Physics:Iron group
In chemistry and physics, the iron group refers to elements that are in some way related to iron; mostly in period (row) 4 of the periodic table. The term has different meanings in different contexts.
In chemistry, the term is largely obsolete, but it often means iron, cobalt, and nickel, also called the iron triad;[1] or, sometimes, other elements that resemble iron in some chemical aspects.
In astrophysics and nuclear physics, the term is still quite common, and it typically means those three plus chromium and manganese—five elements that are exceptionally abundant, both on Earth and elsewhere in the universe, compared to their neighbors in the periodic table. Titanium and vanadium are also produced in Type Ia supernovae.[2]
General chemistry
In chemistry, "iron group" used to refer to iron and the next two elements in the periodic table, namely cobalt and nickel. These three comprised the "iron triad".[1] They are the top elements of groups 8, 9, and 10 of the periodic table; or the top row of "group VIII" in the old (pre-1990) IUPAC system, or of "group VIIIB" in the CAS system.[3] These three metals (and the three of the platinum group, immediately below them) were set aside from the other elements because they have obvious similarities in their chemistry, but are not obviously related to any of the other groups. The iron group and its alloys exhibit ferromagnetism.
The similarities in chemistry were noted as one of Döbereiner's triads and by Adolph Strecker in 1859.[4] Indeed, Newlands' "octaves" (1865) were harshly criticized for separating iron from cobalt and nickel.[5] Mendeleev stressed that groups of "chemically analogous elements" could have similar atomic weights as well as atomic weights which increase by equal increments, both in his original 1869 paper[6] and his 1889 Faraday Lecture.[7]
Analytical chemistry
In the traditional methods of qualitative inorganic analysis, the iron group consists of those cations which
- have soluble chlorides; and
- are not precipitated as sulfides by hydrogen sulfide in acidic conditions;
- are precipitated as hydroxides at around pH 10 (or less) in the presence of ammonia.
The main cations in the iron group are iron itself (Fe2+ and Fe3+), aluminium (Al3+) and chromium (Cr3+).[8] If manganese is present in the sample, a small amount of hydrated manganese dioxide is often precipitated with the iron group hydroxides.[8] Less common cations which are precipitated with the iron group include beryllium, titanium, zirconium, vanadium, uranium, thorium and cerium.[9]
Astrophysics
The iron group in astrophysics is the group of elements from chromium to nickel, which are substantially more abundant in the universe than those that come after them – or immediately before them – in order of atomic number.[10] The study of the abundances of iron group elements relative to other elements in stars and supernovae allows the refinement of models of stellar evolution.

The explanation for this relative abundance can be found in the process of nucleosynthesis in certain stars, specifically those of about 8–11 Solar masses. At the end of their lives, once other fuels have been exhausted, such stars can enter a brief phase of "silicon burning".[11] This involves the sequential addition of helium nuclei 42He (an "alpha process") to the heavier elements present in the star, starting from 2814Si:
2814Si + 42He → 3216S 3216S + 42He → 3618Ar 3618Ar + 42He → 4020Ca 4020Ca + 42He → 4422Ti [note 1] 4422Ti + 42He → 4824Cr 4824Cr + 42He → 5226Fe 5226Fe + 42He → 5628Ni
All of these nuclear reactions are exothermic: the energy that is released partially offsets the gravitational contraction of the star. However, the series ends at 5628Ni, as the next reaction in the series
5628Ni + 42He → 6030Zn
is endothermic. With no further source of energy to support itself, the core of the star collapses on itself while the outer regions are blown off in a Type II supernova.[11]
Nickel-56 is unstable with respect to beta decay, and the final stable product of silicon burning is 5626Fe.
It is often incorrectly stated that iron-56 is exceptionally common because it is the most stable of all the nuclides.[10] This is not quite true: 6228Ni and 5826Fe have slightly higher binding energies per nucleon – that is, they are slightly more stable as nuclides – as can be seen from the table on the right.[15] However, there are no rapid nucleosynthetic routes to these nuclides.
In fact, there are several stable nuclides of elements from chromium to nickel around the top of the stability curve, accounting for their relative abundance in the universe. The nuclides which are not on the direct alpha-process pathway are formed by the s-process, the capture of slow neutrons within the star.
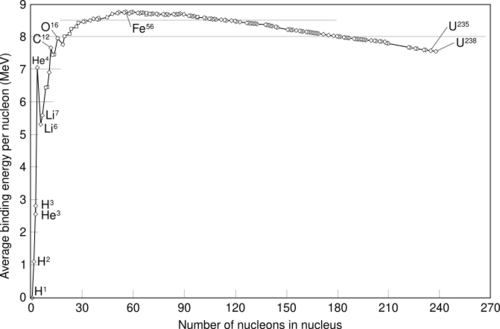
See also
- Singly ionized iron-group elements
- S-process
- Silicon burning process
- Abundance of the chemical elements
Notes and references
Notes
- ↑ In lighter stars, with less gravitational pressure, the alpha process is much slower and effectively stops at this stage as titanium-44 is unstable with respect to beta decay (t1/2 = 60.0(11) years).
