Biology:Telomerase
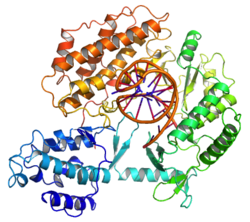
Telomerase, also called terminal transferase,[1] is a ribonucleoprotein that adds a species-dependent telomere repeat sequence to the 3' end of telomeres. A telomere is a region of repetitive sequences at each end of the chromosomes of most eukaryotes. Telomeres protect the end of the chromosome from DNA damage or from fusion with neighbouring chromosomes. The fruit fly Drosophila melanogaster lacks telomerase, but instead uses retrotransposons to maintain telomeres.[2]
Telomerase is a reverse transcriptase enzyme that carries its own RNA molecule (e.g., with the sequence 3′-CCCAAUCCC-5′ in Trypanosoma brucei)[3] which is used as a template when it elongates telomeres. Telomerase is active in gametes and most cancer cells, but is normally absent in most somatic cells.
History
The existence of a compensatory mechanism for telomere shortening was first found by Soviet biologist Alexey Olovnikov in 1973,[4] who also suggested the telomere hypothesis of aging and the telomere's connections to cancer and perhaps some neurodegenerative diseases.[5]
Telomerase in the ciliate Tetrahymena was discovered by Carol W. Greider and Elizabeth Blackburn in 1984.[6] Together with Jack W. Szostak, Greider and Blackburn were awarded the 2009 Nobel Prize in Physiology or Medicine for their discovery.[7] Later the cryo-EM structure of telomerase was first reported in T. thermophila, to be followed a few years later by the cryo-EM structure of telomerase in humans.[8]
The role of telomeres and telomerase in cell aging and cancer was established by scientists at biotechnology company Geron with the cloning of the RNA and catalytic components of human telomerase[9] and the development of a polymerase chain reaction (PCR) based assay for telomerase activity called the TRAP assay, which surveys telomerase activity in multiple types of cancer.[10]
The negative stain electron microscopy (EM) structures of human and Tetrahymena telomerases were characterized in 2013.[11][12] Two years later, the first cryo-electron microscopy (cryo-EM) structure of telomerase holoenzyme (Tetrahymena) was determined.[13] In 2018, the structure of human telomerase was determined through cryo-EM by UC Berkeley scientists.[14]
Human telomerase structure
The molecular composition of the human telomerase complex was determined by Scott Cohen and his team at the Children's Medical Research Institute (Sydney Australia) and consists of two molecules each of human telomerase reverse transcriptase (TERT), Telomerase RNA Component (TR or TERC), and dyskerin (DKC1).[15] The genes of telomerase subunits, which include TERT,[16] TERC,[17] DKC1[18] and TEP1,[19] are located on different chromosomes. The human TERT gene (hTERT) is translated into a protein of 1132 amino acids.[20] TERT polypeptide folds with (and carries) TERC, a non-coding RNA (451 nucleotides long). TERT has a 'mitten' structure that allows it to wrap around the chromosome to add single-stranded telomere repeats.
TERT is a reverse transcriptase, which is a class of enzymes that creates single-stranded DNA using single-stranded RNA as a template.
The protein consists of four conserved domains (RNA-Binding Domain (TRBD), fingers, palm and thumb), organized into a "right hand" ring configuration that shares common features with retroviral reverse transcriptases, viral RNA replicases and bacteriophage B-family DNA polymerases.[21][22]
TERT proteins from many eukaryotes have been sequenced.[23]
Mechanism
The shelterin protein TPP1 is both necessary and sufficient to recruit the telomerase enzyme to telomeres, and is the only shelterin protein in direct contact with telomerase.[24]
By using TERC, TERT can add a six-nucleotide repeating sequence, 5'-TTAGGG (in vertebrates; the sequence differs in other organisms) to the 3' strand of chromosomes. These TTAGGG repeats (with their various protein binding partners) are called telomeres. The template region of TERC is 3'-CAAUCCCAAUC-5'.[25]
Telomerase can bind the first few nucleotides of the template to the last telomere sequence on the chromosome, add a new telomere repeat (5'-GGTTAG-3') sequence, let go, realign the new 3'-end of telomere to the template, and repeat the process. Telomerase reverses telomere shortening.
