Biology:Behavioral epigenetics
Behavioral epigenetics is the field of study examining the role of epigenetics in shaping animal and human behavior.[1] It seeks to explain how nurture shapes nature,[2] where nature refers to biological heredity[3] and nurture refers to virtually everything that occurs during the life-span (e.g., social-experience, diet and nutrition, and exposure to toxins).[4] Behavioral epigenetics attempts to provide a framework for understanding how the expression of genes is influenced by experiences and the environment[5] to produce individual differences in behaviour,[6] cognition,[2] personality,[7] and mental health.[8][9]
Epigenetic gene regulation involves changes other than to the sequence of DNA and includes changes to histones (proteins around which DNA is wrapped) and DNA methylation.[10][4][11] These epigenetic changes can influence the growth of neurons in the developing brain[12] as well as modify the activity of neurons in the adult brain.[13][14] Together, these epigenetic changes in neuron structure and function can have a marked influence on an organism's behavior.[1]
Background
In biology, and specifically genetics, epigenetics is the study of heritable changes in gene activity which are not caused by changes in the DNA sequence; the term can also be used to describe the study of stable, long-term alterations in the transcriptional potential of a cell that are not necessarily heritable.[15][16]
Examples of mechanisms that produce such changes are DNA methylation[17] and histone modification,[18] each of which alters how genes are expressed without altering the underlying DNA sequence. Gene expression can be controlled through the action of repressor proteins that attach to silencer regions of the DNA.
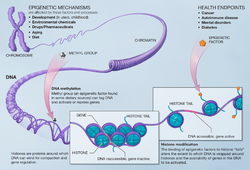
DNA methylation turns a gene "off" – it results in the inability of genetic information to be read from DNA; removing the methyl tag can turn the gene back "on".[19][20]
Histone modification changes the way that DNA is packaged into chromosomes. These changes impact how genes are expressed.[21]
Epigenetics has a strong influence on the development of an organism and can alter the expression of individual traits.[11] Epigenetic changes occur not only in the developing fetus, but also in individuals throughout the human life-span.[4][22] Because some epigenetic modifications can be passed from one generation to the next,[23] subsequent generations may be affected by the epigenetic changes that took place in the parents.[23]
Discovery
The first documented example of epigenetics affecting behavior was provided by Michael Meaney and Moshe Szyf.[24] While working at McGill University in Montréal in 2004, they discovered that the type and amount of nurturing a mother rat provides in the early weeks of the rat's infancy determines how that rat responds to stress later in life.[4] This stress sensitivity was linked to a down-regulation in the expression of the glucocorticoid receptor in the brain. In turn, this down-regulation was found to be a consequence of the extent of methylation in the promoter region of the glucocorticoid receptor gene.[1] Immediately after birth, Meaney and Szyf found that methyl groups repress the glucocorticoid receptor gene in all rat pups, making the gene unable to unwind from the histone in order to be transcribed, causing a decreased stress response. Nurturing behaviours from the mother rat were found to stimulate activation of stress signalling pathways that remove methyl groups from DNA. This releases the tightly wound gene, exposing it for transcription. The glucocorticoid gene is activated, resulting in lowered stress response. Rat pups that receive a less nurturing upbringing are more sensitive to stress throughout their life-span.
This pioneering work in rodents has been difficult to replicate in humans because of a general lack of availability of human brain tissue for measurement of epigenetic changes.[1]
Research into epigenetics in psychology
Anxiety and risk-taking
In a small clinical study in humans published in 2008,[25] epigenetic differences were linked to differences in risk-taking and reactions to stress in monozygotic twins.[25] The study identified twins with different life paths, wherein one twin displayed risk-taking behaviours, and the other displayed risk-averse behaviours. Epigenetic differences in DNA methylation of the CpG islands proximal to the DLX1 gene correlated with the differing behavior.[25] The authors of the twin study noted that despite the associations between epigenetic markers and differences personality traits, epigenetics cannot predict complex decision-making processes like career selection.[25]
Stress

Animal and human studies have found correlations between poor care during infancy and epigenetic changes that correlate with long-term impairments that result from neglect.[26][27][28]
Studies in rats have shown correlations between maternal care in terms of the parental licking of offspring and epigenetic changes.[26] A high level of licking results in a long-term reduction in stress response as measured behaviorally and biochemically in elements of the hypothalamic-pituitary-adrenal axis (HPA). Further, decreased DNA methylation of the glucocorticoid receptor gene were found in offspring that experienced a high level of licking; the glucorticoid receptor plays a key role in regulating the HPA.[26] The opposite is found in offspring that experienced low levels of licking, and when pups are switched, the epigenetic changes are reversed. This research provides evidence for an underlying epigenetic mechanism.[26] Further support comes from experiments with the same setup, using drugs that can increase or decrease methylation.[27] Finally, epigenetic variations in parental care can be passed down from one generation to the next, from mother to female offspring. Female offspring who received increased parental care (i.e., high licking) became mothers who engaged in high licking and offspring who received less licking became mothers who engaged in less licking.[26]
In humans, a small clinical research study showed the relationship between prenatal exposure to maternal mood and genetic expression resulting in increased reactivity to stress in offspring.[4] Three groups of infants were examined: those born to mothers medicated for depression with serotonin reuptake inhibitors; those born to depressed mothers not being treated for depression; and those born to non-depressed mothers. Prenatal exposure to depressed/anxious mood was associated with increased DNA methylation at the glucocorticoid receptor gene and to increased HPA axis stress reactivity.[26] The findings were independent of whether the mothers were being pharmaceutically treated for depression.[26]
Recent research has also shown the relationship of methylation of the maternal glucocorticoid receptor and maternal neural activity in response to mother-infant interactions on video.[29] Longitudinal follow-up of those infants will be important to understand the impact of early caregiving in this high-risk population on child epigenetics and behavior.
