Chemistry:Lithium nickel manganese cobalt oxides
Lithium nickel manganese cobalt oxides (abbreviated NMC, Li-NMC, LNMC, or NCM) are mixed metal oxides of lithium, nickel, manganese and cobalt with the general formula LiNixMnyCo1-x-yO2. These materials are commonly used in lithium-ion batteries for mobile devices and electric vehicles, acting as the positively charged cathode.
There is a particular interest in optimizing NMC for electric vehicle applications because of the material's high energy density and operating voltage. Reducing the cobalt content in NMC is also a current target, owing to ethical issues with cobalt mining and the metal's high cost.[1] Furthermore, an increased nickel content provides more capacity within the stable operation window.[2]
Structure
NMC materials have layered structures similar to the individual metal oxide compound lithium cobalt oxide (LiCoO2).[3] Lithium ions intercalate between the layers upon discharging, remaining between the lattice planes until the battery gets charged, at which point the lithium de-intercalates and moves to the anode.[4]
Points in a solid solution phase diagram between the end members LiCoO2, LiMnO2, and LiNiO2 represent stoichiometric NMC cathodes.[5] Three numbers immediately following the NMC abbreviation indicate the relative stoichiometry of the three defining metals. For example, an NMC molar composition of 33% nickel, 33% manganese, and 33% cobalt would abbreviate to NMC111 (also NMC333 or NCM333) and have a chemical formula of LiNi 0.33Mn0.33Co 0.33O2. A composition of 50% nickel, 30% manganese, and 20% cobalt would be called NMC532 (or NCM523) and have the formula LiNi0.5Mn0.3Co0.2O2. Other common compositions are NMC622 and NMC811.[4] The general lithium content typically remains around 1:1 with the total transition metal content, with commercial NMC samples usually containing less than 5% excess lithium.[6][7]
For NMC111, the ideal oxidation states for charge distribution are Mn4+, Co3+, and Ni2+. Cobalt and nickel oxidize partially to Co4+ and Ni4+ during charging, while Mn4+ remains inactive and maintains structural stability.[8] Modifying the transition metal stoichiometry changes the material's properties, providing a way to adjust cathode performance.[3] Most notably, increasing the nickel content in NMC increases its initial discharge capacity, but lowers its thermal stability and capacity retention. Increasing cobalt content comes at the cost of replacing either higher-energy nickel or chemically stable manganese while also being expensive. Oxygen can generate from the metal oxide at 300 °C when fully discharged, degrading the lattice. Higher nickel content decreases the oxygen generation temperature while also increasing the heat generation during battery operation.[3] Cation mixing, a process in which Li+ substitutes Ni2+ ions in the lattice, increases as nickel concentration increases as well.[9] The similar size of Ni2+ (0.69 Å) and Li+ (0.76 Å) facilitates cation mixing. Displacing nickel from the layered structure can alter the material's bonding characteristics, forming undesirable phases and lowering its capacity.[10][11]
Synthesis
The crystallinity, particle size distribution, morphology, and composition all affect the performance of NMC materials, and these parameters can be tuned by using different synthesis methods.[4][12] The first report of nickel manganese cobalt oxide used a coprecipitation method,[13] which is still commonly used today.[14] This method involves dissolving the desired amount of metal precursors together and then drying them to remove the solvent. This material is then blended with a lithium source and heated to temperatures up to 900 °C under oxygen in a process called calcination. Hydroxides, oxalic acid, and carbonates are the most common coprecipitation agents.[14]
Sol-gel methods are another common NMC synthesis method. In this method, transition metal precursors are dissolved in a nitrate or acetate solution, then combined with a lithium nitrate or lithium acetate and citric acid solution. This mixture is stirred and heated to about 80 °C under basic conditions until a viscous gel forms. The gel is dried at around 120 °C and calcined twice, once at 450 °C and again at 800-900 °C, to obtain NMC material.[12]
Hydrothermal treatment can be paired with either the coprecipitation or sol-gel routes. It involves heating the coprecipitate or gel precursors in an autoclave. The treated precursors are then filtered off and calcined normally. Hydrothermal treatments before calcination improves the crystallinity of NMC, which increases the material's performance in cells. However, this comes at the cost of longer material processing times.[12]
History
NMC cathode materials are historically derived from John B. Goodenough's 1980s work on lithium cobalt oxide (LiCoO2).[15] The invention(s) of Li-NCM cathode material(s) occurred ca. 2000-2001 almost simultaneously and independently in four research teams:
1) at Argonne National Laboratory in the USA led by Thackeray;[16][17]
2) at Pacific Lithium in New Zealand led by Brett Amundsen;
3) at Dalhousie University in Canada led by Jeff Dahn;[18]
4) at Osaka City University led by Tsutomu Ohzuku.[19] The latter group also developed lithium nickel cobalt aluminium oxides.