Clinical implications
Aging
Telomerase restores short bits of DNA known as telomeres, which are otherwise shortened after repeated division of a cell via mitosis.
In normal circumstances, where telomerase is absent, if a cell divides recursively, at some point the progeny reach their Hayflick limit,[26] which is believed to be between 50 and 70 cell divisions. At the limit the cells become senescent and cell division stops.[27] Telomerase allows each offspring to replace the lost bit of DNA, allowing the cell line to divide without ever reaching the limit. This same unbounded growth is a feature of cancerous growth.[28]
Embryonic stem cells express telomerase, which allows them to divide repeatedly and form the individual. In adults, telomerase is highly expressed only in cells that need to divide regularly, especially in male sperm cells,[29] but also in epidermal cells,[30] in activated T cell[31] and B cell[32] lymphocytes, as well as in certain adult stem cells, but in the great majority of cases somatic cells do not express telomerase.[33]
A comparative biology study of mammalian telomeres indicated that telomere length of some mammalian species correlates inversely, rather than directly, with lifespan, and concluded that the contribution of telomere length to lifespan is unresolved.[34] Telomere shortening does not occur with age in some postmitotic tissues, such as in the rat brain.[35] In humans, skeletal muscle telomere lengths remain stable from ages 23 –74.[36] In baboon skeletal muscle, which consists of fully differentiated postmitotic cells, less than 3% of myonuclei contain damaged telomeres and this percentage does not increase with age.[37] Thus, telomere shortening does not appear to be a major factor in the aging of the differentiated cells of brain or skeletal muscle. In human liver, cholangiocytes and hepatocytes show no age-related telomere shortening.[38] Another study found little evidence that, in humans, telomere length is a significant biomarker of normal aging with respect to important cognitive and physical abilities.[39]
Some experiments have raised questions on whether telomerase can be used as an anti-aging therapy, namely, the fact that mice with elevated levels of telomerase have higher cancer incidence and hence do not live longer.[40] On the other hand, one study showed that activating telomerase in cancer-resistant mice by overexpressing its catalytic subunit extended lifespan.[41] A study found that long-lived subjects inherited a hyperactive version of telomerase.[42]
Premature aging
Premature aging syndromes including Werner syndrome, Progeria, Ataxia telangiectasia, Ataxia-telangiectasia like disorder, Bloom syndrome, Fanconi anemia and Nijmegen breakage syndrome are associated with short telomeres.[43] However, the genes that have mutated in these diseases all have roles in the repair of DNA damage and the increased DNA damage may, itself, be a factor in the premature aging (see DNA damage theory of aging). An additional role in maintaining telomere length is an active area of investigation.
Cancer
In vitro, when cells approach the Hayflick limit, the time to senescence can be extended by inactivating the tumor suppressor proteins p53 and Retinoblastoma protein (pRb).[44] Cells that have been so-altered eventually undergo an event termed a "crisis" when the majority of the cells in the culture die. Sometimes, a cell does not stop dividing once it reaches a crisis. In a typical situation, the telomeres are shortened[45] and chromosomal integrity declines with every subsequent cell division. Exposed chromosome ends are interpreted as double-stranded breaks (DSB) in DNA; such damage is usually repaired by reattaching the broken ends together. When the cell does this due to telomere-shortening, the ends of different chromosomes can be attached to each other. This solves the problem of lacking telomeres, but during cell division anaphase, the fused chromosomes are randomly ripped apart, causing many mutations and chromosomal abnormalities. As this process continues, the cell's genome becomes unstable. Eventually, either fatal damage is done to the cell's chromosomes (killing it via apoptosis), or an additional mutation that activates telomerase occurs.[44]
With telomerase activation some types of cells and their offspring become immortal (bypass the Hayflick limit), thus avoiding cell death as long as the conditions for their duplication are met. Many cancer cells are considered 'immortal' because telomerase activity allows them to live much longer than any other somatic cell, which, combined with uncontrollable cell proliferation[46] is why they can form tumors. A good example of immortal cancer cells is HeLa cells, which have been used in laboratories as a model cell line since 1951.