Cognition
Learning and memory
A 2010 review discussed the role of DNA methylation in memory formation and storage, but the precise mechanisms involving neuronal function, memory, and methylation reversal remained unclear at the time.[30]
Further research investigated the molecular basis for long-term memory. By 2015 it had become clear that long-term memory requires gene transcription activation and de novo protein synthesis.[31] Long-term memory formation depends on both the activation of memory promoting genes and the inhibition of memory suppressor genes, and DNA methylation/DNA demethylation was found to be a major mechanism for achieving this dual regulation.[32]
Rats with a new, strong long-term memory due to contextual fear conditioning have reduced expression of about 1,000 genes and increased expression of about 500 genes in the hippocampus of the brain 24 hours after training, thus exhibiting modified expression of 9.17% of the rat hippocampal genome. Reduced gene expressions were associated with methylations of those genes and hypomethylation was found for genes involved in synaptic transmission and neuronal differentiation.[33]
Further research into long-term memory has shed light on the molecular mechanisms by which methylation is created or removed, as reviewed in 2022.[34] These mechanisms include, for instance, signal-responsive TOP2B-induced double-strand breaks in immediate early genes. More than 100 DNA double-strand breaks occur, both in the hippocampus and in the medial prefrontal cortex (mPFC), in two peaks, at 10 minutes and at 30 minutes after contextual fear conditioning.[35] This appears to be earlier than the DNA methylations and demethylations of neuron DNA in the hippocampus that were measured at one hour and 24 hours after contextual fear conditioning.
The double strand breaks occur at known memory-related immediate early genes (among other genes) in neurons after neuron activation.[36][35] These double-strand breaks allow the genes to be transcribed and then translated into active proteins.
One immediate early gene newly transcribed after a double-strand break is EGR1. EGR1 is an important transcription factor in memory formation. It has an essential role in brain neuron epigenetic reprogramming. EGR1 recruits the TET1 protein that initiates a pathway of DNA demethylation. Removing DNA methylation marks allows the activation of downstream genes (see Regulation of gene expression. EGR1 brings TET1 to promoter sites of genes that need to be demethylated and activated (transcribed) during memory formation.[37] EGR-1, together with TET1, is employed in programming the distribution of DNA demethylation sites on brain DNA during memory formation and in long-term neuronal plasticity.[37]
DNMT3A2 is another immediate early gene whose expression in neurons can be induced by sustained synaptic activity.[38] DNMTs bind to DNA and methylate cytosines at particular locations in the genome. If this methylation is prevented by DNMT inhibitors, then memories do not form.[39] If DNMT3A2 is over-expressed in the hippocampus of young adult mice it converts a weak learning experience into long-term memory and also enhances fear memory formation.[32]
In another mechanism reviewed in 2022,[34] the messenger RNAs of many genes that had been subjected to methylation-controlled increases or decreases are transported by neural granules (messenger RNPs) to the dendritic spines. At these locations the messenger RNAs can be translated into the proteins that control signaling at neuronal synapses.
Studies in rodents have found that the environment exerts an influence on epigenetic changes related to cognition, in terms of learning and memory;[4] environmental enrichment correlated with increased histone acetylation, and verification by administering histone deacetylase inhibitors induced sprouting of dendrites, an increased number of synapses, and reinstated learning behaviour and access to long-term memories.[1][40] Research has also linked learning and long-term memory formation to reversible epigenetic changes in the hippocampus and cortex in animals with normal-functioning, non-damaged brains.[1][41] In human studies, post-mortem brains from Alzheimer's patients show increased histone de-acetylase levels.[42][43]
Psychopathology and mental health
Drug addiction
![]() |
Environmental and epigenetic influences seem to work together to increase the risk of addiction.[51] For example, environmental stress has been shown to increase the risk of substance abuse.[52] In an attempt to cope with stress, alcohol and drugs can be used as an escape.[53] Once substance abuse commences, however, epigenetic alterations may further exacerbate the biological and behavioural changes associated with addiction.[51]
Even short-term substance abuse can produce long-lasting epigenetic changes in the brain of rodents,[51] via DNA methylation and histone modification.[18] Epigenetic modifications have been observed in studies on rodents involving ethanol, nicotine, cocaine, amphetamine, methamphetamine and opiates.[4] Specifically, these epigenetic changes modify gene expression, which in turn increases the vulnerability of an individual to engage in repeated substance overdose in the future. In turn, increased substance abuse results in even greater epigenetic changes in various components of a rodent's reward system[51] (e.g., in the nucleus accumbens[54]). Hence, a cycle emerges whereby changes in areas of the reward system contribute to the long-lasting neural and behavioural changes associated with the increased likelihood of addiction, the maintenance of addiction and relapse.[51] In humans, alcohol consumption has been shown to produce epigenetic changes that contribute to the increased craving of alcohol. As such, epigenetic modifications may play a part in the progression from the controlled intake to the loss of control of alcohol consumption.[55] These alterations may be long-term, as is evidenced in smokers who still possess nicotine-related epigenetic changes ten years after cessation.[56] Therefore, epigenetic modifications[51] may account for some of the behavioural changes generally associated with addiction. These include: repetitive habits that increase the risk of disease, and personal and social problems; need for immediate gratification; high rates of relapse following treatment; and, the feeling of loss of control.[57]
Evidence for relevant epigenetic changes came from human studies involving alcohol,[58] nicotine, and opiate abuse. Evidence for epigenetic changes stemming from amphetamine and cocaine abuse derives from animal studies. In animals, drug-related epigenetic changes in fathers have also been shown to negatively affect offspring in terms of poorer spatial working memory, decreased attention and decreased cerebral volume.[59]
Imprecise DNA repair can leave epigenetic scars
DNA damage is increased in the brain of rodents by administration of the addictive substances cocaine,[60] methamphetamine,[61][62] alcohol[63] and tobacco smoke.[64] When such DNA damages are repaired, imprecise DNA repair may lead to persistent alterations such as methylation of DNA or the acetylation or methylation of histones at the sites of repair.[65] These alterations may be epigenetic scars in the chromatin that contribute to the persistent epigenetic changes found in addiction.
