counts. They are found in every part of the earth's lithosphere, from the top of mountains to the bottom of oceanic trenches.[51] By count they represent 90% of all animals on the ocean floor.[52] Their numerical dominance, often exceeding a million individuals per square meter and accounting for about 80% of all individual animals on earth, their diversity of life cycles, and their presence at various trophic levels point at an important role in many ecosystems.[53]
Giant tube worms cluster around hydrothermal vents
Lamellibrachia luymes, a cold seep tubeworm, lives over 250 years.
Nematodes are ubiquitous pseudocoelomates which can parasite marine plants and animals.
Bobbit worms are ambush predators that live on the seafloor
Echinoderms
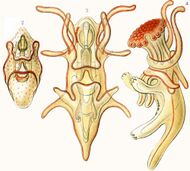
Echinoderms (Greek for spiny skin) is a phylum which contains only marine invertebrates. The adults are recognizable by their radial symmetry (usually five-point) and include starfish, sea urchins, sand dollars, and sea cucumbers, as well as the sea lilies.[citation needed] Echinoderms are found at every ocean depth, from the intertidal zone to the abyssal zone. The phylum contains about 7000 living species,[54] making it the second-largest grouping of deuterostomes (a superphylum), after the chordates (which include the vertebrates, such as birds, fishes, mammals, and reptiles).
Echinoderms are unique among animals in having bilateral symmetry at the larval stage, but fivefold symmetry (pentamerism, a special type of radial symmetry) as adults.[55]
The echinoderms are important both biologically and geologically. Biologically, there are few other groupings so abundant in the biotic desert of the deep sea, as well as shallower oceans. Most echinoderms are able to regenerate tissue, organs, limbs, and reproduce asexually; in some cases, they can undergo complete regeneration from a single limb. Geologically, the value of echinoderms is in their ossified skeletons, which are major contributors to many limestone formations, and can provide valuable clues as to the geological environment. They were the most used species in regenerative research in the 19th and 20th centuries. Further, it is held by some scientists that the radiation of echinoderms was responsible for the Mesozoic Marine Revolution.
Echinoderm literally means "spiny skin", as this water melon sea urchin illustrates
Sea cucumbers filter feed on plankton and suspended solids
Benthopelagic sea cucumbers can lift off the seafloor and journey as much as 1,000 m (3,300 ft) up the water column
Colorful sea lilies in shallow waters
Aside from the hard-to-classify Arkarua (a Precambrian animal with echinoderm-like pentamerous radial symmetry), the first definitive members of the phylum appeared near the start of the Cambrian.
Marine molluscs
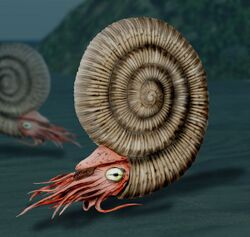
Molluscs (Latin for soft) form a phylum with about 85,000 extant recognized species.[57] By species count they are the largest marine phylum, comprising about 23% of all the named marine organisms.[58] Molluscs have more varied forms than other invertebrate phyla. They are highly diverse, not just in size and in anatomical structure, but also in behaviour and in habitat. The majority of species still live in the oceans, from the seashores to the abyssal zone, but some form a significant part of the freshwater fauna and the terrestrial ecosystems.
The mollusc phylum is divided into 9 or 10 taxonomic classes, two of which are extinct. These classes include gastropods, bivalves and cephalopods, as well as other lesser-known but distinctive classes. Gastropods with protective shells are referred to as snails (sea snails), whereas gastropods without protective shells are referred to as slugs (sea slugs). Gastropods are by far the most numerous molluscs in terms of classified species, accounting for 80% of the total.[25] Bivalves include clams, oysters, cockles, mussels, scallops, and numerous other families. There are about 8,000 marine bivalves species (including brackish water and estuarine species), and about 1,200 freshwater species. Cephalopod include octopus, squid and cuttlefish. They are found in all oceans, and neurologically are the most advanced of the invertebrates.[59] About 800 living species of marine cephalopods have been identified,[60] and an estimated 11,000 extinct taxa have been described.[61] There are no fully freshwater cephalopods.[62]
Colossal squid, largest of all invertebrates[63]
The nautilus is a living fossil little changed since it evolved 500 million years ago as one of the first cephalopods.[64][65][66]
Marine gastropods are sea snails or sea slugs. This nudibranch is a sea slug.