While this method of modelling human cancer in cell culture is effective and has been used for many years by scientists, it is also very imprecise. The exact changes that allow for the formation of the tumorigenic clones in the above-described experiment are not clear. Scientists addressed this question by the serial introduction of multiple mutations present in a variety of human cancers. This has led to the identification of mutation combinations that form tumorigenic cells in a variety of cell types. While the combination varies by cell type, the following alterations are required in all cases: TERT activation, loss of p53 pathway function, loss of pRb pathway function, activation of the Ras or myc proto-oncogenes, and aberration of the PP2A protein phosphatase.[47] That is to say, the cell has an activated telomerase, eliminating the process of death by chromosome instability or loss, absence of apoptosis-induction pathways, and continued mitosis activation.
This model of cancer in cell culture accurately describes the role of telomerase in actual human tumors. Telomerase activation has been observed in ~90% of all human tumors,[48] suggesting that the immortality conferred by telomerase plays a key role in cancer development. Of the tumors without TERT activation,[49] most employ a separate pathway to maintain telomere length termed Alternative Lengthening of Telomeres (ALT).[50] The exact mechanism behind telomere maintenance in the ALT pathway is unclear, but likely involves multiple recombination events at the telomere.
Elizabeth Blackburn et al., identified the upregulation of 70 genes known or suspected in cancer growth and spread through the body, and the activation of glycolysis, which enables cancer cells to rapidly use sugar to facilitate their programmed growth rate (roughly the growth rate of a fetus).[51]
Approaches to controlling telomerase and telomeres for cancer therapy include gene therapy, immunotherapy, small-molecule and signal pathway inhibitors.[52]
Drugs
The ability to maintain functional telomeres may be one mechanism that allows cancer cells to grow in vitro for decades.[53] Telomerase activity is necessary to preserve many cancer types and is inactive in somatic cells, creating the possibility that telomerase inhibition could selectively repress cancer cell growth with minimal side effects.[54] If a drug can inhibit telomerase in cancer cells, the telomeres of successive generations will progressively shorten, limiting tumor growth.[55]
Telomerase is a good biomarker for cancer detection because most human cancer cells express high levels of it. Telomerase activity can be identified by its catalytic protein domain (hTERT). This[clarify] is the rate-limiting step in telomerase activity. It is associated with many cancer types. Various cancer cells and fibroblasts transformed with hTERT cDNA have high telomerase activity, while somatic cells do not. Cells testing positive for hTERT have positive nuclear signals. Epithelial stem cell tissue and its early daughter cells are the only noncancerous cells in which hTERT can be detected. Since hTERT expression is dependent only on the number of tumor cells within a sample, the amount of hTERT indicates the severity of cancer.[56]
The expression of hTERT can also be used to distinguish benign tumors from malignant tumors. Malignant tumors have higher hTERT expression than benign tumors. Real-time reverse transcription polymerase chain reaction (RT-PCR) quantifying hTERT expression in various tumor samples verified this varying expression.[57]
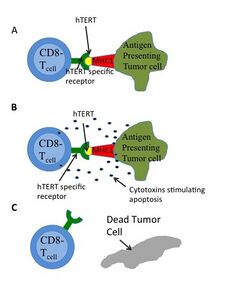
The lack of telomerase does not affect cell growth until the telomeres are short enough to cause cells to "die or undergo growth arrest". However, inhibiting telomerase alone is not enough to destroy large tumors. It must be combined with surgery, radiation, chemotherapy or immunotherapy.[56]
Cells may reduce their telomere length by only 50-252 base pairs per cell division, which can lead to a long lag phase.[58][59]
A telomerase activator TA-65 is commercially available and is claimed to delay aging and to provide relief from certain disease conditions.[60][61][62][63][64] This formulation contains a molecule called cycloastragenol derived from a legume Astragalus membranaceus.
Immunotherapy
Immunotherapy successfully treats some kinds of cancer, such as melanoma. This treatment involves manipulating a human's immune system to destroy cancerous cells. Humans have two major antigen identifying lymphocytes: CD8+ cytotoxic T-lymphocytes (CTL) and CD4+ helper T-lymphocytes that can destroy cells. Antigen receptors on CTL can bind to a 9-10 amino acid chain that is presented by the major histocompatibility complex (MHC) as in Figure 4. HTERT is a potential target antigen. Immunotargeting should result in relatively few side effects since hTERT expression is associated only with telomerase and is not essential in almost all somatic cells.[65] GV1001 uses this pathway.[52] Experimental drug and vaccine therapies targeting active telomerase have been tested in mouse models, and clinical trials have begun. One drug, imetelstat, is being clinically researched as a means of interfering with telomerase in cancer cells.[66] Most of the harmful cancer-related effects of telomerase are dependent on an intact RNA template. Cancer stem cells that use an alternative method of telomere maintenance are still killed when telomerase's RNA template is blocked or damaged.