Eating disorders and obesity
Epigenetic changes may help to facilitate the development and maintenance of eating disorders via influences in the early environment and throughout the life-span.[22] Pre-natal epigenetic changes due to maternal stress, behaviour and diet may later predispose offspring to persistent, increased anxiety and anxiety disorders. These anxiety issues can precipitate the onset of eating disorders and obesity, and persist even after recovery from the eating disorders.[66]
Epigenetic differences accumulating over the life-span may account for the incongruent differences in eating disorders observed in monozygotic twins. At puberty, sex hormones may exert epigenetic changes (via DNA methylation) on gene expression, thus accounting for higher rates of eating disorders in men as compared to women [citation needed]. Overall, epigenetics contribute to persistent, unregulated self-control behaviours related to the urge to binge.[22]
Schizophrenia
Epigenetic changes including hypomethylation of glutamatergic genes (i.e., NMDA-receptor-subunit gene NR3B and the promoter of the AMPA-receptor-subunit gene GRIA2) in the post-mortem brains of people with schizophrenia are associated with increased levels of the neurotransmitter glutamate.[67] Since glutamate is the most prevalent, fast, excitatory neurotransmitter, increased levels may result in the psychotic episodes related to schizophrenia. Epigenetic changes affecting a greater number of genes have been detected in men with schizophrenia as compared to women with the illness.[68]
Population studies have established a strong association linking schizophrenia in children born to older fathers.[69][70] Specifically, children born to fathers over the age of 35 years are up to three times more likely to develop schizophrenia.[70] Epigenetic dysfunction in human male sperm cells, affecting numerous genes, have been shown to increase with age. This provides a possible explanation for increased rates of the disease in men.[68][70][failed verification] To this end, toxins[68][70] (e.g., air pollutants) have been shown to increase epigenetic differentiation. Animals exposed to ambient air from steel mills and highways show drastic epigenetic changes that persist after removal from the exposure.[71] Therefore, similar epigenetic changes in older human fathers are likely.[70] Schizophrenia studies provide evidence that the nature versus nurture debate in the field of psychopathology should be re-evaluated to accommodate the concept that genes and the environment work in tandem. As such, many other environmental factors (e.g., nutritional deficiencies and cannabis use) have been proposed to increase the susceptibility of psychotic disorders like schizophrenia via epigenetics.[70]
Bipolar disorder
Evidence for epigenetic modifications for bipolar disorder is unclear.[72] One study found hypomethylation of a gene promoter of a prefrontal lobe enzyme (i.e., membrane-bound catechol-O-methyl transferase, or COMT) in post-mortem brain samples from individuals with bipolar disorder. COMT is an enzyme that metabolizes dopamine in the synapse. These findings suggest that the hypomethylation of the promoter results in over-expression of the enzyme. In turn, this results in increased degradation of dopamine levels in the brain. These findings provide evidence that epigenetic modification in the prefrontal lobe is a risk factor for bipolar disorder.[73] However, a second study found no epigenetic differences in post-mortem brains from bipolar individuals.[74]
Major depressive disorder
The causes of major depressive disorder (MDD) are poorly understood from a neuroscience perspective.[75] The epigenetic changes leading to changes in glucocorticoid receptor expression and its effect on the HPA stress system discussed above, have also been applied to attempts to understand MDD.[76]
Much of the work in animal models has focused on the indirect downregulation of brain derived neurotrophic factor (BDNF) by over-activation of the stress axis.[77][78] Studies in various rodent models of depression, often involving induction of stress, have found direct epigenetic modulation of BDNF as well.[79]
Psychopathy
Epigenetics may be relevant to aspects of psychopathic behaviour through methylation and histone modification.[80] These processes are heritable but can also be influenced by environmental factors such as smoking and abuse.[81] Epigenetics may be one of the mechanisms through which the environment can impact the expression of the genome.[82] Studies have also linked methylation of genes associated with nicotine and alcohol dependence in women, ADHD, and drug abuse.[83][84][85] It is probable that epigenetic regulation as well as methylation profiling will play an increasingly important role in the study of the play between the environment and genetics of psychopaths.[86]
Suicide
A study of the brains of 24 who died by suicide, 12 of whom had a history of child abuse and 12 who did not, found decreased levels of glucocorticoid receptor in victims of child abuse and associated epigenetic changes.[87]
Social insects
Several studies have indicated DNA cytosine methylation linked to the social behavior of insects, such as honeybees and ants. In honeybees, when nurse bee switched from her in-hive tasks to out foraging, cytosine methylation marks are changing. When a forager bee was reversed to do nurse duties, the cytosine methylation marks were also reversed.[88] Knocking down the DNMT3 in the larvae changed the worker to queen-like phenotype.[89] Queen and worker are two distinguish castes with different morphology, behavior, and physiology. Studies in DNMT3 silencing also indicated DNA methylation may regulate gene alternative splicing and pre-mRNA maturation.[90]
Limitations and future direction
Many researchers contribute information to the Human Epigenome Consortium.[91] The aim of future research is to reprogram epigenetic changes to help with addiction, mental illness, age related changes,[2] memory decline, and other issues.[1] However, the sheer volume of consortium-based data makes analysis difficult.[2] Most studies also focus on one gene.[92] In actuality, many genes and interactions between them likely contribute to individual differences in personality, behaviour and health.[93] As social scientists often work with many variables, determining the number of affected genes also poses methodological challenges. More collaboration between medical researchers, geneticists and social scientists has been advocated to increase knowledge in this field of study.[94]
Limited access to human brain tissue poses a challenge to conducting human research.[2] Not yet knowing if epigenetic changes in the blood and (non-brain) tissues parallel modifications in the brain, places even greater reliance on brain research.[91] Although some epigenetic studies have translated findings from animals to humans,[87] a some researchers caution about the extrapolation of animal studies to humans.[1] One view notes that when animal studies do not consider how the subcellular and cellular components, organs and the entire individual interact with the influences of the environment, results are too reductive to explain behaviour.[93]
Some researchers note that epigenetic perspectives will likely be incorporated into pharmacological treatments.[8] Others caution that more research is necessary as drugs are known to modify the activity of multiple genes and may, therefore, cause serious side effects.[1] However, the ultimate goal is to find patterns of epigenetic changes that can be targeted to treat mental illness, and reverse the effects of childhood stressors, for example. If such treatable patterns eventually become well-established, the inability to access brains in living humans to identify them poses an obstacle to pharmacological treatment.[91] Future research may also focus on epigenetic changes that mediate the impact of psychotherapy on personality and behaviour.[26]
Most epigenetic research is correlational; it merely establishes associations. More experimental research is necessary to help establish causation.[95] Lack of resources has also limited the number of intergenerational studies.[2] Therefore, advancing longitudinal[94] and multigenerational, experience-dependent studies will be critical to further understanding the role of epigenetics in psychology.[5]
See also
- Behavioral genetics
- Behavioral neuroscience
- Epigenetics of anxiety and stress-related disorders
- Evolutionary neuroscience
- Neuroscience
- Personality psychology
References
- ↑ 1.0 1.1 1.2 1.3 1.4 1.5 1.6 1.7 1.8 "Epigenetics. The seductive allure of behavioral epigenetics". Science 329 (5987): 24–7. Jul 2010. doi:10.1126/science.329.5987.24. PMID 20595592. Bibcode: 2010Sci...329...24M.