Properties
The cell voltage of lithium ion batteries with NMC cathodes is 3.6–3.7 V.[20]
Arumugam Manthiram has reported that the relative positioning of the metals' 3d bands to the oxygen 2p band leads to each metal's role within NMC cathode materials. The manganese 3d band is above the oxygen 2p band, resulting in manganese's high chemical stability. The cobalt and nickel 3d bands overlap the oxygen 2p band, allowing them to charge to their 4+ oxidation states without the oxygen ions losing electron density.[21]
Usage
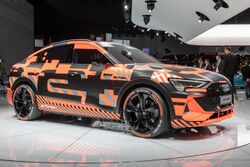
Many electric cars use NMC cathode batteries. NMC batteries were installed in the BMW ActiveE in 2011, and in the BMW i8 starting from 2013.[22] Other electric cars with NMC batteries include, as of 2020: Audi e-tron GE, BAIC EU5 R550, BMW i3, BYD Yuan EV535, Chevrolet Bolt, Hyundai Kona Electric, Jaguar I-Pace, Jiangling Motors JMC E200L, NIO ES6, Nissan Leaf S Plus, Renault ZOE, Roewe Ei5, VW e-Golf and VW ID.3.[23] Only a few electric car manufacturers do not use NMC cathodes in their traction batteries. Tesla is a significant exception, as they use nickel cobalt aluminium oxide and lithium iron phosphate batteries for their vehicles. In 2015, Elon Musk reported that the home storage Tesla Powerwall is based on NMC in order to increase the number of charge/discharge cycles over the life of the units.[23]
Mobile electronics such as mobile phones/smartphones, laptops, and pedelecs can also use NMC-based batteries.[24] These applications almost exclusively used lithium cobalt oxide batteries previously.[25] Another application of NMC batteries is battery storage power stations. Two such storage systems were installed in Korea in 2016 with a combined capacity of 15 MWh.[26] In 2017, a 35 MW NMC battery with a capacity of 11 MWh was installed and commissioned in Newman in the Australian state of Western Australia.[27][28]
See also
References
- ↑ Warner, John T. (2019-01-01), Warner, John T., ed., "Chapter 8 - The materials" (in en), Lithium-Ion Battery Chemistries (Elsevier): pp. 171–217, doi:10.1016/b978-0-12-814778-8.00008-9, ISBN 978-0-12-814778-8, https://www.sciencedirect.com/science/article/pii/B9780128147788000089, retrieved 2023-04-02
- ↑ Oswald, Stefan; Gasteiger, Hubert A. (2023-03-01). "The Structural Stability Limit of Layered Lithium Transition Metal Oxides Due to Oxygen Release at High State of Charge and Its Dependence on the Nickel Content". Journal of the Electrochemical Society 170 (3): 030506. doi:10.1149/1945-7111/acbf80. ISSN 0013-4651. Bibcode: 2023JElS..170c0506O. https://iopscience.iop.org/article/10.1149/1945-7111/acbf80.
- ↑ 3.0 3.1 3.2 Manthiram, Arumugam; Knight, James C.; Myung, Seung-Taek; Oh, Seung-Min; Sun, Yang-Kook (2015-10-07). "Nickel-Rich and Lithium-Rich Layered Oxide Cathodes: Progress and Perspectives" (in en). Advanced Energy Materials 6 (1): 1501010. doi:10.1002/aenm.201501010. https://onlinelibrary.wiley.com/doi/10.1002/aenm.201501010.
- ↑ 4.0 4.1 4.2 Warner, John T. (2019-01-01), Warner, John T., ed., "Chapter 5 - The Cathodes" (in en), Lithium-Ion Battery Chemistries (Elsevier): pp. 99–114, doi:10.1016/b978-0-12-814778-8.00005-3, ISBN 978-0-12-814778-8, https://www.sciencedirect.com/science/article/pii/B9780128147788000053, retrieved 2023-04-02
- ↑ Houchins, Gregory; Viswanathan, Venkatasubramanian (2020-01-01). "Towards Ultra Low Cobalt Cathodes: A High Fidelity Computational Phase Search of Layered Li-Ni-Mn-Co Oxides". Journal of the Electrochemical Society 167 (7): 070506. doi:10.1149/2.0062007JES. ISSN 0013-4651. Bibcode: 2020JElS..167g0506H. https://iopscience.iop.org/article/10.1149/2.0062007JES.