The sea snail Syrinx aruanus has the largest shell of any living gastropod
Molluscs usually have eyes. Bordering the edge of the mantle of a scallop, a bivalve mollusc, can be over 100 simple eyes.
Molluscs have such diverse shapes that many textbooks base their descriptions of molluscan anatomy on a generalized or hypothetical ancestral mollusc. This generalized mollusc is unsegmented and bilaterally symmetrical with an underside consisting of a single muscular foot.[67][68]:484–628 Beyond that it has three further key features. Firstly, it has a muscular cloak called a mantle covering its viscera and containing a significant cavity used for breathing and excretion. A shell secreted by the mantle covers the upper surface.[68] Secondly (apart from bivalves) it has a rasping tongue called a radula used for feeding. Thirdly, it has a nervous system including a complex digestive system using microscopic, muscle-powered hairs called cilia to exude mucus. The generalized mollusc has two paired nerve cords (three in bivalves). The brain, in species that have one, encircles the esophagus. Most molluscs have eyes and all have sensors detecting chemicals, vibrations, and touch. The simplest type of molluscan reproductive system relies on external fertilization, but more complex variations occur. All produce eggs, from which may emerge trochophore larvae, more complex veliger larvae, or miniature adults. The depiction is rather similar to modern monoplacophorans, and some suggest it may resemble very early molluscs.[67]:284–291[67]:298–300[69][70]
Good evidence exists for the appearance of marine gastropods, cephalopods and bivalves in the Cambrian period 541 to 485.4 million years ago. However, the evolutionary history both of molluscs' emergence from the ancestral Lophotrochozoa and of their diversification into the well-known living and fossil forms are still subjects of vigorous debate among scientists.
Marine arthropods
Arthropods (Greek for jointed feet) have an exoskeleton (external skeleton), a segmented body, and jointed appendages (paired appendages). They form a phylum which includes insects, arachnids, myriapods, and crustaceans. Arthropods are characterized by their jointed limbs and cuticle made of chitin, often mineralised with calcium carbonate. The arthropod body plan consists of segments, each with a pair of appendages. The rigid cuticle inhibits growth, so arthropods replace it periodically by moulting. Their versatility has enabled them to become the most species-rich members of all ecological guilds in most environments.
Marine arthropods range in size from the microscopic crustacean Stygotantulus to the Japanese spider crab. Arthropods' primary internal cavity is a hemocoel, which accommodates their internal organs, and through which their haemolymph - analogue of blood - circulates; they have open circulatory systems. Like their exteriors, the internal organs of arthropods are generally built of repeated segments. Their nervous system is "ladder-like", with paired ventral nerve cords running through all segments and forming paired ganglia in each segment. Their heads are formed by fusion of varying numbers of segments, and their brains are formed by fusion of the ganglia of these segments and encircle the esophagus. The respiratory and excretory systems of arthropods vary, depending as much on their environment as on the subphylum to which they belong.
Their vision relies on various combinations of compound eyes and pigment-pit ocelli: in most species the ocelli can only detect the direction from which light is coming, and the compound eyes are the main source of information, but the main eyes of spiders are ocelli that can form images and, in a few cases, can swivel to track prey. Arthropods also have a wide range of chemical and mechanical sensors, mostly based on modifications of the many setae (bristles) that project through their cuticles. Arthropods' methods of reproduction and development are diverse; all terrestrial species use internal fertilization, but this is often by indirect transfer of the sperm via an appendage or the ground, rather than by direct injection. Marine species all lay eggs and use either internal or external fertilization. Arthropod hatchlings vary from miniature adults to grubs that lack jointed limbs and eventually undergo a total metamorphosis to produce the adult form.
Trilobites, now extinct, roamed oceans for 270 million years.[71]
Horseshoe crab, a living fossil arthropod from 450 million years ago
- Crustaceans
Many crustaceans are very small, like this tiny amphipod, and make up a significant part of the ocean's zooplankton
The banded cleaner shrimp is a crustacean common in the tropics.
The Tasmanian giant crab is long-lived and slow-growing, making it vulnerable to overfishing.[72]
The Japanese spider crab has the longest leg span of any arthropod.
