Biology:Dinoflagellate
Dinoflagellate | |
---|---|
![]() | |
Ceratium sp. | |
Scientific classification ![]() | |
Domain: | Eukaryota |
Clade: | Diaphoretickes |
Clade: | SAR |
Clade: | Alveolata |
Phylum: | Myzozoa |
Subphylum: | Dinozoa |
Superclass: | Dinoflagellata Bütschli 1885 [1880–1889] sensu Gomez 2012[2][3][4] |
Classes | |
| |
Synonyms | |
|
The dinoflagellates (Greek δῖνος dinos "whirling" and Latin flagellum "whip, scourge") are a monophyletic group of single-celled eukaryotes constituting the phylum Dinoflagellata[5] and are usually considered protists. Dinoflagellates are mostly marine plankton, but they also are common in freshwater habitats. Their populations vary with sea surface temperature, salinity, and depth. Many dinoflagellates are photosynthetic, but a large fraction of these are in fact mixotrophic, combining photosynthesis with ingestion of prey (phagotrophy and myzocytosis).[6][7]
In terms of number of species, dinoflagellates are one of the largest groups of marine eukaryotes, although substantially smaller than diatoms.[8] Some species are endosymbionts of marine animals and play an important part in the biology of coral reefs. Other dinoflagellates are unpigmented predators on other protozoa, and a few forms are parasitic (for example, Oodinium and Pfiesteria). Some dinoflagellates produce resting stages, called dinoflagellate cysts or dinocysts, as part of their lifecycles; this occurs in 84 of the 350 described freshwater species and a little more than 10% of the known marine species.[9][10] Dinoflagellates are alveolates possessing two flagella, the ancestral condition of bikonts.
About 1,555 species of free-living marine dinoflagellates are currently described.[11] Another estimate suggests about 2,000 living species, of which more than 1,700 are marine (free-living, as well as benthic) and about 220 are from fresh water.[12] The latest estimates suggest a total of 2,294 living dinoflagellate species, which includes marine, freshwater, and parasitic dinoflagellates.[2]
A rapid accumulation of certain dinoflagellates can result in a visible coloration of the water, colloquially known as red tide (a harmful algal bloom), which can cause shellfish poisoning if humans eat contaminated shellfish. Some dinoflagellates also exhibit bioluminescence—primarily emitting blue-green light. Thus, some parts of the ocean light up at night giving blue-green light.
Etymology
The term "dinoflagellate" is a combination of the Greek dinos and the Latin flagellum. Dinos means "whirling" and signifies the distinctive way in which dinoflagellates were observed to swim. Flagellum means "whip" and this refers to their flagella.[13]
History
In 1753, the first modern dinoflagellates were described by Henry Baker as "Animalcules which cause the Sparkling Light in Sea Water",[14] and named by Otto Friedrich Müller in 1773.[15] The term derives from the Greek word δῖνος (dînos), meaning whirling, and Latin flagellum, a diminutive term for a whip or scourge.
In the 1830s, the German microscopist Christian Gottfried Ehrenberg examined many water and plankton samples and proposed several dinoflagellate genera that are still used today including Peridinium, Prorocentrum, and Dinophysis.[16]
These same dinoflagellates were first defined by Otto Bütschli in 1885 as the flagellate order Dinoflagellida.[17] Botanists treated them as a division of algae, named Pyrrophyta or Pyrrhophyta ("fire algae"; Greek pyrr(h)os, fire) after the bioluminescent forms, or Dinophyta. At various times, the cryptomonads, ebriids, and ellobiopsids have been included here, but only the last are now considered close relatives. Dinoflagellates have a known ability to transform from noncyst to cyst-forming strategies, which makes recreating their evolutionary history extremely difficult.
Morphology
Dinoflagellates are unicellular and possess two dissimilar flagella arising from the ventral cell side (dinokont flagellation). They have a ribbon-like transverse flagellum with multiple waves that beats to the cell's left, and a more conventional one, the longitudinal flagellum, that beats posteriorly.[18][19][20] The transverse flagellum is a wavy ribbon in which only the outer edge undulates from base to tip, due to the action of the axoneme which runs along it. The axonemal edge has simple hairs that can be of varying lengths. The flagellar movement produces forward propulsion and also a turning force. The longitudinal flagellum is relatively conventional in appearance, with few or no hairs. It beats with only one or two periods to its wave. The flagella lie in surface grooves: the transverse one in the cingulum and the longitudinal one in the sulcus, although its distal portion projects freely behind the cell. In dinoflagellate species with desmokont flagellation (e.g., Prorocentrum), the two flagella are differentiated as in dinokonts, but they are not associated with grooves.
Dinoflagellates have a complex cell covering called an amphiesma or cortex, composed of a series of membranes, flattened vesicles called alveoli (= amphiesmal vesicles) and related structures.[21][22] In thecate ("armoured") dinoflagellates, these support overlapping cellulose plates to create a sort of armor called the theca or lorica, as opposed to athecate ("nude") dinoflagellates. These occur in various shapes and arrangements, depending on the species and sometimes on the stage of the dinoflagellate. Conventionally, the term tabulation has been used to refer to this arrangement of thecal plates. The plate configuration can be denoted with the plate formula or tabulation formula. Fibrous extrusomes are also found in many forms.[23][24]
A transverse groove, the so-called cingulum (or cigulum) runs around the cell, thus dividing it into an anterior (episoma) and posterior (hyposoma). If and only if a theca is present, the parts are called epitheca and hypotheca, respectively. Posteriorly, starting from the transverse groove, there is a longitudinal furrow called the sulcus. The transverse flagellum strikes in the cingulum, the longitudinal flagellum in the sulcus.[25][24]
Together with various other structural and genetic details, this organization indicates a close relationship between the dinoflagellates, the Apicomplexa, and ciliates, collectively referred to as the alveolates.[23]
Dinoflagellate tabulations can be grouped into six "tabulation types": gymnodinoid, suessoid, gonyaulacoid–peridinioid, nannoceratopsioid, dinophysioid, and prorocentroid.[26]
The chloroplasts in most photosynthetic dinoflagellates are bound by three membranes, suggesting they were probably derived from some ingested algae. Most photosynthetic species contain chlorophylls a and c2, the carotenoid beta-carotene, and a group of xanthophylls that appears to be unique to dinoflagellates, typically peridinin, dinoxanthin, and diadinoxanthin. These pigments give many dinoflagellates their typical golden brown color. However, the dinoflagellates Karenia brevis, Karenia mikimotoi, and Karlodinium micrum have acquired other pigments through endosymbiosis, including fucoxanthin.[27] This suggests their chloroplasts were incorporated by several endosymbiotic events involving already colored or secondarily colorless forms. The discovery of plastids in the Apicomplexa has led some to suggest they were inherited from an ancestor common to the two groups, but none of the more basal lines has them. All the same, the dinoflagellate cell consists of the more common organelles such as rough and smooth endoplasmic reticulum, Golgi apparatus, mitochondria, lipid and starch grains, and food vacuoles. Some have even been found with a light-sensitive organelle, the eyespot or stigma, or a larger nucleus containing a prominent nucleolus. The dinoflagellate Erythropsidinium has the smallest known eye.[28]
Some athecate species have an internal skeleton consisting of two star-like siliceous elements that has an unknown function, and can be found as microfossils. Tappan[29] gave a survey of dinoflagellates with internal skeletons. This included the first detailed description of the pentasters in Actiniscus pentasterias, based on scanning electron microscopy. They are placed within the order Gymnodiniales, suborder Actiniscineae.[5]
Theca structure and formation
The formation of thecal plates has been studied in detail through ultrastructural studies.[22]
The dinoflagellate nucleus: dinokaryon
'Core dinoflagellates' (dinokaryotes) have a peculiar form of nucleus, called a dinokaryon, in which the chromosomes are attached to the nuclear membrane. These carry reduced number of histones. In place of histones, dinoflagellate nuclei contain a novel, dominant family of nuclear proteins that appear to be of viral origin, thus are called Dinoflagellate viral nucleoproteins (DVNPs) which are highly basic, bind DNA with similar affinity to histones, and occur in multiple posttranslationally modified forms.[30] Dinoflagellate nuclei remain condensed throughout interphase rather than just during mitosis, which is closed and involves a uniquely extranuclear mitotic spindle.[31] This sort of nucleus was once considered to be an intermediate between the nucleoid region of prokaryotes and the true nuclei of eukaryotes, so were termed "mesokaryotic", but now are considered derived rather than primitive traits (i. e. ancestors of dinoflagellates had typical eukaryotic nuclei). In addition to dinokaryotes, DVNPs can be found in a group of basal dinoflagellates (known as Marine Alveolates, "MALVs") that branch as sister to dinokaryotes (Syndiniales).[32]
Classification
Generality
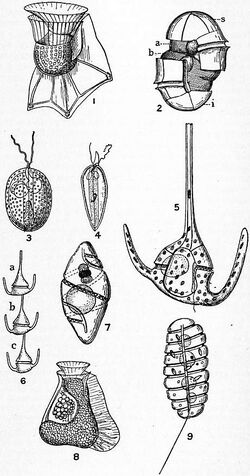
Dinoflagellates are protists and have been classified using both the International Code of Botanical Nomenclature (ICBN, now renamed as ICN) and the International Code of Zoological Nomenclature (ICZN). About half of living dinoflagellate species are autotrophs possessing chloroplasts and half are nonphotosynthesising heterotrophs.