- ↑ Julien, Christian; Mauger, Alain; Zaghib, Karim; Groult, Henri (2016-07-19). "Optimization of Layered Cathode Materials for Lithium-Ion Batteries" (in en). Materials 9 (7): 595. doi:10.3390/ma9070595. ISSN 1996-1944. PMID 28773717. Bibcode: 2016Mate....9..595J.
- ↑ Li, Xuemin; Colclasure, Andrew M.; Finegan, Donal P.; Ren, Dongsheng; Shi, Ying; Feng, Xuning; Cao, Lei; Yang, Yuan et al. (2019-02-20). "Degradation mechanisms of high capacity 18650 cells containing Si-graphite anode and nickel-rich NMC cathode" (in en). Electrochimica Acta 297: 1109–1120. doi:10.1016/j.electacta.2018.11.194. https://linkinghub.elsevier.com/retrieve/pii/S0013468618326781.
- ↑ Yoon, Won-Sub; Grey, Clare P.; Balasubramanian, Mahalingam; Yang, Xiao-Qing; Fischer, Daniel A.; McBreen, James (2004). "Combined NMR and XAS Study on Local Environments and Electronic Structures of Electrochemically Li-Ion Deintercalated Li[sub 1−xCo[sub 1/3]Ni[sub 1/3]Mn[sub 1/3]O[sub 2] Electrode System"] (in en). Electrochemical and Solid-State Letters 7 (3): A53. doi:10.1149/1.1643592. https://iopscience.iop.org/article/10.1149/1.1643592.
- ↑ Zhang, Xiaoyu; Jiang, W. J.; Mauger, A.; Qilu; Gendron, F.; Julien, C. M. (2010-03-01). "Minimization of the cation mixing in Li1+x(NMC)1−xO2 as cathode material" (in en). Journal of Power Sources 195 (5): 1292–1301. doi:10.1016/j.jpowsour.2009.09.029. ISSN 0378-7753. Bibcode: 2010JPS...195.1292Z. https://www.sciencedirect.com/science/article/pii/S0378775309016231.
- ↑ Xu, Bo; Fell, Christopher R.; Chi, Miaofang; Meng, Ying Shirley (2011). "Identifying surface structural changes in layered Li-excess nickel manganese oxides in high voltage lithium ion batteries: A joint experimental and theoretical study" (in en). Energy & Environmental Science 4 (6): 2223. doi:10.1039/c1ee01131f. ISSN 1754-5692. http://xlink.rsc.org/?DOI=c1ee01131f.
- ↑ Zhao, Enyue; Fang, Lincan; Chen, Minmin; Chen, Dongfeng; Huang, Qingzhen; Hu, Zhongbo; Yan, Qing-bo; Wu, Meimei et al. (2017-01-24). "New insight into Li/Ni disorder in layered cathode materials for lithium ion batteries: a joint study of neutron diffraction, electrochemical kinetic analysis and first-principles calculations" (in en). Journal of Materials Chemistry A 5 (4): 1679–1686. doi:10.1039/C6TA08448F. ISSN 2050-7496. https://pubs.rsc.org/en/content/articlelanding/2017/ta/c6ta08448f.
- ↑ 12.0 12.1 12.2 Malik, Monu; Chan, Ka Ho; Azimi, Gisele (2022-08-01). "Review on the synthesis of LiNixMnyCo1-x-yO2 (NMC) cathodes for lithium-ion batteries" (in en). Materials Today Energy 28: 101066. doi:10.1016/j.mtener.2022.101066. ISSN 2468-6069. https://www.sciencedirect.com/science/article/pii/S2468606922001241.
- ↑ Liu, Zhaolin; Yu, Aishui; Lee, Jim Y (1999-09-01). "Synthesis and characterization of LiNi1−x−yCoxMnyO2 as the cathode materials of secondary lithium batteries" (in en). Journal of Power Sources 81-82: 416–419. doi:10.1016/S0378-7753(99)00221-9. ISSN 0378-7753. Bibcode: 1999JPS....81..416L. https://www.sciencedirect.com/science/article/pii/S0378775399002219.
- ↑ 14.0 14.1 Dong, Hongxu; Koenig, Gary M. (2020). "A review on synthesis and engineering of crystal precursors produced via coprecipitation for multicomponent lithium-ion battery cathode materials" (in en). CrystEngComm 22 (9): 1514–1530. doi:10.1039/C9CE00679F. ISSN 1466-8033. http://xlink.rsc.org/?DOI=C9CE00679F.