Telomerase Vaccines
Two telomerase vaccines have been developed: GRNVAC1 and GV1001. GRNVAC1 isolates dendritic cells and the RNA that codes for the telomerase protein and puts them back into the patient to make cytotoxic T cells that kill the telomerase-active cells. GV1001 is a peptide from the active site of hTERT and is recognized by the immune system that reacts by killing the telomerase-active cells.[52]
Targeted apoptosis
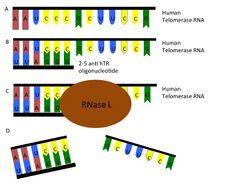
Another independent approach is to use oligoadenylated anti-telomerase antisense oligonucleotides and ribozymes to target telomerase RNA, reducing dissociation and apoptosis (Figure 5). The fast induction of apoptosis through antisense binding may be a good alternative to the slower telomere shortening.[58]
Small interfering RNA (siRNA)
siRNAs are small RNA molecules that induce the sequence-specific degradation of other RNAs. siRNA treatment can function similar to traditional gene therapy by destroying the mRNA products of particular genes, and therefore preventing the expression of those genes. A 2012 study found that targeting TERC with an siRNA reduced telomerase activity by more than 50% and resulted in decreased viability of immortal cancer cells.[67] Treatment with both the siRNA and radiation caused a greater reduction in tumor size in mice than treatment with radiation alone, suggesting that targeting telomerase could be a way to increase the efficacy of radiation in treating radiation-resistant tumors.
Heart disease, diabetes and quality of life
Blackburn also discovered that mothers caring for very sick children have shorter telomeres when they report that their emotional stress is at a maximum and that telomerase was active at the site of blockages in coronary artery tissue, possibly accelerating heart attacks.
In 2009, it was shown that the amount of telomerase activity significantly increased following psychological stress. Across the sample of patients telomerase activity in peripheral blood mononuclear cells increased by 18% one hour after the end of the stress.[68]
A study in 2010 found that there was "significantly greater" telomerase activity in participants than controls after a three-month meditation retreat.[69]
Telomerase deficiency has been linked to diabetes mellitus and impaired insulin secretion in mice, due to loss of pancreatic insulin-producing cells.[70]
Rare human diseases
Mutations in TERT have been implicated in predisposing patients to aplastic anemia, a disorder in which the bone marrow fails to produce blood cells, in 2005.[71]
Cri du chat syndrome (CdCS) is a complex disorder involving the loss of the distal portion of the short arm of chromosome 5. TERT is located in the deleted region, and loss of one copy of TERT has been suggested as a cause or contributing factor of this disease.[72]
Dyskeratosis congenita (DC) is a disease of the bone marrow that can be caused by some mutations in the telomerase subunits.[73] In the DC cases, about 35% cases are X-linked-recessive on the DKC1 locus[74] and 5% cases are autosomal dominant on the TERT[75] and TERC[76] loci.
Patients with DC have severe bone marrow failure manifesting as abnormal skin pigmentation, leucoplakia (a white thickening of the oral mucosa) and nail dystrophy, as well as a variety of other symptoms. Individuals with either TERC or DKC1 mutations have shorter telomeres and defective telomerase activity in vitro versus other individuals of the same age.[77]
In one family autosomal dominant DC was linked to a heterozygous TERT mutation.[5] These patients also exhibited an increased rate of telomere-shortening, and genetic anticipation (i.e., the DC phenotype worsened with each generation).
TERT Splice Variants
See also
- DNA repair
- Imetelstat
- TA-65
- Telomere
- Epitalon
References
- ↑ What are telomeres and telomerase?
- ↑ "Retrotransposons that maintain chromosome ends". PNAS 108 (51): 20317–24. 2011. doi:10.1073/pnas.1100278108. PMID 21821789.