The peridinin dinoflagellates, named after their peridinin plastids, appear to be ancestral for the dinoflagellate lineage. Almost half of all known species have chloroplasts, which are either the original peridinin plastids or new plastids acquired from other lineages of unicellular algae through endosymbiosis. The remaining species have lost their photosynthetic abilities and have adapted to a heterotrophic, parasitic or kleptoplastic lifestyle.[33][34]
Most (but not all) dinoflagellates have a dinokaryon, described below (see: Life cycle, below). Dinoflagellates with a dinokaryon are classified under Dinokaryota, while dinoflagellates without a dinokaryon are classified under Syndiniales.
Although classified as eukaryotes, the dinoflagellate nuclei are not characteristically eukaryotic, as some of them lack histones and nucleosomes, and maintain continually condensed chromosomes during mitosis. The dinoflagellate nucleus was termed 'mesokaryotic' by Dodge (1966),[35] due to its possession of intermediate characteristics between the coiled DNA areas of prokaryotic bacteria and the well-defined eukaryotic nucleus. This group, however, does contain typically eukaryotic organelles, such as Golgi bodies, mitochondria, and chloroplasts.[36]
Jakob Schiller (1931–1937) provided a description of all the species, both marine and freshwater, known at that time.[37] Later, Alain Sournia (1973, 1978, 1982, 1990, 1993) listed the new taxonomic entries published after Schiller (1931–1937).[38][39][40][41][42] Sournia (1986) gave descriptions and illustrations of the marine genera of dinoflagellates, excluding information at the species level.[43] The latest index is written by Gómez.[2]
Identification
English-language taxonomic monographs covering large numbers of species are published for the Gulf of Mexico,[44] the Indian Ocean,[45] the British Isles,[46] the Mediterranean[47] and the North Sea.[48]
The main source for identification of freshwater dinoflagellates is the Süsswasser Flora.[49]
Calcofluor-white can be used to stain thecal plates in armoured dinoflagellates.[50]
Ecology and physiology
Habitats
Dinoflagellates are found in all aquatic environments: marine, brackish, and fresh water, including in snow or ice. They are also common in benthic environments and sea ice.
Endosymbionts
All Zooxanthellae are dinoflagellates and most of them are members within Symbiodiniaceae (e.g. the genus Symbiodinium).[51] The association between Symbiodinium and reef-building corals is widely known. However, endosymbiontic Zooxanthellae inhabit a great number of other invertebrates and protists, for example many sea anemones, jellyfish, nudibranchs, the giant clam Tridacna, and several species of radiolarians and foraminiferans.[52] Many extant dinoflagellates are parasites (here defined as organisms that eat their prey from the inside, i.e. endoparasites, or that remain attached to their prey for longer periods of time, i.e. ectoparasites). They can parasitize animal or protist hosts. Protoodinium, Crepidoodinium, Piscinoodinium, and Blastodinium retain their plastids while feeding on their zooplanktonic or fish hosts. In most parasitic dinoflagellates, the infective stage resembles a typical motile dinoflagellate cell.
Nutritional strategies
Three nutritional strategies are seen in dinoflagellates: phototrophy, mixotrophy, and heterotrophy. Phototrophs can be photoautotrophs or auxotrophs. Mixotrophic dinoflagellates are photosynthetically active, but are also heterotrophic. Facultative mixotrophs, in which autotrophy or heterotrophy is sufficient for nutrition, are classified as amphitrophic. If both forms are required, the organisms are mixotrophic sensu stricto. Some free-living dinoflagellates do not have chloroplasts, but host a phototrophic endosymbiont. A few dinoflagellates may use alien chloroplasts (cleptochloroplasts), obtained from food (kleptoplasty). Some dinoflagellates may feed on other organisms as predators or parasites.[53]
Food inclusions contain bacteria, bluegreen algae, diatoms, ciliates, and other dinoflagellates.[54][55][56][57][58][59][60]
Mechanisms of capture and ingestion in dinoflagellates are quite diverse. Several dinoflagellates, both thecate (e.g. Ceratium hirundinella,[59] Peridinium globulus[57]) and nonthecate (e.g. Oxyrrhis marina,[55] Gymnodinium sp.[61] and Kofoidinium spp.[62]), draw prey to the sulcal region of the cell (either via water currents set up by the flagella or via pseudopodial extensions) and ingest the prey through the sulcus. In several Protoperidinium spp., e.g. P. conicum, a large feeding veil—a pseudopod called the pallium—is extruded to capture prey which is subsequently digested extracellularly (= pallium-feeding).[63][64] Oblea, Zygabikodinium, and Diplopsalis are the only other dinoflagellate genera known to use this particular feeding mechanism.[64][65][66] Katodinium (Gymnodinium) fungiforme, commonly found as a contaminant in algal or ciliate cultures, feeds by attaching to its prey and ingesting prey cytoplasm through an extensible peduncle.[67] Two related species, polykrikos kofoidii and neatodinium, shoots out a harpoon-like organelle to capture prey.[68]
Some mixotrophic dinoflagellates are able to produce neurotoxins that have anti-grazing effects on larger copepods and enhance the ability of the dinoflagellate to prey upon larger copepods. Toxic strains of K. veneficum produce karlotoxin that kills predators who ingest them, thus reducing predatory populations and allowing blooms of both toxic and non-toxic strains of K. veneficum. Further, the production of karlotoxin enhances the predatory ability of K. veneficum by immobilizing its larger prey.[69] K. arminger are more inclined to prey upon copepods by releasing a potent neurotoxin that immobilizes its prey upon contact. When K. arminger are present in large enough, they are able to cull whole populations of its copepods prey.[70]
The feeding mechanisms of the oceanic dinoflagellates remain unknown, although pseudopodial extensions were observed in Podolampas bipes.[71]
Blooms
Introduction
Dinoflagellate blooms are generally unpredictable, short, with low species diversity, and with little species succession.[72] The low species diversity can be due to multiple factors. One way a lack of diversity may occur in a bloom is through a reduction in predation and a decreased competition. The first may be achieved by having predators reject the dinoflagellate, by, for example, decreasing the amount of food it can eat. This additionally helps prevent a future increase in predation pressure by cause predators that reject it to lack the energy to breed. A species can then inhibit the growth of its competitors, thus achieving dominance.[73]
Harmful algal blooms
Dinoflagellates sometimes bloom in concentrations of more than a million cells per millilitre. Under such circumstances, they can produce toxins (generally called dinotoxins) in quantities capable of killing fish and accumulating in filter feeders such as shellfish, which in turn may be passed on to people who eat them. This phenomenon is called a red tide, from the color the bloom imparts to the water. Some colorless dinoflagellates may also form toxic blooms, such as Pfiesteria. Some dinoflagellate blooms are not dangerous. Bluish flickers visible in ocean water at night often come from blooms of bioluminescent dinoflagellates, which emit short flashes of light when disturbed.