- ↑ 2.0 2.1 2.2 2.3 2.4 2.5 "Behavioral epigenetics: How nurture shapes nature". BioScience 61 (8): 588–592. 2011. doi:10.1525/bio.2011.61.8.4.
- ↑ Children and Their Development, Second Canadian Edition with MyDevelopmentLab. Toronto: Pearson Education Canada. 2011. ISBN 978-0-13-255770-2.
- ↑ 4.0 4.1 4.2 4.3 4.4 4.5 4.6 The Developing Genome: An Introduction to Behavioral Epigenetics (1st ed.). Oxford University Press. 2015. ISBN 978-0-19-992234-5.
- ↑ 5.0 5.1 "Genes in context: Gene-environment interplay and the origins of individual differences in behaviour". Current Directions in Psychological Science 18 (3): 127–131. 2012. doi:10.1111/j.1467-8721.2009.01622.x.
- ↑ "Epigenetics and the environmental regulation of the genome and its function". Annual Review of Psychology 61: 439–66, C1-3. 2010. doi:10.1146/annurev.psych.60.110707.163625. PMID 19958180.
- ↑ "Epigenetics and the biological basis of gene x environment interactions". Journal of the American Academy of Child and Adolescent Psychiatry 49 (8): 752–71. Aug 2010. doi:10.1016/j.jaac.2010.06.001. PMID 20643310.
- ↑ 8.0 8.1 "Role of epigenetics in mental disorders". The Australian and New Zealand Journal of Psychiatry 42 (2): 97–107. Feb 2008. doi:10.1080/00048670701787495. PMID 18197504. https://zenodo.org/record/3948906.
- ↑ "Epigenomic profiling reveals DNA-methylation changes associated with major psychosis". American Journal of Human Genetics 82 (3): 696–711. Mar 2008. doi:10.1016/j.ajhg.2008.01.008. PMID 18319075.
- ↑ "Crime investigation through DNA methylation analysis: methods and applications in forensics". Egyptian Journal of Forensic Sciences 8 (1). 24 January 2018. doi:10.1186/s41935-018-0042-1.
- ↑ 11.0 11.1 "Behind the scenes of gene expression". Science 293 (5532): 1064–7. Aug 2001. doi:10.1126/science.293.5532.1064. PMID 11498570.
- ↑ "Epigenetic regulation in neural stem cell differentiation". Development, Growth & Differentiation 52 (6): 493–504. Aug 2010. doi:10.1111/j.1440-169X.2010.01175.x. PMID 20608952.
- ↑ "Epigenetic choreographers of neurogenesis in the adult mammalian brain". Nature Neuroscience 13 (11): 1338–44. Nov 2010. doi:10.1038/nn.2672. PMID 20975758.
- ↑ "Epigenetic regulation of neurogenesis in the adult mammalian brain". The European Journal of Neuroscience 33 (6): 1087–93. Mar 2011. doi:10.1111/j.1460-9568.2011.07607.x. PMID 21395852.
- ↑ "Perceptions of epigenetics". Nature 447 (7143): 396–8. May 2007. doi:10.1038/nature05913. PMID 17522671. Bibcode: 2007Natur.447..396B.
- ↑ "Overview of the Roadmap Epigenomics Project". http://www.roadmapepigenomics.org/overview.
- ↑ "Epigenetic principles and mechanisms underlying nervous system functions in health and disease". Progress in Neurobiology 86 (4): 305–41. Dec 2008. doi:10.1016/j.pneurobio.2008.10.001. PMID 18940229.
- ↑ 18.0 18.1 "The epigenetic landscape of addiction". Annals of the New York Academy of Sciences 1216 (1): 99–113. Jan 2011. doi:10.1111/j.1749-6632.2010.05893.x. PMID 21272014. Bibcode: 2011NYASA1216...99M.
- ↑ "Stability and flexibility of epigenetic gene regulation in mammalian development". Nature 447 (7143): 425–32. May 2007. doi:10.1038/nature05918. PMID 17522676. Bibcode: 2007Natur.447..425R.
- ↑ "Human development: biological and genetic processes". Annual Review of Psychology 56: 263–86. 2005. doi:10.1146/annurev.psych.56.091103.070208. PMID 15709936.
- ↑ "Histone modifications | Abcam". https://www.abcam.com/epigenetics/histone-modifications.
- ↑ 22.0 22.1 22.2 "Eating disorders, gene-environment interactions and epigenetics". Neuroscience and Biobehavioral Reviews 35 (3): 784–93. Jan 2011. doi:10.1016/j.neubiorev.2010.09.012. PMID 20888360.
- ↑ 23.0 23.1 "Regulation of X-chromosome inactivation in development in mice and humans". Microbiology and Molecular Biology Reviews 62 (2): 362–78. Jun 1998. doi:10.1128/MMBR.62.2.362-378.1998. PMID 9618446.
- ↑ "Epigenetic programming by maternal behavior". Nature Neuroscience 7 (8): 847–54. August 2004. doi:10.1038/nn1276. PMID 15220929.
- ↑ 25.0 25.1 25.2 25.3 "Epigenetics of personality traits: an illustrative study of identical twins discordant for risk-taking behavior". Twin Research and Human Genetics 11 (1): 1–11. February 2008. doi:10.1375/twin.11.1.1. PMID 18251670.
- ↑ 26.0 26.1 26.2 26.3 26.4 26.5 26.6 26.7 "Psychology and epigenetics". Review of General Psychology 13 (3): 194–201. 2009. doi:10.1037/a0016301.