- ↑ "Telomerase in kinetoplastid parasitic protozoa". Proceedings of the National Academy of Sciences of the United States of America 96 (7): 3616–21. March 1999. doi:10.1073/pnas.96.7.3616. PMID 10097086. Bibcode: 1999PNAS...96.3616C.
- ↑ "A theory of marginotomy. The incomplete copying of template margin in enzymic synthesis of polynucleotides and biological significance of the phenomenon". Journal of Theoretical Biology 41 (1): 181–90. September 1973. doi:10.1016/0022-5193(73)90198-7. PMID 4754905. Bibcode: 1973JThBi..41..181O.
- ↑ 5.0 5.1 "Haploinsufficiency of telomerase reverse transcriptase leads to anticipation in autosomal dominant dyskeratosis congenita". Proceedings of the National Academy of Sciences of the United States of America 102 (44): 15960–4. November 2005. doi:10.1073/pnas.0508124102. PMID 16247010. Bibcode: 2005PNAS..10215960A.
- ↑ "Identification of a specific telomere terminal transferase activity in Tetrahymena extracts". Cell 43 (2 Pt 1): 405–13. December 1985. doi:10.1016/0092-8674(85)90170-9. PMID 3907856.
- ↑ "The Nobel Prize in Physiology or Medicine 2009". The Nobel Foundation. 2009-10-05. http://nobelprize.org/nobel_prizes/medicine/laureates/2009/press.html.
- ↑ "Structural Biology of Telomerase". Cold Spring Harbor Perspectives in Biology 11 (12): a032383. 2019. doi:10.1101/cshperspect.a032383. PMID 31451513.
- ↑ "The RNA component of human telomerase". Science 269 (5228): 1236–41. September 1995. doi:10.1126/science.7544491. PMID 7544491. Bibcode: 1995Sci...269.1236F.
- ↑ "Specific association of human telomerase activity with immortal cells and cancer". Science 266 (5193): 2011–5. December 1994. doi:10.1126/science.7605428. PMID 7605428. Bibcode: 1994Sci...266.2011K.
- ↑ "Structure of active dimeric human telomerase". Nature Structural & Molecular Biology 20 (4): 454–60. April 2013. doi:10.1038/nsmb.2530. PMID 23474713.
- ↑ "The architecture of Tetrahymena telomerase holoenzyme". Nature 496 (7444): 187–92. April 2013. doi:10.1038/nature12062. PMID 23552895. Bibcode: 2013Natur.496..187J.
- ↑ "Structure of Tetrahymena telomerase reveals previously unknown subunits, functions, and interactions". Science 350 (6260): aab4070. October 2015. doi:10.1126/science.aab4070. PMID 26472759.
- ↑ "Cryo-EM structure of substrate-bound human telomerase holoenzyme" (in En). Nature 557 (7704): 190–195. May 2018. doi:10.1038/s41586-018-0062-x. PMID 29695869. Bibcode: 2018Natur.557..190N.
- ↑ "Protein composition of catalytically active human telomerase from immortal cells". Science 315 (5820): 1850–3. 2007. doi:10.1126/science.1138596. PMID 17395830. Bibcode: 2007Sci...315.1850C.
- ↑ "HGNC database of human gene names - HUGO Gene Nomenclature Committee". genenames.org. https://www.genenames.org/index.html.
- ↑ HGNC - TERC
- ↑ HGNC - DKC1
- ↑ HGNC - TEP1
- ↑ NCBI - telomerase reverse transcriptase isoform 1
- ↑ Gillis AJ, Schuller AP, Skordalakes E. Structure of the Tribolium castaneum telomerase catalytic subunit TERT. Nature. 2008 Oct 2;455(7213):633-7
- ↑ Mitchell M, Gillis A, Futahashi M, Fujiwara H, Skordalakes E. Structural basis for telomerase catalytic subunit TERT binding to RNA template and telomeric DNA. Nat Struct Mol Biol. 2010 Apr;17(4):513-8
- ↑ NCBI - telomerase reverse transcriptase
- ↑ "Molecular mechanisms of telomere biology disorders". Journal of Biological Chemistry 296: 100064. 2021. doi:10.1074/jbc.REV120.014017. PMID 33482595.