A red tide occurs because dinoflagellates are able to reproduce rapidly and copiously as a result of the abundant nutrients in the water. Although the resulting red waves are an interesting visual phenomenon, they contain toxins that not only affect all marine life in the ocean, but the people who consume them, as well.[74] A specific carrier is shellfish. This can introduce both nonfatal and fatal illnesses. One such poison is saxitoxin, a powerful paralytic neurotoxin.[75][76][77]
Human inputs of phosphate further encourage these red tides, so strong interest exists in learning more about dinoflagellates, from both medical and economic perspectives. Dinoflagellates are known to be particularly capable of scavenging dissolved organic phosphorus for P-nutrient, several HAS species have been found to be highly versatile and mechanistically diversified in utilizing different types of DOPs.[75][76][77] The ecology of harmful algal blooms is extensively studied.[78]
Bioluminescence
File:Kayaking in the Bioluminescent Bay Vieques.webm At night, water can have an appearance of sparkling light due to the bioluminescence of dinoflagellates.[79][80] More than 18 genera of dinoflagellates are bioluminescent,[81] and the majority of them emit a blue-green light.[82] These species contain scintillons, individual cytoplasmic bodies (about 0.5 µm in diameter) distributed mainly in the cortical region of the cell, outpockets of the main cell vacuole. They contain dinoflagellate luciferase, the main enzyme involved in dinoflagellate bioluminescence, and luciferin, a chlorophyll-derived tetrapyrrole ring that acts as the substrate to the light-producing reaction. The luminescence occurs as a brief (0.1 sec) blue flash (max 476 nm) when stimulated, usually by mechanical disturbance. Therefore, when mechanically stimulated—by boat, swimming, or waves, for example—a blue sparkling light can be seen emanating from the sea surface.[83]
Dinoflagellate bioluminescence is controlled by a circadian clock and only occurs at night.[84] Luminescent and nonluminescent strains can occur in the same species. The number of scintillons is higher during night than during day, and breaks down during the end of the night, at the time of maximal bioluminescence.[85]
The luciferin-luciferase reaction responsible for the bioluminescence is pH sensitive.[83] When the pH drops, luciferase changes its shape, allowing luciferin, more specifically tetrapyrrole, to bind.[83] Dinoflagellates can use bioluminescence as a defense mechanism. They can startle their predators by their flashing light or they can ward off potential predators by an indirect effect such as the "burglar alarm". The bioluminescence attracts attention to the dinoflagellate and its attacker, making the predator more vulnerable to predation from higher trophic levels.[83]
Bioluminescent dinoflagellate ecosystem bays are among the rarest and most fragile,[86] with the most famous ones being the Bioluminescent Bay in La Parguera, Lajas, Puerto Rico; Mosquito Bay in Vieques, Puerto Rico; and Las Cabezas de San Juan Reserva Natural Fajardo, Puerto Rico. Also, a bioluminescent lagoon is near Montego Bay, Jamaica, and bioluminescent harbors surround Castine, Maine.[87] Within the United States, Central Florida is home to the Indian River Lagoon which is abundant with dinoflagellates in the summer and bioluminescent ctenophore in the winter.[88]
Lipid and sterol production
Dinoflagellates produce characteristic lipids and sterols.[89] One of these sterols is typical of dinoflagellates and is called dinosterol.
Transport
Dinoflagellate theca can sink rapidly to the seafloor in marine snow.[90]
Life cycle
Introduction
Dinoflagellates have a haplontic life cycle, with the possible exception of Noctiluca and its relatives.[5] The life cycle usually involves asexual reproduction by means of mitosis, either through desmoschisis or eleuteroschisis. More complex life cycles occur, more particularly with parasitic dinoflagellates. Sexual reproduction also occurs,[91] though this mode of reproduction is only known in a small percentage of dinoflagellates.[92] This takes place by fusion of two individuals to form a zygote, which may remain mobile in typical dinoflagellate fashion and is then called a planozygote. This zygote may later form a resting stage or hypnozygote, which is called a dinoflagellate cyst or dinocyst. After (or before) germination of the cyst, the hatchling undergoes meiosis to produce new haploid cells. Dinoflagellates appear to be capable of carrying out several DNA repair processes that can deal with different types of DNA damage.[93]

Dinoflagellate cysts
The life cycle of many dinoflagellates includes at least one nonflagellated benthic stage as a cyst. Different types of dinoflagellate cysts are mainly defined based on morphological (number and type of layers in the cell wall) and functional (long- or short-term endurance) differences. These characteristics were initially thought to clearly distinguish pellicle (thin-walled) cysts from resting (double-walled) dinoflagellate cysts. The former were considered short-term (temporal) and the latter long-term (resting) cysts. However, during the last two decades further knowledge has highlighted the great intricacy of dinoflagellate life histories.[94]

More than 10% of the approximately 2000 known marine dinoflagellate species produce cysts as part of their life cycle (see diagram on the right). These benthic phases play an important role in the ecology of the species, as part of a planktonic-benthic link in which the cysts remain in the sediment layer during conditions unfavorable for vegetative growth and, from there, reinoculate the water column when favorable conditions are restored.[94]
Indeed, during dinoflagellate evolution the need to adapt to fluctuating environments and/or to seasonality is thought to have driven the development of this life cycle stage. Most protists form dormant cysts in order to withstand starvation and UV damage.[95] However, there are enormous differences in the main phenotypic, physiological and resistance properties of each dinoflagellate species cysts. Unlike in higher plants most of this variability, for example in dormancy periods, has not been proven yet to be attributed to latitude adaptation or to depend on other life cycle traits.[96][97] Thus, despite recent advances in the understanding of the life histories of many dinoflagellate species, including the role of cyst stages, many gaps remain in knowledge about their origin and functionality.[94]
Recognition of the capacity of dinoflagellates to encyst dates back to the early 20th century, in biostratigraphic studies of fossil dinoflagellate cysts. Paul Reinsch was the first to identify cysts as the fossilized remains of dinoflagellates.[98] Later, cyst formation from gamete fusion was reported, which led to the conclusion that encystment is associated with sexual reproduction.[91] These observations also gave credence to the idea that microalgal encystment is essentially a process whereby zygotes prepare themselves for a dormant period.[99] Because the resting cysts studied until that time came from sexual processes, dormancy was associated with sexuality, a presumption that was maintained for many years. This attribution was coincident with evolutionary theories about the origin of eukaryotic cell fusion and sexuality, which postulated advantages for species with diploid resting stages, in their ability to withstand nutrient stress and mutational UV radiation through recombinational repair, and for those with haploid vegetative stages, as asexual division doubles the number of cells.[95] Nonetheless, certain environmental conditions may limit the advantages of recombination and sexuality,[100] such that in fungi, for example, complex combinations of haploid and diploid cycles have evolved that include asexual and sexual resting stages.[101][94]
However, in the general life cycle of cyst-producing dinoflagellates as outlined in the 1960s and 1970s, resting cysts were assumed to be the fate of sexuality,[91][102] which itself was regarded as a response to stress or unfavorable conditions. Sexuality involves the fusion of haploid gametes from motile planktonic vegetative stages to produce diploid planozygotes that eventually form cysts, or hypnozygotes, whose germination is subject to both endogenous and exogenous controls. Endogenously, a species-specific physiological maturation minimum period (dormancy) is mandatory before germination can occur. Thus, hypnozygotes were also referred to as "resting" or "resistant" cysts, in reference to this physiological trait and their capacity following dormancy to remain viable in the sediments for long periods of time. Exogenously, germination is only possible within a window of favorable environmental conditions.[94]