- ↑ 27.0 27.1 "The social environment and the epigenome". Environmental and Molecular Mutagenesis 49 (1): 46–60. Jan 2008. doi:10.1002/em.20357. PMID 18095330. Bibcode: 2008EnvMM..49...46S.
- ↑ "Epigenetics and its implications for Psychology". Psicothema 25 (1): 3–12. Feb 2013. doi:10.7334/psicothema2012.327. PMID 23336536.
- ↑ Schechter DS, Moser DA, Giacobino A, Stenz L, Gex-Fabry M, Adouan W, Cordero MI, Suardi F, Manini A, Sancho-Rossignol A, Merminod G, Aue T, Ansermet F, Dayer AG, Rusconi-Serpa S. (epub May 29, 2015) Methylation of NR3C1 is related to maternal PTSD, parenting stress and maternal medial prefrontal cortical activity in response to child separation among mothers with histories of violence exposure. Frontiers in Psychology. http://journal.frontiersin.org/article/10.3389/fpsyg.2015.00690/abstract
- ↑ "DNA methylation and memory formation". Nature Neuroscience 13 (11): 1319–23. Nov 2010. doi:10.1038/nn.2666. PMID 20975755.
- ↑ "The regulation of transcription in memory consolidation". Cold Spring Harb Perspect Biol 7 (1): a021741. December 2014. doi:10.1101/cshperspect.a021741. PMID 25475090.
- ↑ 32.0 32.1 "DNA methylation: a permissive mark in memory formation and maintenance". Learn Mem 23 (10): 587–93. October 2016. doi:10.1101/lm.042739.116. PMID 27634149.
- ↑ "Experience-dependent epigenomic reorganization in the hippocampus". Learn Mem 24 (7): 278–288. July 2017. doi:10.1101/lm.045112.117. PMID 28620075.
- ↑ 34.0 34.1 "DNA Methylation and Establishing Memory". Epigenet Insights 15: 25168657211072499. 2022. doi:10.1177/25168657211072499. PMID 35098021.
- ↑ 35.0 35.1 "Profiling DNA break sites and transcriptional changes in response to contextual fear learning". PLOS ONE 16 (7): e0249691. 2021. doi:10.1371/journal.pone.0249691. PMID 34197463. Bibcode: 2021PLoSO..1649691S.
- ↑ "Activity-Induced DNA Breaks Govern the Expression of Neuronal Early-Response Genes". Cell 161 (7): 1592–605. June 2015. doi:10.1016/j.cell.2015.05.032. PMID 26052046.
- ↑ 37.0 37.1 "EGR1 recruits TET1 to shape the brain methylome during development and upon neuronal activity". Nat Commun 10 (1): 3892. August 2019. doi:10.1038/s41467-019-11905-3. PMID 31467272. Bibcode: 2019NatCo..10.3892S.
- ↑ "Rescue of aging-associated decline in Dnmt3a2 expression restores cognitive abilities". Nat Neurosci 15 (8): 1111–3. July 2012. doi:10.1038/nn.3151. PMID 22751036.
- ↑ "Neuronal DNA Methyltransferases: Epigenetic Mediators between Synaptic Activity and Gene Expression?". Neuroscientist 24 (2): 171–185. April 2018. doi:10.1177/1073858417707457. PMID 28513272.
- ↑ "Recovery of learning and memory is associated with chromatin remodelling". Nature 447 (7141): 178–82. May 2007. doi:10.1038/nature05772. PMID 17468743. Bibcode: 2007Natur.447..178F.
- ↑ "Histone methylation regulates memory formation". The Journal of Neuroscience 30 (10): 3589–99. Mar 2010. doi:10.1523/JNEUROSCI.3732-09.2010. PMID 20219993.
- ↑ "An epigenetic blockade of cognitive functions in the neurodegenerating brain". Nature 483 (7388): 222–6. Mar 2012. doi:10.1038/nature10849. PMID 22388814. Bibcode: 2012Natur.483..222G.
- ↑ "Altered histone acetylation is associated with age-dependent memory impairment in mice". Science 328 (5979): 753–6. May 2010. doi:10.1126/science.1186088. PMID 20448184. Bibcode: 2010Sci...328..753P.
- ↑ 44.0 44.1 44.2 Renthal W, Nestler EJ (September 2009). "Chromatin regulation in drug addiction and depression". Dialogues in Clinical Neuroscience 11 (3): 257–268. PMID 19877494. "[Psychostimulants] increase cAMP levels in striatum, which activates protein kinase A (PKA) and leads to phosphorylation of its targets. This includes the cAMP response element binding protein (CREB), the phosphorylation of which induces its association with the histone acetyltransferase, CREB binding protein (CBP) to acetylate histones and facilitate gene activation. This is known to occur on many genes including fosB and c-fos in response to psychostimulant exposure. ΔFosB is also upregulated by chronic psychostimulant treatments, and is known to activate certain genes (eg, cdk5) and repress others (eg, c-fos) where it recruits HDAC1 as a corepressor. ... Chronic exposure to psychostimulants increases glutamatergic [signaling] from the prefrontal cortex to the NAc. Glutamatergic signaling elevates Ca2+ levels in NAc postsynaptic elements where it activates CaMK (calcium/calmodulin protein kinases) signaling, which, in addition to phosphorylating CREB, also phosphorylates HDAC5.".
Figure 2: Psychostimulant-induced signaling events - ↑ "Co-transmission of dopamine and glutamate". The Journal of General Physiology 139 (1): 93–96. January 2012. doi:10.1085/jgp.201110659. PMID 22200950. "Coincident and convergent input often induces plasticity on a postsynaptic neuron. The NAc integrates processed information about the environment from basolateral amygdala, hippocampus, and prefrontal cortex (PFC), as well as projections from midbrain dopamine neurons. Previous studies have demonstrated how dopamine modulates this integrative process. For example, high frequency stimulation potentiates hippocampal inputs to the NAc while simultaneously depressing PFC synapses (Goto and Grace, 2005). The converse was also shown to be true; stimulation at PFC potentiates PFC–NAc synapses but depresses hippocampal–NAc synapses. In light of the new functional evidence of midbrain dopamine/glutamate co-transmission (references above), new experiments of NAc function will have to test whether midbrain glutamatergic inputs bias or filter either limbic or cortical inputs to guide goal-directed behavior.".