The evolutionary ancestry of arthropods dates back to the Cambrian period. The group is generally regarded as monophyletic, and many analyses support the placement of arthropods with cycloneuralians (or their constituent clades) in a superphylum Ecdysozoa. Overall however, the basal relationships of Metazoa are not yet well resolved. Likewise, the relationships between various arthropod groups are still actively debated.
Other phyla
- Tardigrade, Lobopodia, (Onychophora)
- Non-craniate (non-vertebrate) chordates: Cephalochordate, Tunicata and Haikouella. These invertebrates are close relatives of the vertebrates.
- Non-craniate chordates are close relatives of vertebrates
The lancelet, a small translucent fish-like Cephalochordate, is the closest living invertebrate relative of the vertebrates.[73][74]
Fluorescent-colored sea squirts, Rhopalaea crassa. Tunicates may provide clues to vertebrate (and therefore human) ancestry.[75]
Salp chain
Minerals from sea water
There are a number of marine invertebrates that use minerals that are present in the sea in such minute quantities that they were undetectable until the advent of spectroscopy. Vanadium is concentrated by some tunicates for use in their blood cells to a level ten million times that of the surrounding seawater. Other tunicates similarly concentrate niobium and tantalum.[67]:947 Lobsters use copper in their respiratory pigment hemocyanin, despite the proportion of this metal in seawater being minute.[67]:638 Although these elements are present in vast quantities in the ocean, their extraction by man is not economic.[76]
Glass models
- Blaschka glass marine invertebrates, 1863–1880
See also
Notes
- ↑ Myxozoa were thought to be an exception, but are now thought to be heavily modified members of the Cnidaria. Jímenez-Guri, Eva; Philippe, Hervé; Okamura, Beth; Holland, Peter W. H. (July 6, 2007). "Buddenbrockia Is a Cnidarian Worm". Science 317 (5834): 116–118. doi:10.1126/science.1142024. ISSN 0036-8075. PMID 17615357. Bibcode: 2007Sci...317..116J.
References
- ↑ Davidson, Michael W. (May 26, 2005). "Animal Cell Structure". Tallahassee, FL: Florida State University. http://micro.magnet.fsu.edu/cells/animalcell.html.
- ↑ Vogel, Gretchen (2018-09-20). "This fossil is one of the world's earliest animals, according to fat molecules preserved for a half-billion years". AAAS. https://www.science.org/content/article/fossil-one-world-s-earliest-animals-according-fat-molecules-preserved-half-billion.
- ↑ Bobrovskiy, Ilya (2018). "Ancient steroids establish the Ediacaran fossil Dickinsonia as one of the earliest animals". Science 361 (6408): 1246–1249. doi:10.1126/science.aat7228. PMID 30237355. Bibcode: 2018Sci...361.1246B.
- ↑ Retallack, G.J. (2007). "Growth, decay and burial compaction of Dickinsonia, an iconic Ediacaran fossil". Alcheringa: An Australasian Journal of Palaeontology 31 (3): 215–240. doi:10.1080/03115510701484705. http://www.informaworld.com/index/781217204.pdf.
- ↑ Template:PalAss2008
- ↑ Gold, D. A.; Runnegar, B.; Gehling, J. G.; Jacobs, D. K. (2015). "Ancestral state reconstruction of ontogeny supports a bilaterian affinity for Dickinsonia". Evolution & Development 17 (6): 315–397. doi:10.1111/ede.12168. PMID 26492825.
- ↑ Jun-Yuan Chen; Oliveri, Paola; Feng Gao et al. (August 1, 2002). "Precambrian Animal Life: Probable Developmental and Adult Cnidarian Forms from Southwest China". Developmental Biology 248 (1): 182–196. doi:10.1006/dbio.2002.0714. ISSN 0012-1606. PMID 12142030. https://pantherfile.uwm.edu/sdornbos/www/PDF%27s/Chen%20et%20al.%202002.pdf. Retrieved 2015-02-04.
- ↑ Grazhdankin, Dima (June 2004). "Patterns of distribution in the Ediacaran biotas: facies versus biogeography and evolution". Paleobiology 30 (2): 203–221. doi:10.1666/0094-8373(2004)030<0203:PODITE>2.0.CO;2. ISSN 0094-8373.
- ↑ Seilacher, Adolf (August 1992). "Vendobionta and Psammocorallia: lost constructions of Precambrian evolution". Journal of the Geological Society 149 (4): 607–613. doi:10.1144/gsjgs.149.4.0607. ISSN 0016-7649. Bibcode: 1992JGSoc.149..607S. http://jgs.lyellcollection.org/content/149/4/607.abstract. Retrieved 2015-02-04.