Yet, with the discovery that planozygotes were also able to divide it became apparent that the complexity of dinoflagellate life cycles was greater than originally thought.[103][104] Following corroboration of this behavior in several species, the capacity of dinoflagellate sexual phases to restore the vegetative phase, bypassing cyst formation, became well accepted.[105][106] Further, in 2006 Kremp and Parrow showed the dormant resting cysts of the Baltic cold water dinoflagellates Scrippsiella hangoei and Gymnodinium sp. were formed by the direct encystment of haploid vegetative cells, i.e., asexually.[107] In addition, for the zygotic cysts of Pfiesteria piscicida dormancy was not essential.[108][94]
Genomics
One of the most striking features of dinoflagellates is the large amount of cellular DNA that they contain. Most eukaryotic algae contain on average about 0.54 pg DNA/cell, whereas estimates of dinoflagellate DNA content range from 3–250 pg/cell,[31] corresponding to roughly 3000–215 000 Mb (in comparison, the haploid human genome is 3180 Mb and hexaploid Triticum wheat is 16 000 Mb). Polyploidy or polyteny may account for this large cellular DNA content,[109] but earlier studies of DNA reassociation kinetics and recent genome analyses do not support this hypothesis.[110] Rather, this has been attributed, hypothetically, to the rampant retroposition found in dinoflagellate genomes.[111][112]
In addition to their disproportionately large genomes, dinoflagellate nuclei are unique in their morphology, regulation, and composition. Their DNA is so tightly packed that exactly how many chromosomes they have is still uncertain.[113]
The dinoflagellates share an unusual mitochondrial genome organisation with their relatives, the Apicomplexa.[114] Both groups have very reduced mitochondrial genomes (around 6 kilobases (kb) in the Apicomplexa vs ~16kb for human mitochondria). One species, Amoebophrya ceratii, has lost its mitochondrial genome completely, yet still has functional mitochondria.[115] The genes on the dinoflagellate genomes have undergone a number of reorganisations, including massive genome amplification and recombination which have resulted in multiple copies of each gene and gene fragments linked in numerous combinations. Loss of the standard stop codons, trans-splicing of mRNAs for the mRNA of cox3, and extensive RNA editing recoding of most genes has occurred.[116][117] The reasons for this transformation are unknown. In a small group of dinoflagellates, called 'dinotoms' (Durinskia and Kryptoperidinium), the endosymbionts (diatoms) still have mitochondria, making them the only organisms with two evolutionarily distinct mitochondria.[118]
In most of the species, the plastid genome consist of just 14 genes.[119]
The DNA of the plastid in the peridinin-containing dinoflagellates is contained in a series of small circles called minicircles.[120] Each circle contains one or two polypeptide genes. The genes for these polypeptides are chloroplast-specific because their homologs from other photosynthetic eukaryotes are exclusively encoded in the chloroplast genome. Within each circle is a distinguishable 'core' region. Genes are always in the same orientation with respect to this core region.
In terms of DNA barcoding, ITS sequences can be used to identify species,[121] where a genetic distance of p≥0.04 can be used to delimit species,[122] which has been successfully applied to resolve long-standing taxonomic confusion as in the case of resolving the Alexandrium tamarense complex into five species.[123] A recent study[124] revealed a substantial proportion of dinoflagellate genes encode for unknown functions, and that these genes could be conserved and lineage-specific.
Evolutionary history
Dinoflagellates are mainly represented as fossils by dinocysts, which have a long geological record with lowest occurrences during the mid-Triassic,[125] whilst geochemical markers suggest a presence to the Early Cambrian.[126] Some evidence indicates dinosteroids in many Paleozoic and Precambrian rocks might be the product of ancestral dinoflagellates (protodinoflagellates).[127][128] Dinoflagellates show a classic radiation of morphologies during the Late Triassic through the Middle Jurassic.[129][130][131] More modern-looking forms proliferate during the later Jurassic and Cretaceous.[129] This trend continues into the Cenozoic, albeit with some loss of diversity.[129][125]
Molecular phylogenetics show that dinoflagellates are grouped with ciliates and apicomplexans (=Sporozoa) in a well-supported clade, the alveolates. The closest relatives to dinokaryotic dinoflagellates appear to be apicomplexans, Perkinsus, Parvilucifera, syndinians, and Oxyrrhis.[132] Molecular phylogenies are similar to phylogenies based on morphology.[133][134]
The earliest stages of dinoflagellate evolution appear to be dominated by parasitic lineages, such as perkinsids and syndinians (e.g. Amoebophrya and Hematodinium).[135][136][137][138]
All dinoflagellates contain red algal plastids or remnant (nonphotosynthetic) organelles of red algal origin.[139] The parasitic dinoflagellate Hematodinium however lacks a plastid entirely.[140] Some groups that have lost the photosynthetic properties of their original red algae plastids has obtained new photosynthetic plastids (chloroplasts) through so-called serial endosymbiosis, both secondary and tertiary. Like their original plastids, the new chloroplasts in these groups can be traced back to red algae, except from those in the members of the genus Lepidodinium, which possess plastids derived from green algae, possibly Trebouxiophyceae or Ulvophyceae.[141][142] Lineages with tertiary endosymbiosis are Dinophysis, with plastids from a cryptomonad,[143] the Karenia, Karlodinium, and Takayama, which possess plastids of haptophyte origin, and the Kryptoperidiniaceae, Durinskia and Kryptoperidinium, which have plastids derived from diatoms[144][145] Some species also perform kleptoplasty.[146]
Dinoflagellate evolution has been summarized into five principal organizational types: prorocentroid, dinophysoid, gonyaulacoid, peridinioid, and gymnodinoid.[147] The transitions of marine species into fresh water have been frequent events during the diversification of dinoflagellates and have occurred recently.[148]
Many dinoflagellates also have a symbiotic relationship with cyanobacteria, called cyanobionts, which have a reduced genome and has not been found outside their hosts. The Dinophysoid dinoflagellates have two genera, Amphisolenia and Triposolenia, that contain intracellular cyanobionts, and four genera; Citharistes, Histioneis, Parahistioneis, and Ornithocercus, that contain extracellular cyanobionts.[149] Most of the cyanobionts are used for nitrogen fixation, not for photosynthesis, but some don't have the ability to fix nitrogen. The dinoflagellate Ornithocercus magnificus is host for symbionts which resides in an extracellular chamber. While it is not fully known how the dinoflagellate benefit from it, it has been suggested it is farming the cyanobacteria in specialized chambers and regularly digest some of them.[150]
Recently, the living fossil Dapsilidinium pastielsii was found inhabiting the Indo-Pacific Warm Pool, which served as a refugium for thermophilic dinoflagellates,[151] and others such as Calciodinellum operosum and Posoniella tricarinelloides were also described from fossils before later being found alive.[152][153]
Examples
- Alexandrium
- Gonyaulax
- Gymnodinium
- Lingulodinium polyedrum
Unknown dinoflagellate under SEM (Dinophyceae)
Symbiodinium sp. (Dinophyceae): zooxanthella, a coral endosymbiont
Noctiluca scintillans (Noctiluciphyceae)
See also
References
- ↑ "Estimating the timing of early eukaryotic diversification with multigene molecular clocks". Proceedings of the National Academy of Sciences of the United States of America 108 (33): 13624–9. August 2011. doi:10.1073/pnas.1110633108. PMID 21810989. Bibcode: 2011PNAS..10813624P.