References
- ↑ Jump up to: 1.0 1.1 M. Green, ed. (2002): Organometallic Chemistry, volume 10, page 283. Royal Society of Chemistry; 430 pages, ISBN 9780854043330
- ↑ Bravo, E. (2013). "Insights into thermonuclear supernovae from the incomplete Si-burning process". Astronomy & Astrophysics 550: A24. doi:10.1051/0004-6361/201220309. Bibcode: 2013A&A...550A..24B. https://www.aanda.org/articles/aa/abs/2013/02/aa20309-12/aa20309-12.html.
- ↑ Sherwood Taylor, F. (1942), Inorganic and Theoretical Chemistry (6th ed.), London: Heinemann, pp. 151–54, 727–28.
- ↑ Strecker, A. (1859), Theorien und Experimente zur Bestimmung der Atomgewichte der Elemente, Braunschweig: Friedrich Vieweg, https://books.google.com/books?id=2rc5AAAAcAAJ.
- ↑ "Proceedings of Societies [Report on the Law of Octaves]", Chemical News 13: 113, 1866, http://web.lemoyne.edu/~giunta/EA/NEWLANDSann.HTML#newlands5.
- ↑ Mendelejeff, D. (1869), "On the Relationship of the Properties of the Elements to their Atomic Weights", Z. Chem. 12: 405–6, http://web.lemoyne.edu/~giunta/EA/MENDELEEVann.HTML.
- ↑ Mendeléeff, D. (1889), "The Periodic Law of the Chemical Elements", J. Chem. Soc. 55: 634–56, doi:10.1039/ct8895500634, http://web.lemoyne.edu/~giunta/EA/MENDELann.HTML.
- ↑ Jump up to: 8.0 8.1 Template:VogelQualitative4th.
- ↑ Template:VogelQualitative4th.
- ↑ Jump up to: 10.0 10.1 Greenwood, Norman N.; Earnshaw, Alan (1984). Chemistry of the Elements. Oxford: Pergamon Press. pp. 13–16. ISBN 978-0-08-022057-4. https://books.google.com/books?id=OezvAAAAMAAJ&q=0-08-022057-6&dq=0-08-022057-6&source=bl&ots=m4tIRxdwSk&sig=XQTTjw5EN9n5z62JB3d0vaUEn0Y&hl=en&sa=X&ei=UoAWUN7-EM6ziQfyxIDoCQ&ved=0CD8Q6AEwBA..
- ↑ Jump up to: 11.0 11.1 Woosley, Stan; Janka, Thomas (2005), "The Physics of Core-Collapse Supernovae", Nature Physics 1 (3): 147–54, doi:10.1038/nphys172, Bibcode: 2005NatPh...1..147W.
- ↑ Wapstra, A.H.; Audi, G.; Thibault, C. (2003), The AME2003 Atomic Mass Evaluation (Online ed.), National Nuclear Data Center, http://www.nndc.bnl.gov/masses/. Based on:
- Wapstra, A.H.; Audi, G.; Thibault, C. (2003), "The AME2003 atomic mass evaluation (I)", Nuclear Physics A 729: 129–336, doi:10.1016/j.nuclphysa.2003.11.002, Bibcode: 2003NuPhA.729..129W
- Audi, G.; Wapstra, A.H.; Thibault, C. (2003), "The AME2003 atomic mass evaluation (II)", Nuclear Physics A 729: 337–676, doi:10.1016/j.nuclphysa.2003.11.003, Bibcode: 2003NuPhA.729..337A
- ↑ Particle Data Group (2008), "Review of Particle Physics", Phys. Lett. B 667 (1–5): 1–6, doi:10.1016/j.physletb.2008.07.018, Bibcode: 2008PhLB..667....1A, https://www.zora.uzh.ch/id/eprint/11249/2/scalarsV.pdf, retrieved 2019-12-13. Data tables.
- ↑ "CODATA Recommended Values of the Fundamental Physical Constants: 2006". Reviews of Modern Physics 80 (2): 633–730. 2008. doi:10.1103/RevModPhys.80.633. Bibcode: 2008RvMP...80..633M. https://www.nist.gov/pml/div684/fcdc/upload/rmp2006-2.pdf. Direct link to value.
- ↑ Fewell, M. P. (1995), "The atomic nuclide with the highest mean binding energy", Am. J. Phys. 63 (7): 653–58, doi:10.1119/1.17828, Bibcode: 1995AmJPh..63..653F.
![]() | Original source: https://en.wikipedia.org/wiki/Iron group.
Read more |