- ↑ "Minimum length requirement of the alignment domain of human telomerase RNA to sustain catalytic activity in vitro". Nucleic Acids Res. 30 (20): 4470–80. October 2002. doi:10.1093/nar/gkf575. PMID 12384594.
- ↑ "The serial cultivation of human diploid cell strains". Exp Cell Res 25 (3): 585–621. 1961. doi:10.1016/0014-4827(61)90192-6. PMID 13905658.
- ↑ Siegel, L (2013). Are Telomeres the Key to Aging and Cancer? The University of Utah. Retrieved 30 September 2013
- ↑ "Hallmarks of cancer: the next generation". Cell 144 (5): 646–74. March 2011. doi:10.1016/j.cell.2011.02.013. PMID 21376230.
- ↑ "Telomere Dynamics Throughout Spermatogenesis". Genes 10 (7): 525. July 2019. doi:10.3390/genes10070525. PMID 31336906.
- ↑ "Telomerase activity in the regenerative basal layer of the epidermis inhuman skin and in immortal and carcinoma-derived skin keratinocytes". Proceedings of the National Academy of Sciences of the United States of America 93 (13): 6476–6481. June 1996. doi:10.1073/pnas.93.13.6476. PMID 8692840. Bibcode: 1996PNAS...93.6476H.
- ↑ "Telomerase and primary T cells: biology and immortalization for adoptive immunotherapy". Immunotherapy 3 (3): 407–421. March 2011. doi:10.2217/imt.10.107. PMID 21395382.
- ↑ "PAX5 activates the transcription of the human telomerase reverse transcriptase gene in B cells". The Journal of Pathology 220 (1): 87–96. January 2010. doi:10.1002/path.2620. PMID 19806612.
- ↑ "Human telomerase and its regulation". Microbiology and Molecular Biology Reviews 66 (3): 407–25, table of contents. September 2002. doi:10.1128/MMBR.66.3.407-425.2002. PMID 12208997.
- ↑ "Comparative biology of mammalian telomeres: hypotheses on ancestral states and the roles of telomeres in longevity determination". Aging Cell 10 (5): 761–8. October 2011. doi:10.1111/j.1474-9726.2011.00718.x. PMID 21518243.
- ↑ "Ageing and telomeres: a study into organ- and gender-specific telomere shortening". Nucleic Acids Research 31 (5): 1576–83. March 2003. doi:10.1093/nar/gkg208. PMID 12595567.
- ↑ "Regenerative potential of human skeletal muscle during aging". Aging Cell 1 (2): 132–9. December 2002. doi:10.1046/j.1474-9728.2002.00017.x. PMID 12882343.
- ↑ "Accumulation of senescent cells in mitotic tissue of aging primates". Mechanisms of Ageing and Development 128 (1): 36–44. January 2007. doi:10.1016/j.mad.2006.11.008. PMID 17116315.
- ↑ "Sustained telomere length in hepatocytes and cholangiocytes with increasing age in normal liver". Hepatology 56 (4): 1510–20. October 2012. doi:10.1002/hep.25787. PMID 22504828.
- ↑ "Telomere length and aging biomarkers in 70-year-olds: the Lothian Birth Cohort 1936". Neurobiology of Aging 33 (7): 1486.e3–8. July 2012. doi:10.1016/j.neurobiolaging.2010.11.013. PMID 21194798.
- ↑ "Telomeres and telomerase: a modern fountain of youth?". Rejuvenation Res 7 (2): 126–33. 2004. doi:10.1089/1549168041553044. PMID 15312299.
- ↑ "Telomerase reverse transcriptase delays aging in cancer-resistant mice". Cell 135 (4): 609–22. November 2008. doi:10.1016/j.cell.2008.09.034. PMID 19013273.
- ↑ "Genetic variation in human telomerase is associated with telomere length in Ashkenazi centenarians". Proc. Natl. Acad. Sci. U.S.A. 107 (Suppl 1): 1710–7. January 2010. doi:10.1073/pnas.0906191106. PMID 19915151.
- Lay summary in: "One Key Found for Living to 100". November 12, 2009. http://www.livescience.com/health/091112-anti-aging-telomeres.html.
- ↑ "Telomeres and human disease: ageing, cancer and beyond". Nature Reviews. Genetics 6 (8): 611–22. August 2005. doi:10.1038/nrg1656. PMID 16136653.