- ↑ Kanehisa Laboratories (10 October 2014). "Amphetamine – Homo sapiens (human)". KEGG Pathway. http://www.genome.jp/kegg-bin/show_pathway?hsa05031+2354. Retrieved 31 October 2014. "Most addictive drugs increase extracellular concentrations of dopamine (DA) in nucleus accumbens (NAc) and medial prefrontal cortex (mPFC), projection areas of mesocorticolimbic DA neurons and key components of the "brain reward circuit". Amphetamine achieves this elevation in extracellular levels of DA by promoting efflux from synaptic terminals. ... Chronic exposure to amphetamine induces a unique transcription factor delta FosB, which plays an essential role in long-term adaptive changes in the brain."
- ↑ "Transcriptional and epigenetic substrates of methamphetamine addiction and withdrawal: evidence from a long-access self-administration model in the rat". Molecular Neurobiology 51 (2): 696–717. 2015. doi:10.1007/s12035-014-8776-8. PMID 24939695. "Figure 1".
- ↑ 48.0 48.1 48.2 Robison AJ, Nestler EJ (November 2011). "Transcriptional and epigenetic mechanisms of addiction". Nature Reviews Neuroscience 12 (11): 623–637. doi:10.1038/nrn3111. PMID 21989194. "ΔFosB serves as one of the master control proteins governing this structural plasticity. ... ΔFosB also represses G9a expression, leading to reduced repressive histone methylation at the cdk5 gene. The net result is gene activation and increased CDK5 expression. ... In contrast, ΔFosB binds to the c-fos gene and recruits several co-repressors, including HDAC1 (histone deacetylase 1) and SIRT 1 (sirtuin 1). ... The net result is c-fos gene repression.".
Figure 4: Epigenetic basis of drug regulation of gene expression - ↑ 49.0 49.1 49.2 Nestler EJ (December 2012). "Transcriptional mechanisms of drug addiction". Clinical Psychopharmacology and Neuroscience 10 (3): 136–143. doi:10.9758/cpn.2012.10.3.136. PMID 23430970. "The 35-37 kD ΔFosB isoforms accumulate with chronic drug exposure due to their extraordinarily long half-lives. ... As a result of its stability, the ΔFosB protein persists in neurons for at least several weeks after cessation of drug exposure. ... ΔFosB overexpression in nucleus accumbens induces NFκB ... In contrast, the ability of ΔFosB to repress the c-Fos gene occurs in concert with the recruitment of a histone deacetylase and presumably several other repressive proteins such as a repressive histone methyltransferase".
- ↑ Nestler EJ (October 2008). "Transcriptional mechanisms of addiction: Role of ΔFosB". Philosophical Transactions of the Royal Society B: Biological Sciences 363 (1507): 3245–3255. doi:10.1098/rstb.2008.0067. PMID 18640924. "Recent evidence has shown that ΔFosB also represses the c-fos gene that helps create the molecular switch—from the induction of several short-lived Fos family proteins after acute drug exposure to the predominant accumulation of ΔFosB after chronic drug exposure".
- ↑ 51.0 51.1 51.2 51.3 51.4 51.5 "Drugs and addiction: an introduction to epigenetics". Addiction 106 (3): 480–9. Mar 2011. doi:10.1111/j.1360-0443.2010.03321.x. PMID 21205049.
- ↑ "Desperately driven and no brakes: developmental stress exposure and subsequent risk for substance abuse". Neuroscience and Biobehavioral Reviews 33 (4): 516–24. Apr 2009. doi:10.1016/j.neubiorev.2008.09.009. PMID 18938197.
- ↑ "Coping". The Handbook of Stress Science: Biology, Psychology, and Health. New York: Springer Publishing Company. 2010. pp. 223. ISBN 978-0-8261-1471-6.
- ↑ "Epigenetic mechanisms in drug addiction". Trends in Molecular Medicine 14 (8): 341–50. Aug 2008. doi:10.1016/j.molmed.2008.06.004. PMID 18635399.
- ↑ "Abstracts of ESBRA 2011, European Society for Biomedical Research on Alcoholism. Vienna, Austria. September 4–7, 2011". Alcohol and Alcoholism 46 (Suppl 1): i1-63. 2011. doi:10.1093/alcalc/agr085. PMID 21863600.
- ↑ "Smoking induces long-lasting effects through a monoamine-oxidase epigenetic regulation". PLOS ONE 4 (11): e7959. 2009. doi:10.1371/journal.pone.0007959. PMID 19956754. Bibcode: 2009PLoSO...4.7959L.
- ↑ "Addictive behaviors: etiology and treatment". Annual Review of Psychology 39: 223–52. 1988. doi:10.1146/annurev.ps.39.020188.001255. PMID 3278676.
- ↑ "Homocysteine associated genomic DNA hypermethylation in patients with chronic alcoholism". Journal of Neural Transmission 111 (12): 1611–6. Dec 2004. doi:10.1007/s00702-004-0232-x. PMID 15565495.
- ↑ "Consequences of paternal cocaine exposure in mice". Neurotoxicology and Teratology 28 (2): 198–209. 2006. doi:10.1016/j.ntt.2005.12.003. PMID 16458479.
- ↑ "Cocaine induces DNA damage in distinct brain areas of female rats under different hormonal conditions". Clinical and Experimental Pharmacology & Physiology 41 (4): 265–269. April 2014. doi:10.1111/1440-1681.12218. PMID 24552452.
- ↑ "Methamphetamine induces DNA damage in specific regions of the female rat brain". Clinical and Experimental Pharmacology & Physiology 42 (6): 570–575. June 2015. doi:10.1111/1440-1681.12404. PMID 25867833.
- ↑ "The peroxidative DNA damage and apoptosis in methamphetamine-treated rat brain". The Journal of Medical Investigation 55 (3–4): 241–245. August 2008. doi:10.2152/jmi.55.241. PMID 18797138.