- ↑ Martin, Mark W.; Grazhdankin, Dmitriy V.; Bowring, Samuel A. et al. (May 5, 2000). "Age of Neoproterozoic Bilaterian Body and Trace Fossils, White Sea, Russia: Implications for Metazoan Evolution". Science 288 (5467): 841–845. doi:10.1126/science.288.5467.841. ISSN 0036-8075. PMID 10797002. Bibcode: 2000Sci...288..841M.
- ↑ Fedonkin, Mikhail A.; Waggoner, Benjamin M. (August 28, 1997). "The late Precambrian fossil Kimberella is a mollusc-like bilaterian organism". Nature 388 (6645): 868–871. doi:10.1038/42242. ISSN 0028-0836. Bibcode: 1997Natur.388..868F.
- ↑ Mooi, Rich; David, Bruno (December 1998). "Evolution Within a Bizarre Phylum: Homologies of the First Echinoderms". American Zoologist 38 (6): 965–974. doi:10.1093/icb/38.6.965. ISSN 1540-7063. http://icb.oxfordjournals.org/content/38/6/965.full.pdf. Retrieved 2015-02-05.
- ↑ McMenamin, Mark A. S. (September 2003). "Spriggina is a trilobitoid ecdysozoan". Geoscience Horizons Seattle 2003. 35. Boulder, CO: Geological Society of America. p. 105. OCLC 249088612. https://gsa.confex.com/gsa/2003AM/finalprogram/abstract_62056.htm. Retrieved 2007-11-24. Paper No. 40-2 presented at the Geological Society of America's 2003 Seattle Annual Meeting (November 2–5, 2003) on November 2, 2003, at the Washington State Convention Center.
- ↑ Jih-Pai Lin; Gon, Samuel M. III; Gehling, James G. et al. (2006). "A Parvancorina-like arthropod from the Cambrian of South China". Historical Biology: An International Journal of Paleobiology 18 (1): 33–45. doi:10.1080/08912960500508689. ISSN 1029-2381.
- ↑ Butterfield, Nicholas J. (December 2006). "Hooking some stem-group 'worms': fossil lophotrochozoans in the Burgess Shale". BioEssays 28 (12): 1161–1166. doi:10.1002/bies.20507. ISSN 0265-9247. PMID 17120226.
- ↑ 16.0 16.1 Bengtson, Stefan (2004). "Early Skeletal Fossils". in Lipps, Jere H.; Waggoner, Benjamin M.. Neoproterozoic-Cambrian Biological Revolutions: Presented as a Paleontological Society Short Course at the Annual Meeting of the Geological Society of America, Denver, Colorado, November 6, 2004. Paleontological Society Papers. 10. New Haven, CT: Yale University Reprographics & Imaging Service; Paleontological Society. pp. 67–78. OCLC 57481790.
- ↑ 17.0 17.1 Gould, Stephen Jay (1990) Wonderful Life: The Burgess Shale and the Nature of History W. W. Norton. ISBN:9780393307009.
- ↑ Budd, Graham E. (February 2003). "The Cambrian Fossil Record and the Origin of the Phyla" (PDF). Integrative and Comparative Biology 43 (1): 157–165. doi:10.1093/icb/43.1.157. ISSN 1557-7023. PMID 21680420. http://icb.oxfordjournals.org/content/43/1/157.full.pdf+html. Retrieved 2015-02-06.
- ↑ Budd, Graham E. (March 1996). "The morphology of Opabinia regalis and the reconstruction of the arthropod stem-group". Lethaia 29 (1): 1–14. doi:10.1111/j.1502-3931.1996.tb01831.x. ISSN 0024-1164.
- ↑ Marshall, Charles R. (May 2006). "Explaining the Cambrian 'Explosion' of Animals". Annual Review of Earth and Planetary Sciences 34: 355–384. doi:10.1146/annurev.earth.33.031504.103001. ISSN 1545-4495. Bibcode: 2006AREPS..34..355M.
- ↑ Valentine, James W. (2004). On the Origin of Phyla. Chicago: University Of Chicago Press. p. 7. ISBN 978-0-226-84548-7. "Classifications of organisms in hierarchical systems were in use by the seventeenth and eighteenth centuries. Usually organisms were grouped according to their morphological similarities as perceived by those early workers, and those groups were then grouped according to their similarities, and so on, to form a hierarchy."