- ↑ 2.0 2.1 2.2 Gómez F (2012). "A checklist and classification of living dinoflagellates (Dinoflagellata, Alveolata)". CICIMAR Oceánides 27 (1): 65–140. doi:10.37543/oceanides.v27i1.111.
- ↑ Ruggiero (2015), "Higher Level Classification of All Living Organisms", PLOS ONE 10 (4): e0119248, doi:10.1371/journal.pone.0119248, PMID 25923521, Bibcode: 2015PLoSO..1019248R
- ↑ Silar, Philippe (2016), "Protistes Eucaryotes: Origine, Evolution et Biologie des Microbes Eucaryotes", HAL Archives-ouvertes: 1–462, ISBN 9782955584101, OCLC 1019558675, https://hal.archives-ouvertes.fr/hal-01263138, retrieved 2016-09-04
- ↑ 5.0 5.1 5.2 A classification of living and fossil dinoflagellates. Micropaleontology Special Publication. 7. Hanover PA: Sheridan Press. 1993. OCLC 263894965.
- ↑ Stoecker, D. K. (1999). "Mixotrophy among Dinoflagellates". The Journal of Eukaryotic Microbiology 46 (4): 397–401. doi:10.1111/j.1550-7408.1999.tb04619.x.
- ↑ Esser, Karl; Lüttge, Ulrich; Beyschlag, Wolfram; Murata, Jin (2012-12-06). Progress in Botany: Genetics Physiology Systematics Ecology. Springer. ISBN 978-3-6421-8819-0. https://books.google.com/books?id=BuL7CAAAQBAJ&q=%22Some+of+the+photosynthetic+species+are+mixotrophic+and+feed+by+myzocytosis%22&pg=PA36. Retrieved 2020-10-22.
- ↑ "How many species of algae are there?". Journal of Phycology 48 (5): 1057–63. October 2012. doi:10.1111/j.1529-8817.2012.01222.x. PMID 27011267. Bibcode: 2012JPcgy..48.1057G.
- ↑ "A review of recent freshwater dinoflagellate cysts: Taxonomy, phylogeny, ecology and palaeocology". Phycologia 51 (6): 612–619. 2012. doi:10.2216/11-89.1. Bibcode: 2012Phyco..51..612M.
- ↑ "Towards an Ecological Understanding of Dinoflagellate Cyst Functions". Microorganisms 2 (1): 11–32. January 2014. doi:10.3390/microorganisms2010011. PMID 27694774.
- ↑ Gómez, F. (2005). "A list of free-living dinoflagellate species in the world's oceans". Acta Botanica Croatica 64 (1): 129–212. https://hrcak.srce.hr/clanak/5709.
- ↑ "Dinoflagellate diversity and distribution". Biodivers. Conserv. 17 (2): 407–418. February 2008. doi:10.1007/s10531-007-9258-3. Bibcode: 2008BiCon..17..407T.
- ↑ Carty, Susan; Parrow, Matthew W. (2015). "Dinoflagellates". Freshwater Algae of North America. Academic Press. pp. 773–807. doi:10.1016/B978-0-12-385876-4.00017-7. ISBN 978-0-12-385876-4.
- ↑ Baker, M. (1753). Employment for the microscope. London: Dodsley. doi:10.5962/bhl.title.45920. OCLC 722119426. http://catalog.hathitrust.org/api/volumes/oclc/4322834.html.
- ↑ Müller, O.F. 1773. Vermium terrestrium et fluviatilium, seu Animalium Infusoriorum, Helmithicorum et Testaceorum, non marinorum, succincta historia, vol. 1. Pars prima. p. 34, 135. Faber, Havniae, et Lipsiae 1773.
- ↑ Ehrenberg C.G. (1832) Beiträge zur Kenntnis der Organisation der Infusorien und ihrer geographischer Verbreitung, besonders in Sibirien. — Abhandlungen der Königlichen Akademie der Wissenschaften zu Berlin. Aus dem Jahre 1830. Physikalische Abhandlungen 1830: 1–88, Pls 1–8.
- ↑ Bütschli O. (1885) 3. Unterabtheilung (Ordnung) Dinoflagellata. – In: Dr. H.G. Bronn's Klassen und Ordnungen des Thier-Reichs, wissenschaftlich dargestellt in Wort und Bild. Erster Band Protozoa. – C.F. Winter'sche Verlagshandlung, Leipzig und Heidelberg. Pp. 906–1029; Pl.
- ↑ Taylor FJR (March 1975). "Non-helical transverse flagella in dinoflagellates". Phycologia 14 (1): 45–7. doi:10.2216/i0031-8884-14-1-45.1. Bibcode: 1975Phyco..14...45T.
- ↑ "The propulsive mechanism of the dinoflagellate transverse flagellum reconsidered". Bio Systems 8 (1): 33–9. April 1976. doi:10.1016/0303-2647(76)90005-8. PMID 986199. Bibcode: 1976BiSys...8...33L.
- ↑ "Form and function of the dinoflagellate transverse flagellum". J. Protozool. 32 (2): 290–6. May 1985. doi:10.1111/j.1550-7408.1985.tb03053.x.
- ↑ Ultrastructure of the dinoflagellate amphiesma. International Review of Cytology. 82. 1983. pp. 151–80. doi:10.1016/s0074-7696(08)60825-6. ISBN 978-0-1236-4482-4.