- ↑ 44.0 44.1 "Reactivation of telomerase in cancer". Cellular and Molecular Life Sciences 73 (8): 1659–1670. April 2016. doi:10.1007/s00018-016-2146-9. PMID 26846696.
- ↑ The Immortal Life of Henrietta Lacks. New York: Broadway Paperbacks. 2010. pp. 216, 217. ISBN 978-1-4000-5218-9. https://archive.org/details/unset0000unse_h0q7/page/216.
- ↑ Dr. Todd Hennessey, 2016 University at Buffalo
- ↑ "Deregulation of the protein phosphatase 2A, PP2A in cancer: complexity and therapeutic options". Tumour Biology 37 (9): 11691–11700. September 2016. doi:10.1007/s13277-016-5145-4. PMID 27444275.
- ↑ "A survey of telomerase activity in human cancer". European Journal of Cancer 33 (5): 787–91. April 1997. doi:10.1016/S0959-8049(97)00062-2. PMID 9282118.
- ↑ "Telomere elongation in immortal human cells without detectable telomerase activity". The EMBO Journal 14 (17): 4240–8. September 1995. doi:10.1002/j.1460-2075.1995.tb00098.x. PMID 7556065.
- ↑ "Alternative lengthening of telomeres in mammalian cells". Oncogene 21 (4): 598–610. January 2002. doi:10.1038/sj.onc.1205058. PMID 11850785.
- ↑ "Telomeres and telomerase: their mechanisms of action and the effects of altering their functions". FEBS Letters 579 (4): 859–862. February 2005. doi:10.1016/j.febslet.2004.11.036. PMID 15680963.
- ↑ 52.0 52.1 52.2 "Telomere and telomerase as targets for cancer therapy". Applied Biochemistry and Biotechnology 160 (5): 1460–72. March 2010. doi:10.1007/s12010-009-8633-9. PMID 19412578.
- ↑ Introduction to Genetic Analysis. W. H. Freeman. 2008. ISBN 978-0-7167-6887-6. https://books.google.com/books?id=MsFkrBY2-5AC.
- ↑ "No end in sight for telomerase-targeted cancer drugs". Nature Medicine 19 (1): 6. January 2013. doi:10.1038/nm0113-6. PMID 23295993.
- ↑ "Telomeres in Cancer Therapy". Journal of Biomedicine & Biotechnology 1 (1): 3–4. 2001. doi:10.1155/S1110724301000109. PMID 12488618.
- ↑ 56.0 56.1 "Telomerase and cancer". Human Molecular Genetics 10 (7): 677–85. April 2001. doi:10.1093/hmg/10.7.677. PMID 11257099.
- ↑ "The status of telomerase enzyme activity in benign and malignant gynaecologic pathologies". Balkan Medical Journal 30 (3): 287–92. September 2013. doi:10.5152/balkanmedj.2013.7328. PMID 25207121.
- ↑ 58.0 58.1 "Telomerase inhibition as cancer therapy". Cancer Letters 194 (2): 209–19. May 2003. doi:10.1016/s0304-3835(02)00708-5. PMID 12757979.
- ↑ "T-loop deletion factor showing speeding aging of Homo telomere diversity and evolution". Rejuvenation Research 12 (1): 52. 2009.
- ↑ "TA-65, A Telomerase Activator improves Cardiovascular Markers in Patients with Metabolic Syndrome". Current Pharmaceutical Design 24 (17): 1905–1911. 2018. doi:10.2174/1381612824666180316114832. PMID 29546832.
- ↑ "A natural product telomerase activator as part of a health maintenance program". Rejuvenation Research 14 (1): 45–56. February 2011. doi:10.1089/rej.2010.1085. PMID 20822369.
- ↑ "A Natural Product Telomerase Activator Lengthens Telomeres in Humans: A Randomized, Double Blind, and Placebo Controlled Study". Rejuvenation Research 19 (6): 478–484. December 2016. doi:10.1089/rej.2015.1793. PMID 26950204.
- ↑ "A natural product telomerase activator as part of a health maintenance program: metabolic and cardiovascular response". Rejuvenation Research 16 (5): 386–395. October 2013. doi:10.1089/rej.2013.1430. PMID 23808324.