- ↑ Mizushima, K.; Jones, P. C.; Wiseman, P. J.; Goodenough, J. B. (1980-06-01). "LixCoO2 (0" (in en). Materials Research Bulletin 15 (6): 783–789. doi:10.1016/0025-5408(80)90012-4. ISSN 0025-5408. https://dx.doi.org/10.1016/0025-5408%2880%2990012-4.
- ↑ , Michael M.; Christopher S. Johnson & Khalil Amine et al."Lithium metal oxide electrodes for lithium cells and batteries" patent US6677082B2, issued 2004-01-13
- ↑ , Michael M.; Christopher S. Johnson & Khalil Amine et al."Lithium metal oxide electrodes for lithium cells and batteries" patent US6680143B2, issued 2004-01-20
- ↑ , Zhonghua & Jeffrey R. Dahn"Cathode compositions for lithium-ion batteries" patent US6964828B2, issued 2005-11-15
- ↑ Makimura, Yoshinari; Ohzuku, Tsutomu (2003-06-01). "Lithium insertion material of LiNi1/2Mn1/2O2 for advanced lithium-ion batteries" (in en). Journal of Power Sources. Selected papers presented at the 11th International Meeting on Lithium Batteries 119-121: 156–160. doi:10.1016/S0378-7753(03)00170-8. ISSN 0378-7753. Bibcode: 2003JPS...119..156M. https://www.sciencedirect.com/science/article/pii/S0378775303001708.
- ↑ Miller, Peter (2015-01-01). "Automotive Lithium-Ion Batteries". Johnson Matthey Technology Review 59 (1): 4–13. doi:10.1595/205651315X685445.
- ↑ Manthiram, Arumugam (2020-03-25). "A reflection on lithium-ion battery cathode chemistry" (in en). Nature Communications 11 (1): 1550. doi:10.1038/s41467-020-15355-0. ISSN 2041-1723. PMID 32214093. Bibcode: 2020NatCo..11.1550M.
- ↑ Sakti, Apurba; Michalek, Jeremy J.; Fuchs, Erica R. H.; Whitacre, Jay F. (2015-01-01). "A techno-economic analysis and optimization of Li-ion batteries for light-duty passenger vehicle electrification" (in en). Journal of Power Sources 273: 966–980. doi:10.1016/j.jpowsour.2014.09.078. ISSN 0378-7753. Bibcode: 2015JPS...273..966S. https://www.sciencedirect.com/science/article/pii/S0378775314014888.
- ↑ 23.0 23.1 Li, Wangda; Erickson, Evan M.; Manthiram, Arumugam (2020-01-13). "High-nickel layered oxide cathodes for lithium-based automotive batteries" (in en). Nature Energy 5 (1): 26–34. doi:10.1038/s41560-019-0513-0. ISSN 2058-7546. Bibcode: 2020NatEn...5...26L. https://www.nature.com/articles/s41560-019-0513-0.
- ↑ Li-battery safety. Jürgen Garche, Klaus Brandt. Amsterdam, Netherlands. 2019. ISBN 978-0-444-64008-6. OCLC 1054022372. https://www.worldcat.org/oclc/1054022372.
- ↑ Patoux, Sébastien; Sannier, Lucas; Lignier, Hélène; Reynier, Yvan; Bourbon, Carole; Jouanneau, Séverine; Le Cras, Frédéric; Martinet, Sébastien (2008-05-01). "High voltage nickel manganese spinel oxides for Li-ion batteries" (in en). Electrochimica Acta 53 (12): 4137–4145. doi:10.1016/j.electacta.2007.12.054. ISSN 0013-4686. https://www.sciencedirect.com/science/article/pii/S0013468607015046.
- ↑ Kokam (March 7, 2016). "Kokam's 56 Megawatt Energy Storage Project Features World's Largest Lithium NMC Energy Storage System for Frequency Regulation". PR Newswire.. https://www.prnewswire.com/news-releases/kokams-56-megawatt-energy-storage-project-features-worlds-largest-lithium-nmc-energy-storage-system-for-frequency-regulation-300229219.html.
- ↑ Giles Parkinson (2019-08-12). "Alinta sees sub 5-year payback for unsubsidised big battery at Newman" (in en-AU). https://reneweconomy.com.au/alinta-sees-sub-5-year-payback-for-unsubsidised-big-battery-at-newman-78605/.
- ↑ "Energy Storage Solution Provider" (in en). https://kokam.com/data/Kokam_ESS_Brochure_200131.pdf.
![]() | Original source: https://en.wikipedia.org/wiki/Lithium nickel manganese cobalt oxides.
Read more |