- ↑ 22.0 22.1 Netzel, Harald; Dürr, Georg (2012-12-02). Ch. 3: Dinoflagellate cell cortex. Academic Press. pp. 43–106. ISBN 978-0-3231-3813-0. https://books.google.com/books?id=qCcgFximE8oC&pg=PA43. Retrieved 2016-03-05. In Spector 1984
- ↑ 23.0 23.1 Cavalier-Smith, T. (1991). "Cell diversification in heterotrophic flagellates". in Patterson, D.J.; Larsen, J.. The biology of free-living heterotrophic flagellates. Systematics Association Publications. 45. Clarendon Press. pp. 113–131. ISBN 978-0-1985-7747-8.
- ↑ 24.0 24.1 Freudenthal, Hugo D. (1962). "Symbiodinium gen. Nov. And Symbiodinium microadriaticum sp. nov., a Zooxanthella: Taxonomy, Life Cycle, and Morphology". The Journal of Protozoology 9 (1): 45–52. doi:10.1111/j.1550-7408.1962.tb02579.x.
- ↑ Trench, Robert K.; Blank, Rudolf J. (1987). "Symbiodinium microadriaticum Freudenthal, S. goreauii sp. nov., S. kawagutii sp. nov. And S. pilosum sp. nov.: Gymnodinioid Dinoflagellate Symbionts of Marine Invertebrates". Journal of Phycology 23 (3): 469–81. doi:10.1111/j.1529-8817.1987.tb02534.x. Bibcode: 1987JPcgy..23..469T.
- ↑ Medlin, L. K.; Fensome, R. A. (2013), "Dinoflagellate macroevolution", Biological and Geological Perspectives of Dinoflagellates (Geological Society of London): pp. 263–274, doi:10.1144/tms5.25, ISBN 978-1-86239-368-4, https://doi.org/10.1144/TMS5.25, retrieved 2023-08-20
- ↑ "Dinoflagellates: a remarkable evolutionary experiment". American Journal of Botany 91 (10): 1523–34. October 2004. doi:10.3732/ajb.91.10.1523. PMID 21652307.
- ↑ "You are what you eat". The British Journal of Ophthalmology 88 (9): 1113. September 2004. doi:10.1136/bjo.2004.049510. PMID 15352316.
- ↑ Tappan, H.N. (1980). The Paleobiology of Plant Protists. Geology. W.H. Freeman. ISBN 978-0-7167-1109-4.
- ↑ "Loss of nucleosomal DNA condensation coincides with appearance of a novel nuclear protein in dinoflagellates". Current Biology 22 (24): 2303–12. December 2012. doi:10.1016/j.cub.2012.10.036. PMID 23159597.
- ↑ 31.0 31.1 Spector, D.L. (2012-12-02). Dinoflagellate nuclei. Academic Press. pp. 107–147. ISBN 978-0-3231-3813-0. https://books.google.com/books?id=qCcgFximE8oC&pg=PA107. Retrieved 2016-03-05. In Spector 1984
- ↑ "Single cell genomics of uncultured marine alveolates shows paraphyly of basal dinoflagellates". The ISME Journal 12 (1): 304–308. January 2018. doi:10.1038/ismej.2017.167. PMID 28994824. PMC 5739020. Bibcode: 2018ISMEJ..12..304S. http://discovery.ucl.ac.uk/10030027/. Retrieved 2018-10-23.
- ↑ Gabrielsen, T. M.; Minge, M. A.; Espelund, M.; Tooming-Klunderud, A.; Patil, V.; Nederbragt, A. J.; Otis, C.; Turmel, M. et al. (2011). "Genome Evolution of a Tertiary Dinoflagellate Plastid – PLOS". PLOS ONE 6 (4): e19132. doi:10.1371/journal.pone.0019132. PMID 21541332.
- ↑ Bodył, Andrzej; Moszczyński, Krzysztof (2006). "Did the peridinin plastid evolve through tertiary endosymbiosis? A hypothesis". European Journal of Phycology 41 (4): 435–448. doi:10.1080/09670260600961080. Bibcode: 2006EJPhy..41..435B.
- ↑ Dodge (1966). Cited but unreferenced in Steidinger KA; Jangen K (1997). "Dinoflagellates". in Tomas, C.R.. Identifying Marine Phytoplankton. Academic Press. pp. 387–584. ISBN 978-0-0805-3442-8. https://books.google.com/books?id=8WLABHmo-K8C&pg=PA387. Retrieved 2016-03-05.
- ↑ "Dinoflagellates". Identifying Marine Phytoplankton. Academic Press. 1997. pp. 387–584. ISBN 978-0-0805-3442-8. https://books.google.com/books?id=8WLABHmo-K8C&pg=PA387. Retrieved 2016-03-05.
- ↑ Schiller, J., 1931–1937: Dinoflagellatae (Peridinineae) in monographischer Behandlung. In: RABENHORST, L. (ed.), Kryptogamen-Flora von Deutschland, Österreichs und der Schweiz. Akad. Verlag., Leipzig. Vol. 10 (3): Teil 1 (1–3) (1931–1933): Teil 2 (1–4)(1935–1937).
- ↑ Sournia A (1973). "Catalogue des espèces et taxons infraspécifiques de dinoflagellés marins actuels publiés depuis la révision de J. Schiller. I. Dinoflagellés libres". Beih. Nova Hedwigia 48: 1–92.
- ↑ Sournia A (1978). "Catalogue des espèces et taxons infraspécifiques de dinoflagellésmarins actuels publiés depuis la révision de J. Schiller. III (Complément)". Rev. Algol. 13: 3–40 +erratum 13, 186. ISSN 0035-0702. https://www.biodiversitylibrary.org/item/281428#page/4/mode/2up.
- ↑ Sournia A (1982). "Catalogue des espèces et taxons infraspécifiques de dinoflagellésmarins actuels publiés depuis la révision de J. Schiller. IV. (Complément)". Arch. Protistenkd. 126 (2): 151–168. doi:10.1016/S0003-9365(82)80046-8.
- ↑ Sournia A (1990). "Catalogue des espèces et taxons infraspécifiques de dinoflagellésmarins actuels publiés depuis la révision de J. Schiller. V. (Complément)". Acta Protozool. 29: 321–346. ISSN 0065-1583.
- ↑ Sournia A (1993). "Catalogue des espèces et taxons infraspécifiques de dinoflagellésmarins actuels publiés depuis la révision de J. Schiller. VI. (Complément)". Cryptog. Algol. 14: 133–144. ISSN 0181-1568.
- ↑ SOURNIA, A., 1986: Atlas du Phytoplancton Marin. Vol. I: Introduction, Cyanophycées,Dictyochophycées, Dinophycées et Raphidophycées. Editions du CNRS, Paris.
- ↑ Dinoflagellates. Memoirs of the Hourglass Cruises. 2. Florida: Marine Research Laboratory. 1970. OCLC 6206528.
- ↑ Taylor, F.J.R. (1976). Dinoflagellates from the International Indian Ocean Expedition: A Report on Material Collected by the R.V. "Anton Bruun" 1963–1964. Biblioteca Botanica. 132. E. Schweizerbart. ISBN 978-3-5104-8003-6. OCLC 3026853.
- ↑ Dodge, J.D.; Hart-Jones, B. (1982). Marine Dinoflagellates of the British Isles. London: Her Majesty's Stationery Office. ISBN 9780112411963. OCLC 681855348. http://catalog.hathitrust.org/api/volumes/oclc/9407842.html.