- ↑ "Telomerase as a Therapeutic Target in Cardiovascular Disease". Arteriosclerosis, Thrombosis, and Vascular Biology 41 (3): 1047–1061. March 2021. doi:10.1161/ATVBAHA.120.315695. PMID 33504179.
- ↑ "Telomerase as a tumor-associated antigen for cancer immunotherapy". Cytotechnology 45 (1–2): 91–9. June 2004. doi:10.1007/s10616-004-5132-2. PMID 19003246.
- ↑ "Experimental blood disorder therapy shows promise in new studies". Modern Healthcare. 2 September 2015. http://www.modernhealthcare.com/article/20150902/NEWS/150909967.
- ↑ "siRNA-mediated inhibition of hTERC enhances radiosensitivity of cervical cancer". Asian Pacific Journal of Cancer Prevention 13 (12): 5975–9. 2012. doi:10.7314/apjcp.2012.13.12.5975. PMID 23464388.
- ↑ "Dynamics of telomerase activity in response to acute psychological stress". Brain, Behavior, and Immunity 24 (4): 531–9. May 2010. doi:10.1016/j.bbi.2009.11.018. PMID 20018236.
- ↑ "Intensive meditation training, immune cell telomerase activity, and psychological mediators". Psychoneuroendocrinology 36 (5): 664–81. June 2011. doi:10.1016/j.psyneuen.2010.09.010. PMID 21035949.
- ↑ "Telomerase deficiency impairs glucose metabolism and insulin secretion". Aging 2 (10): 650–8. October 2010. doi:10.18632/aging.100200. PMID 20876939. PMC 2993795. https://www.aging-us.com/article/100200/text.
- ↑ "Mutations in TERT, the gene for telomerase reverse transcriptase, in aplastic anemia". The New England Journal of Medicine 352 (14): 1413–24. April 2005. doi:10.1056/NEJMoa042980. PMID 15814878.
- ↑ "Deletion of the telomerase reverse transcriptase gene and haploinsufficiency of telomere maintenance in Cri du chat syndrome". American Journal of Human Genetics 72 (4): 940–8. April 2003. doi:10.1086/374565. PMID 12629597.
- ↑ "Mutations of telomerase complex genes linked to bone marrow failures". Journal of Nippon Medical School 74 (3): 202–9. June 2007. doi:10.1272/jnms.74.202. PMID 17625368.
- ↑ "X-linked dyskeratosis congenita is caused by mutations in a highly conserved gene with putative nucleolar functions". Nature Genetics 19 (1): 32–8. May 1998. doi:10.1038/ng0598-32. PMID 9590285.
- ↑ "Mutations in the reverse transcriptase component of telomerase (TERT) in patients with bone marrow failure". Blood Cells, Molecules & Diseases 34 (3): 257–63. 2005. doi:10.1016/j.bcmd.2004.12.008. PMID 15885610.
- ↑ "The RNA component of telomerase is mutated in autosomal dominant dyskeratosis congenita". Nature 413 (6854): 432–5. September 2001. doi:10.1038/35096585. PMID 11574891. Bibcode: 2001Natur.413..432V.
- ↑ "Dyskeratosis congenita: telomerase, telomeres and anticipation". Current Opinion in Genetics & Development 15 (3): 249–57. June 2005. doi:10.1016/j.gde.2005.04.004. PMID 15917199.
Further reading
- The Immortal Cell, by Michael D. West, Doubleday (2003) ISBN:978-0-385-50928-2
External links
- Gene Ontology: GO:0003720: telomerase activity
- Human telomerase reverse transcriptase (TERT) gene on genecards.org
- The Telomerase Database - A Web-based tool for telomerase research
- Three-dimensional model of telomerase at MUN
- Elizabeth Blackburn's Seminars: Telomeres and Telomerase
- Telomerase at the US National Library of Medicine Medical Subject Headings (MeSH)
- Overview of all the structural information available in the PDB for UniProt: O14746 (Human Telomerase reverse transcriptase) at the PDBe-KB.
- Overview of all the structural information available in the PDB for UniProt: Q0QHL8 (Tribolium castaneum Telomerase reverse transcriptase) at the PDBe-KB.
![]() | Original source: https://en.wikipedia.org/wiki/Telomerase.
Read more |