- ↑ "Alcohol induces DNA damage and the Fanconi anemia D2 protein implicating FANCD2 in the DNA damage response pathways in brain". Alcoholism: Clinical and Experimental Research 32 (7): 1186–1196. July 2008. doi:10.1111/j.1530-0277.2008.00673.x. PMID 18482162.
- ↑ "Biomarkers of disease can be detected in mice as early as 4 weeks after initiation of exposure to third-hand smoke levels equivalent to those found in homes of smokers". Clinical Science (London, England) 131 (19): 2409–2426. October 2017. doi:10.1042/CS20171053. PMID 28912356.
- ↑ "Epigenome Maintenance in Response to DNA Damage". Molecular Cell 62 (5): 712–727. June 2016. doi:10.1016/j.molcel.2016.04.006. PMID 27259203.
- ↑ "Influence of overanxious disorder of childhood on the expression of anorexia nervosa". The International Journal of Eating Disorders 41 (4): 326–32. May 2008. doi:10.1002/eat.20508. PMID 18213688.
- ↑ "Epigenetics in Psychiatry". Epigenetic Aspects of Chronic Diseases. Berlin: Springer. 2011. pp. 163–174. ISBN 978-1-84882-643-4.
- ↑ 68.0 68.1 68.2 "Genetics and epigenetics in major psychiatric disorders: dilemmas, achievements, applications, and future scope". American Journal of Pharmacogenomics 5 (3): 149–60. 2005. doi:10.2165/00129785-200505030-00002. PMID 15952869.
- ↑ "Advancing paternal age and the risk of schizophrenia". Archives of General Psychiatry 58 (4): 361–7. Apr 2001. doi:10.1001/archpsyc.58.4.361. PMID 11296097.
- ↑ 70.0 70.1 70.2 70.3 70.4 70.5 "Epigenetic mediation of environmental influences in major psychotic disorders". Schizophrenia Bulletin 35 (6): 1045–56. Nov 2009. doi:10.1093/schbul/sbp104. PMID 19783603.
- ↑ "Germ-line mutations, DNA damage, and global hypermethylation in mice exposed to particulate air pollution in an urban/industrial location". Proceedings of the National Academy of Sciences of the United States of America 105 (2): 605–10. Jan 2008. doi:10.1073/pnas.0705896105. PMID 18195365. Bibcode: 2008PNAS..105..605Y.
- ↑ "Epigenetics in mood disorders". Environmental Health and Preventive Medicine 13 (1): 16–24. Jan 2008. doi:10.1007/s12199-007-0002-0. PMID 19568875. Bibcode: 2008EHPM...13...16M.
- ↑ "Hypomethylation of MB-COMT promoter is a major risk factor for schizophrenia and bipolar disorder". Human Molecular Genetics 15 (21): 3132–45. Nov 2006. doi:10.1093/hmg/ddl253. PMID 16984965.
- ↑ "The quantification of COMT mRNA in post mortem cerebellum tissue: diagnosis, genotype, methylation and expression". BMC Medical Genetics 7: 10. 2006. doi:10.1186/1471-2350-7-10. PMID 16483362.
- ↑ "Beyond the monoaminergic hypothesis: neuroplasticity and epigenetic changes in a transgenic mouse model of depression". Philosophical Transactions of the Royal Society of London. Series B, Biological Sciences 367 (1601): 2485–94. Sep 2012. doi:10.1098/rstb.2012.0212. PMID 22826347.
- ↑ "The HPA axis in major depression: classical theories and new developments". Trends in Neurosciences 31 (9): 464–8. Sep 2008. doi:10.1016/j.tins.2008.06.006. PMID 18675469.
- ↑ "Sustained hippocampal chromatin regulation in a mouse model of depression and antidepressant action". Nature Neuroscience 9 (4): 519–25. Apr 2006. doi:10.1038/nn1659. PMID 16501568.
- ↑ "Dynamic plasticity: the role of glucocorticoids, brain-derived neurotrophic factor and other trophic factors". Neuroscience 239: 214–27. Jun 2013. doi:10.1016/j.neuroscience.2012.08.034. PMID 22922121.
- ↑ "Epigenetic effects of stress and corticosteroids in the brain". Frontiers in Cellular Neuroscience 6: 18. 2012. doi:10.3389/fncel.2012.00018. PMID 22529779.
- ↑ "Epigenetics in the nervous system". The Journal of Neuroscience 28 (46): 11753–9. Nov 2008. doi:10.1523/JNEUROSCI.3797-08.2008. PMID 19005036.
- ↑ "The effect of smoking on MAOA promoter methylation in DNA prepared from lymphoblasts and whole blood". American Journal of Medical Genetics Part B 153B (2): 619–28. Mar 2010. doi:10.1002/ajmg.b.31031. PMID 19777560.
- ↑ "The relationship of 5HTT (SLC6A4) methylation and genotype on mRNA expression and liability to major depression and alcohol dependence in subjects from the Iowa Adoption Studies". American Journal of Medical Genetics Part B 147B (5): 543–9. Jul 2008. doi:10.1002/ajmg.b.30657. PMID 17987668.
- ↑ "MAOA methylation is associated with nicotine and alcohol dependence in women". American Journal of Medical Genetics Part B 147B (5): 565–70. Jul 2008. doi:10.1002/ajmg.b.30778. PMID 18454435.
- ↑ "Endogenous opiates and behavior: 2009". Peptides 31 (12): 2325–59. Dec 2010. doi:10.1016/j.peptides.2010.09.016. PMID 20875476.
- ↑ "Pre- and peri-natal environmental risks for attention-deficit hyperactivity disorder (ADHD): the potential role of epigenetic processes in mediating susceptibility". Journal of Child Psychology and Psychiatry, and Allied Disciplines 49 (10): 1020–30. Oct 2008. doi:10.1111/j.1469-7610.2008.01909.x. PMID 18492038.
- ↑ "Behavioral genetics in antisocial spectrum disorders and psychopathy: a review of the recent literature". Behavioral Sciences & the Law 28 (2): 148–73. 2010. doi:10.1002/bsl.923. PMID 20422643.
- ↑ 87.0 87.1 "Epigenetic regulation of the glucocorticoid receptor in human brain associates with childhood abuse". Nature Neuroscience 12 (3): 342–8. Mar 2009. doi:10.1038/nn.2270. PMID 19234457.