- ↑ Gómez F (April 2003). "Checklist of Mediterranean free-living dinoflagellates". Botanica Marina 46 (3): 215–242. doi:10.1515/BOT.2003.021.
- ↑ Marine phytoplankton: selected microphytoplankton species from the North Sea around Helgoland and Sylt. Stuttgart: E. Schweizerbart'sche Verlagsbuchhandlung (Nägele und Obermiller). 2009. ISBN 978-3-5106-1392-2.
- ↑ Dinophyceae (Dinoflagellida). Süßwasserflora von Mitteleuropa. 6. Fischer. 1990. ISBN 978-3-3340-0247-6. https://books.google.com/books?id=MXIVAQAAIAA.[yes|permanent dead link|dead link}}]
- ↑ "A rapid simple technique utilizing calcofluor white M2R for the visualization of dinoflagellate thecal plates". J. Phycol. 21 (4): 662–4. December 1985. doi:10.1111/j.0022-3646.1985.00662.x. Bibcode: 1985JPcgy..21..662F.
- ↑ Freudenthal et al. 2007
- ↑ Trench, R.K. (1997). "Diversity of symbiotic dinoflagellates and the evolution of microalgal-invertebrate symbioses". in Lessios, H.A.; MacIntyre, I.G.. Proceedings of the eighth International Coral Reef Symposium, Panama, June 24–29, 1996. 2. Balboa, Panama: Smithsonian Tropical Research Institute. pp. 1275–86. OCLC 833272061. http://www.reefbase.org/download/download.aspx?type=1&docid=8278.
- ↑ "Nutritional strategies in dinoflagellates: A review with emphasis on cell biological aspects". European Journal of Protistology 28 (1): 3–24. February 1992. doi:10.1016/S0932-4739(11)80315-9. PMID 23194978.
- ↑ "The free-living unarmoured dinoflagellata". Mere. Univ. Calif. 5: 1–538. 1921. doi:10.5962/bhl.title.24995. https://www.biodiversitylibrary.org/itempdf/66471. Retrieved 2019-09-24.
- ↑ 55.0 55.1 Barker HA (1935). "The culture and physiology of the marine dinoflagellates". Arch. Mikrobiol. 6 (1–5): 157–181. doi:10.1007/BF00407285.
- ↑ Biecheler B (1952). "Recherches sur les Peridiniens". Bull. Biol. Fr. Belg. 36 (Suppl): 1–149. ISSN 0994-575X.
- ↑ 57.0 57.1 Bursa AS (1961). "The annual oceanographic cycle at Igloolik in the Canadian Arctic. II. The phytoplankton". J. Fish. Res. Board Can. 18 (4): 563–615. doi:10.1139/f61-046.
- ↑ Norris DR (1969). "Possible phagotrophic feeding in Ceratium lunula Schimper". Limnol. Oceanogr. 14 (3): 448–9. doi:10.4319/lo.1969.14.3.0448. Bibcode: 1969LimOc..14..448N.
- ↑ 59.0 59.1 "The morphology and fine structure of Ceratium hirundinella (Dinophyceae)". J. Phycol. 6 (2): 137–149. June 1970. doi:10.1111/j.1529-8817.1970.tb02372.x. Bibcode: 1970JPcgy...6..137D.
- ↑ Elbrachter M (1979). "On the taxonomy of unarmored dinophytes (Dinophyta) from the Northwest African upwelling region". Meteor Forschungsergebnisse 30: 1–22.
- ↑ "Dinoflagellate phagotrophy in the upper Great Lakes". Trans. Am. Microsc. Soc. 99 (4): 439–444. 1980. doi:10.2307/3225654.
- ↑ "Le systeme stomatopharyngien de Kofoidinium Pavillard. Comparisons avec celui divers Peridiniens fibres et parasites". Protistologica 10: 217–222.
- ↑ "Extracellular digestion in marine dinoflagellates". J. Plankton Res. 6 (6): 1057–61. 1984. doi:10.1093/plankt/6.6.1057.
- ↑ 64.0 64.1 "Thecate heterotrophic dinoflagellates: feeding behavior and mechanisms". J. Phycol. 22 (3): 249–258. September 1986. doi:10.1111/j.1529-8817.1986.tb00021.x.
- ↑ "Feeding, growth, and behavior of the thecate heterotrophic dinoflagellate Oblea rotunda". Limnol. Oceanogr. 38 (5): 965–977. 1993. doi:10.4319/lo.1993.38.5.0965. Bibcode: 1993LimOc..38..965S.
- ↑ Naustvoll LJ (January 1998). "Growth and grazing by the thecate heterotrophic dinoflagellates Diplopsalis lenticula (Diplopsalidaceae, Dinophyceae)". Phycologia 37 (1): 1–9. doi:10.2216/i0031-8884-37-1-1.1. Bibcode: 1998Phyco..37....1N.
- ↑ Spero HJ (September 1982). "Phagotrophy in Gymnodinium fungiforme (Pyrrophyta): the peduncle as an organelle of ingestion". J. Phycol. 18 (3): 356–360. doi:10.1111/j.1529-8817.1982.tb03196.x. Bibcode: 1982JPcgy..18..356S.
- ↑ "Researchers capture dinoflagellate on video shooting harpoons at prey". https://phys.org/news/2017-04-capture-dinoflagellate-video-harpoons-prey.html.
- ↑ Adolf, Jason E; Krupatkina, Danara; Bachvaroff, Tsvetan; Place, Allen R. (2007). "Karlotoxin mediates grazing by Oxyrrhis marina on strains of Karlodinium veneficum". Harmful Algae 6 (3): 400–412. doi:10.1016/j.hal.2006.12.003.
- ↑ Berge, Terje; Poulsen, Louise K.; Moldrup, Morten; Daugbjerg, Niels; Juel Hansen, Per (2012). "Marine microalgae attack and feed on metazoans". ISME J 6 (10): 1926–36. doi:10.1038/ismej.2012.29. PMID 22513533. PMC 3446796. Bibcode: 2012ISMEJ...6.1926B. https://rdcu.be/cXbPa.
- ↑ Schütt, F. (1895). "2. Teil, Studien über die Zellen der Peridineen". Die Peridineen der Plankton-Expedition. Ergebnisse der Plankton-Expedition der Humboldt-Stiftung. Allgemeiner Theil. pp. 1–170. OCLC 69377189.
- ↑ Smayda, Theodore J. (2002). "Adaptive ecology, growth strategies and the global bloom expansion of dinoflagellates". Journal of Oceanography 58 (2): 281–294. doi:10.1023/A:1015861725470. ISSN 0916-8370.
- ↑ "Chemically-mediated rejection of dinoflagellate prey by the copepods Calanus pacificus and Paracalanus parvus: mechanism, occurrence and significance". Marine Ecology Progress Series 28: 105–120. 1986. doi:10.3354/meps028105. Bibcode: 1986MEPS...28..105H.
- ↑ Faust, M.A.; Gulledge, R.A. (2002). Identifying Harmful Marine Dinoflagellates. Contributions from the United States National Herbarium. 42. Washington, D.C.: Department of Systematic Biology, Botany, National Museum of Natural History. http://www.nmnh.si.edu/botany/projects/dinoflag/. Retrieved 2007-05-18.