- ↑ 22.0 22.1 Valentine, James W (2004-06-18). On the Origin of Phyla. University of Chicago Press. ISBN 978-0-226-84548-7. https://books.google.com/books?id=DMBkmHm5fe4C.
- ↑ Erwin, Douglas; Valentine, James; Jablonski, David (1997). "Recent fossil finds and new insights into animal development are providing fresh perspectives on the riddle of the explosion of animals during the Early Cambrian". American Scientist (March–April). http://www.americanscientist.org/issues/num2/the-origin-of-animal-body-plans/3.
- ↑ 24.0 24.1 Budd, G.E.; Jensen, S. (May 2000). "A critical reappraisal of the fossil record of the bilaterian phyla". Biological Reviews 75 (2): 253–295. doi:10.1111/j.1469-185X.1999.tb00046.x. PMID 10881389. http://www.journals.cambridge.org/abstract_S000632310000548X.
- ↑ 25.0 25.1 Ponder, W.F., ed (2008). Phylogeny and Evolution of the Mollusca. Berkeley: University of California Press. p. 481. ISBN 978-0-520-25092-5.
- ↑ Porifera (n.) Online Etymology Dictionary. Retrieved 18 August 2016.
- ↑ "Prey capture and digestion in the carnivorous sponge Asbestopluma hypogea (Porifera: Demospongiae)". Zoomorphology 123 (4): 179–190. 2004. doi:10.1007/s00435-004-0100-0.
- ↑ C. K. 'Bob' Brain; Anthony R. Prave; Karl-Heinz Hoffmann; Anthony E. Fallick; Andre Botha; Donald A. Herd; Craig Sturrock; Iain Young et al. (2012). "The first animals: ca. 760-million-year-old sponge-like fossils from Namibia". South African Journal of Science 108 (1/2). doi:10.4102/sajs.v108i1/2.658. http://www.sajs.co.za/index.php/SAJS/article/view/658/966.
- ↑ "Spongia Linnaeus, 1759". World Register of Marine Species. http://www.marinespecies.org/aphia.php?p=sourceget&id=44036.
- ↑ Rowland, S. M.; Stephens, T. (2001). "Archaeocyatha: A history of phylogenetic interpretation". Journal of Paleontology 75 (6): 1065–1078. doi:10.1666/0022-3360(2001)075<1065:AAHOPI>2.0.CO;2.
- ↑ Sperling, E. A.; Pisani, D.; Peterson, K. J. (January 1, 2007). "Poriferan paraphyly and its implications for Precambrian palaeobiology". Geological Society, London, Special Publications 286 (1): 355–368. doi:10.1144/SP286.25. Bibcode: 2007GSLSP.286..355S. http://www.dartmouth.edu/~peterson/Sperling,%20Pisani%20and%20Peterson.pdf. Retrieved August 22, 2012.
- ↑ Zhang, Z.-Q. (2011). "Animal biodiversity: An introduction to higher-level classification and taxonomic richness". Zootaxa 3148: 7–12. doi:10.11646/zootaxa.3148.1.3. http://mapress.com/zootaxa/2011/f/zt03148p012.pdf.
- ↑ "Nematostella vectensis v1.0". Genome Portal. University of California. http://genome.jgi-psf.org/Nemve1/Nemve1.home.html.
- ↑ "Nematostella". Nematostella.org. http://www.nematostella.org/.
- ↑ 35.0 35.1 Genikhovich, G.; U. Technau (2009). "The Starlet sea anemone Nematostella vectensis: An anthozoan model organism for studies in comparative genomics and functional evolutionary developmental biology". Cold Spring Harbor Protocols 2009 (9): pdb.emo129. doi:10.1101/pdb.emo129. PMID 20147257.
- ↑ "Where Does Our Head Come From? Brainless Sea Anemone Sheds New Light on the Evolutionary Origin of the Head". Science Daily. 2013-02-12. https://www.sciencedaily.com/releases/2013/02/130220084436.htm#.USs4Y9Dvwpg.twitter.
- ↑ Sinigaglia, C. (2013). "The bilaterian head patterning gene six3/6 controls aboral domain development in a cnidarian". PLOS Biology 11 (2): e1001488. doi:10.1371/journal.pbio.1001488. PMID 23483856.