- ↑ "Reversible switching between epigenetic states in honeybee behavioral subcastes". Nature Neuroscience 15 (10): 1371–3. Oct 2012. doi:10.1038/nn.3218. PMID 22983211.
- ↑ "Nutritional control of reproductive status in honeybees via DNA methylation". Science 319 (5871): 1827–30. Mar 2008. doi:10.1126/science.1153069. PMID 18339900. Bibcode: 2008Sci...319.1827K.
- ↑ "RNA interference knockdown of DNA methyl-transferase 3 affects gene alternative splicing in the honey bee". Proceedings of the National Academy of Sciences of the United States of America 110 (31): 12750–5. Jul 2013. doi:10.1073/pnas.1310735110. PMID 23852726. Bibcode: 2013PNAS..11012750L.
- ↑ 91.0 91.1 91.2 "Epigenetics in mental illness: hope or hype?". Journal of Psychiatry & Neuroscience 35 (6): 366–8. Nov 2010. doi:10.1503/jpn.100148. PMID 20964959.
- ↑ "Genetic epidemiology of stress and gene by stress interaction". The Handbook of Stress Science: Biology, Psychology, and Health. New York: Springer Publishing Company. 2010. pp. 78–85. ISBN 978-0-8261-1471-6.
- ↑ 93.0 93.1 "What neurobiology cannot tell us about addiction". Addiction 105 (5): 780–9. May 2010. doi:10.1111/j.1360-0443.2009.02739.x. PMID 19919596.
- ↑ 94.0 94.1 "Family influences on children's well being: potential roles of molecular genetics and epigenetics". Biosocial Foundations of Family Processes (National Symposium on Family Issues). Berlin: Springer. 2010. pp. 181–204. ISBN 978-1-4419-7360-3.
- ↑ "Disease-associated epigenetic changes in monozygotic twins discordant for schizophrenia and bipolar disorder". Human Molecular Genetics 20 (24): 4786–96. Dec 2011. doi:10.1093/hmg/ddr416. PMID 21908516.
- Image legend
- ↑ (Text color) Transcription factors
Further reading
- "Behavioral epigenetics". Annals of the New York Academy of Sciences 1226 (1): 14–33. May 2011. doi:10.1111/j.1749-6632.2011.06037.x. PMID 21615751. Bibcode: 2011NYASA1226...14L.
- "Behavioral epigenetics: a new frontier in the study of hormones and behavior". Hormones and Behavior 59 (3): 277–8. Mar 2011. doi:10.1016/j.yhbeh.2011.02.011. PMID 21419246.
- "Epigenetics in the mature mammalian brain: effects on behavior and synaptic transmission". Neurobiology of Learning and Memory 96 (1): 53–60. Jul 2011. doi:10.1016/j.nlm.2011.02.015. PMID 21396474.
- "Proteomic investigation of epigenetics in neuropsychiatric disorders: a missing link between genetics and behavior?". Journal of Proteome Research 10 (1): 58–65. Jan 2011. doi:10.1021/pr100463y. PMID 20735116.
- "Social influences on neurobiology and behavior: epigenetic effects during development". Psychoneuroendocrinology 36 (3): 352–71. Apr 2011. doi:10.1016/j.psyneuen.2010.06.005. PMID 20650569.
- "Epigenetic modifications of brain and behavior: theory and practice". Hormones and Behavior 59 (3): 393–8. Mar 2011. doi:10.1016/j.yhbeh.2010.07.001. PMID 20633562.
- "Stress, genetics and epigenetic effects on the neurobiology of suicidal behavior and depression". European Psychiatry 25 (5): 268–71. Jun 2010. doi:10.1016/j.eurpsy.2010.01.009. PMID 20451357.
- "Epigenetics, brain, behavior, and the environment". Hormones 9 (1): 41–50. 2010. doi:10.14310/horm.2002.1251. PMID 20363720.
- "Epigenetic mechanisms underlying extinction of memory and drug-seeking behavior". Mammalian Genome 20 (9–10): 612–23. 2009. doi:10.1007/s00335-009-9224-3. PMID 19789849.
- "Prenatal imprinting of postnatal specific appetites and feeding behavior". Metabolism 57 (Suppl 2): S22-6. Oct 2008. doi:10.1016/j.metabol.2008.07.004. PMID 18803961.
- "Diet and the epigenetic (re)programming of phenotypic differences in behavior". Brain Research 1237: 12–24. Oct 2008. doi:10.1016/j.brainres.2008.07.074. PMID 18694740.
- "Maternal care, the epigenome and phenotypic differences in behavior". Reproductive Toxicology 24 (1): 9–19. Jul 2007. doi:10.1016/j.reprotox.2007.05.001. PMID 17561370.
- "Genomic comparison of the ants Camponotus floridanus and Harpegnathos saltator". Science 329 (5995): 1068–71. Aug 2010. doi:10.1126/science.1192428. PMID 20798317. Bibcode: 2010Sci...329.1068B.
External links
- "The Fingerprints of Poverty". Quirks & Quarks. CBC Radio. 2011. http://www.cbc.ca/video/news/audioplayer.html?clipid=2161607978. "Audio interview with Moshe Szyf, a professor of Pharmacology and Therapeutics at McGill University, discusses how epigenetic changes are related to differences in socioeconomic status."
- "Control Your Pregnancy". The Dr. Oz Show. 2011. http://www.doctoroz.com/videos/control-your-pregnancy. "Video explaining how epigenetics can affect the unborn fetus."
- "Epigenetic Landscapes". 2010. http://www.scivee.tv/node/20905. "This video addresses how, in principle, accumulated epigenetic changes may result in personality differences in identical twins. This video was made by a Ph.D. candidate in experimental medicine and award winning filmmaker Ben Paylor." page text.
- "Epigenetics Explained (Animation)". Scientific American. November 2011. http://www.scientificamerican.com/article.cfm?id=epigenetics-explained. "A series of diagrams explaining how epigenetic marks affect genetic expression.".
![]() | Original source: https://en.wikipedia.org/wiki/Behavioral epigenetics.
Read more |