- ↑ Karleskint G, Richard Turner R and, James Small J (2012) Introduction to Marine Biology Cengage Learning, edition 4, page 445. ISBN:9781133364467.
- ↑ Michael Le Page (March 2019). "Animal with an anus that comes and goes could reveal how ours evolved". New Scientist. https://www.newscientist.com/article/2195656-animal-with-an-anus-that-comes-and-goes-could-reveal-how-ours-evolved/.
- ↑ Bavestrello, Giorgio; Christian Sommer; Michele Sarà (1992). "Bi-directional conversion in Turritopsis nutricula (Hydrozoa)". Scientia Marina 56 (2–3): 137–140.
- ↑ Piraino, Stefano; F. Boero; B. Aeschbach; V. Schmid (1996). "Reversing the life cycle: medusae transforming into polyps and cell transdifferentiation in Turritopsis nutricula (Cnidaria, Hydrozoa)". Biological Bulletin 190 (3): 302–312. doi:10.2307/1543022. PMID 29227703.
- ↑ "Cornwall – Nature – Superstar Worm". BBC. https://www.bbc.co.uk/cornwall/content/articles/2009/04/07/nature_worm_feature.shtml.
- ↑ Mark Carwardine (1995) The Guinness Book of Animal Records. Guinness Publishing. p. 232.
- ↑ "The Persistent Parasites". Time Magazine. 1957-04-08. http://www.time.com/time/magazine/article/0,9171,809356-1,00.html.
- ↑ Hargis, William J. (1985). "Parasitology and pathology of marine organisms of the world ocean". NOAA Tech. Rep..
- ↑ "Classification of Animal Parasites". http://plpnemweb.ucdavis.edu/nemaplex/General/animpara.htm.
- ↑ Garcia, Lynne. "Classification of Human Parasites, Vectors, and Similar Organisms". UCLA Medical Center Los Angeles California. https://cid.oxfordjournals.org/content/29/4/734.full.pdf.
- ↑ Hodda, M (2011). "Phylum Nematoda Cobb, 1932. In: Zhang, Z.-Q. (Ed.) Animal biodiversity: An outline of higher-level classification and survey of taxonomic richness". Zootaxa 3148: 63–95. doi:10.11646/zootaxa.3148.1.11.
- ↑ Zhang, Z (2013). "Animal biodiversity: An update of classification and diversity in 2013. In: Zhang, Z.-Q. (Ed.) Animal Biodiversity: An Outline of Higher-level Classification and Survey of Taxonomic Richness (Addenda 2013)". Zootaxa 3703 (1): 5–11. doi:10.11646/zootaxa.3703.1.3.
- ↑ Lambshead PJD (1993). "Recent developments in marine benthic biodiversity research". Oceanis 19 (6): 5–24.
- ↑ "Nematoda from the terrestrial deep subsurface of South Africa". Nature 474 (7349): 79–82. June 2011. doi:10.1038/nature09974. PMID 21637257. Bibcode: 2011Natur.474...79B. https://biblio.ugent.be/publication/1269676.
- ↑ "Exponential decline of deep-sea ecosystem functioning linked to benthic biodiversity loss". Curr. Biol. 18 (1): 1–8. January 2008. doi:10.1016/j.cub.2007.11.056. PMID 18164201.
- "Deep-sea species' loss could lead to oceans' collapse, study suggests". EurekAlert! (Press release). 27 December 2007.
- ↑ Platt HM (1994). "foreword". The phylogenetic systematics of freeliving nematodes. London: The Ray Society. ISBN 978-0-903874-22-9.
- ↑ "Animal Diversity Web - Echinodermata". University of Michigan Museum of Zoology. http://animaldiversity.ummz.umich.edu/site/accounts/information/Echinodermata.html.
- ↑ Fox, Richard. "Asterias forbesi". Invertebrate Anatomy OnLine. Lander University. http://lanwebs.lander.edu/faculty/rsfox/invertebrates/asterias.html.
- ↑ Holsinger, K. (2005). Keystone species. Retrieved May 10, 2010, from "Keystone species". http://darwin.eeb.uconn.edu/eeb310/lecture-notes/interactions/node2.html.
- ↑ Chapman, A.D. (2009). Numbers of Living Species in Australia and the World, 2nd edition. Australian Biological Resources Study, Canberra. Retrieved 12 January 2010. ISBN:978-0-642-56860-1 (printed); ISBN:978-0-642-56861-8 (online).
- ↑ Hancock, Rebecca (2008). "Recognising research on molluscs". Australian Museum. http://www.austmus.gov.au/display.cfm?id=2897.
- ↑ The Invertebrates, A Synthesis (3 ed.). UK: Blackwell Science. 2001.
- ↑ "Welcome to CephBase". CephBase. http://cephbase.eol.org/.
- ↑ Wilbur, Karl M.; Clarke, M.R.; Trueman, E.R., eds. (1985), The Mollusca, 12. Paleontology and neontology of Cephalopods, New York: Academic Press, ISBN 0-12-728702-7
- ↑ "Are there any freshwater cephalopods?". 16 January 2013. http://www.abc.net.au/science/articles/2013/01/16/3670198.htm.
- ↑ Black, Richard (April 26, 2008). "Colossal squid out of the freezer". BBC News. http://news.bbc.co.uk/1/hi/sci/tech/7367774.stm.
- ↑ Ewen Callaway (2 June 2008). "Simple-Minded Nautilus Shows Flash of Memory". New Scientist. https://www.newscientist.com/article/dn14033-simpleminded-nautilus-reveals-flash-of-memory.html.
- ↑ Kathryn Phillips (15 June 2008). "Living Fossil Memories". Journal of Experimental Biology 211 (12): iii. doi:10.1242/jeb.020370.
- ↑ Robyn Crook; Jennifer Basil (2008). "A biphasic memory curve in the chambered nautilus, Nautilus pompilius L. (Cephalopoda: Nautiloidea)". The Journal of Experimental Biology 211 (12): 1992–1998. doi:10.1242/jeb.018531. PMID 18515730.
- ↑ 67.0 67.1 67.2 67.3 67.4 67.5 Ruppert, Edward E.; Fox, Richard S.; Barnes, Robert D. (2004). Invertebrate Zoology, 7th edition. Cengage Learning. ISBN 978-81-315-0104-7.
- ↑ 68.0 68.1 Hayward, PJ (1996). Handbook of the Marine Fauna of North-West Europe. Oxford University Press. ISBN 978-0-19-854055-7.
- ↑ Giribet, G.; Okusu, A, A.; Lindgren, A.R., A. R.; Huff, S.W., S. W.; Schrödl, M, M.; Nishiguchi, M.K., M. K. (May 2006). "Evidence for a clade composed of molluscs with serially repeated structures: monoplacophorans are related to chitons". Proceedings of the National Academy of Sciences of the United States of America 103 (20): 7723–7728. doi:10.1073/pnas.0602578103. PMID 16675549. Bibcode: 2006PNAS..103.7723G.
- ↑ Healy, J.M. (2001). "The Mollusca". in Anderson, D.T.. Invertebrate Zoology (2 ed.). Oxford University Press. pp. 120–171. ISBN 978-0-19-551368-4.
- ↑ Work, Yes We. "Learn more about First Life - David Attenborough's First Life". http://firstlifeseries.com/learn-more/.
- ↑ D. R. Currie; T. M. Ward (2009). South Australian Giant Crab (Pseudocarcinus gigas) Fishery. South Australian Research and Development Institute. Fishery Assessment Report for PIRSA. http://www.sardi.sa.gov.au/__data/assets/pdf_file/0019/109522/No_345_SA_Giant_Crab_Fishery_Assessment_Report_2007_08Final_Report_published_alias.pdf. Retrieved 9 December 2013.
- ↑ Gewin, V (2005). "Functional genomics thickens the biological plot". PLOS Biology 3 (6): e219. doi:10.1371/journal.pbio.0030219. PMID 15941356.
- ↑ Lancelet (amphioxus) genome and the origin of vertebrates Ars Technica, 19 June 2008.
- ↑ Lemaire, P (2011). "Evolutionary crossroads in developmental biology: the tunicates". Development 138 (11): 2143–2152. doi:10.1242/dev.048975. PMID 21558365.
- ↑ Carson, Rachel (1997). The Sea Around Us. Oxford Paperbacks. pp. 190–191. ISBN 978-0-19-506997-6.
Other references
![]() | Original source: https://en.wikipedia.org/wiki/Marine invertebrates.
Read more |