- ↑ 75.0 75.1 Lin, S.; Litaker, R.W.; Sunda, W. (2016). "Phosphorus physiological ecology and molecular mechanisms in marine phytoplankton.". Journal of Phycology 52 (1): 10–36. doi:10.1111/jpy.12365. PMID 26987085. Bibcode: 2016JPcgy..52...10L.
- ↑ 76.0 76.1 Zhang, C.; Luo, H.; Huang, L.; Lin, S. (2017). "Molecular mechanism of glucose-6-phosphate utilization in the dinoflagellate Karenia mikimotoi.". Harmful Algae 67: 74–84. doi:10.1016/j.hal.2017.06.006. PMID 28755722.
- ↑ 77.0 77.1 Luo, H.; Lin, X.; Li, L.; Zhang, C.; Lin, L.; Lin, S. (2017). "Transcriptomic and physiological analyses of the dinoflagellate Karenia mikimotoi reveal non-alkaline phosphatase-based molecular machinery of ATP utilization.". Environmental Microbiology 19 (11): 4506–18. doi:10.1111/1462-2920.13899. PMID 28856827.
- ↑ Ecology of Harmful Algae. Ecological Studies. 189. Springer. 2007. ISBN 978-3-5407-4009-4. https://books.google.com/books?id=-707tqiXoZUC. Retrieved 2016-03-05.
- ↑ Castro, Peter; Huber, Michael E. (2010). Marine Biology (8th ed.). McGraw Hill. pp. 95. ISBN 978-0-0711-1302-1.
- ↑ "Chemistries and colors of bioluminescent reactions: a review". Gene 173 (1 Spec No): 5–11. 1996. doi:10.1016/0378-1119(95)00676-1. PMID 8707056.
- ↑ Poupin, J., A.-S. Cussatlegras, and P. Geistdoerfer. 1999. Plancton marin bioluminescent. Rapport scientifique du Laboratoire d'Océanographie de l'École Navale LOEN, Brest, France, 83 pp.
- ↑ Sweeney, B.. Bioluminescence and circadian rhythms. In: Taylor 1987, pp. 269–281
- ↑ 83.0 83.1 83.2 83.3 "Bioluminescence in the sea". Annual Review of Marine Science 2: 443–93. 1 October 2009. doi:10.1146/annurev-marine-120308-081028. PMID 21141672. Bibcode: 2010ARMS....2..443H.
- ↑ "The circadian rhythm of bioluminescence in Pyrocystis is not due to differences in the amount of luciferase: a comparative study of three bioluminescent marine dinoflagellates". J. Phycol. 34 (1): 167–172. February 1998. doi:10.1046/j.1529-8817.1998.340167.x. Bibcode: 1998JPcgy..34..167K.
- ↑ "The circadian bioluminescence rhythm of Gonyaulax is related to daily variations in the number of light-emitting organelles". Journal of Cell Science 95 (Pt 2): 321–8. February 1990. doi:10.1242/jcs.95.2.321. PMID 2196272.
- ↑ Dybas CL (May 2012). "Bright Microbes—Scientists uncover new clues to bioluminescence". Scientific American 360 (5): 19. doi:10.1038/scientificamerican0512-19. Bibcode: 2012SciAm.306e..19D.
- ↑ Castine Kayak (2015). "Castine Kayak Bioluminescent Bay Night Kayak Excursion". visitmaine.com. http://visitmaine.com/deals/castine-kayak-bioluminescent-bay-night-kayak-excursion/?uid=vtm35E9052A846C4F67E.
- ↑ Kennedy Duckett, Maryellen (2015-02-10). "Florida by Water: Experience Bioluminescence". https://www.nationalgeographic.com/travel/florida-land-and-sea/experience-bioluminescence/.
- ↑ Withers, N.. Dinoflagellate sterols. In: Taylor 1987, pp. 316–359
- ↑ "The characteristics and transparent exopolymer particle (TEP) content of marine snow formed from thecate dinoflagellates". J. Plankton Res. 20 (3): 393–406. 1998. doi:10.1093/plankt/20.3.393.
- ↑ 91.0 91.1 91.2 von Stosch HA (1973). "Observations on vegetative reproduction and sexual life cycles of two freshwater dinoflagellates, Gymnodinium pseudopalustre Schiller and Woloszynskia apiculata sp. nov.". British Phycological Journal 8 (2): 105–34. doi:10.1080/00071617300650141. http://www.tandfonline.com/toc/tejp19/8/2. Retrieved 2014-05-13.
- ↑ Pfiester; Anderson (1987). "Ch. 14". in Taylor, F.J.R.. The Biology of dinoflagellates. Botanical monographs. 21. Blackwell Scientific. ISBN 978-0-6320-0915-2. https://archive.org/details/biologyofdinofla0000unse.
- ↑ "DNA Damage Response Pathways in Dinoflagellates". Microorganisms 7 (7): 191. July 2019. doi:10.3390/microorganisms7070191. PMID 31284474.
- ↑ 94.0 94.1 94.2 94.3 94.4 94.5 94.6 94.7 Bravo, Isabel; Figueroa, Rosa (2014). "Towards an Ecological Understanding of Dinoflagellate Cyst Functions". Microorganisms 2 (1): 11–32. doi:10.3390/microorganisms2010011. PMID 27694774. 50px Material was copied from this source, which is available under a Creative Commons Attribution 3.0 International License .
- ↑ 95.0 95.1 Cavalier-Smith, T. (2002). "Origins of the machinery of recombination and sex". Heredity 88 (2): 125–141. doi:10.1038/sj.hdy.6800034. PMID 11932771.
- ↑ Wagmann, Kristen; Hautekèete, Nina-Coralie; Piquot, Yves; Meunier, Cécile; Schmitt, S. Eric; Van Dijk, Henk (2012). "Seed dormancy distribution: Explanatory ecological factors". Annals of Botany 110 (6): 1205–1219. doi:10.1093/aob/mcs194. PMID 22952378.
- ↑ Debieu, Marilyne; Tang, Chunlao; Stich, Benjamin; Sikosek, Tobias; Effgen, Sigi; Josephs, Emily; Schmitt, Johanna; Nordborg, Magnus et al. (2013). "Co-Variation between Seed Dormancy, Growth Rate and Flowering Time Changes with Latitude in Arabidopsis thaliana". PLOS ONE 8 (5): e61075. doi:10.1371/journal.pone.0061075. PMID 23717385. Bibcode: 2013PLoSO...861075D.
- ↑ Reinsch, P.F. (1905) "Die palinosphärien, ein mikroskopischer vegetabile organismus in der mukronatenkreide". ..Cent. Miner. Geol. Palaeontol..., 402–407.
- ↑ Loeblich, A.R.; Loeblich, L.A. (2012). "Dinoflagellate cysts". in Spector, D.L.. Dinoflagellates. Academic Press. pp. 444–474. ISBN 978-0-323-13813-0. https://books.google.com/books?id=qCcgFximE8oC&pg=PA443.
- ↑ Lenormand, Thomas; Otto, Sarah P. (2000). "The Evolution of Recombination in a Heterogeneous Environment". Genetics 156: 423–438. doi:10.1093/genetics/156.1.423. PMID 10978305.
- ↑ Lee, Soo Chan; Ni, Min; Li, Wenjun; Shertz, Cecelia; Heitman, Joseph (2010). "The Evolution of Sex: A Perspective from the Fungal Kingdom". Microbiology and Molecular Biology Reviews 74 (2): 298–340. doi:10.1128/MMBR.00005-10. PMID 20508251.
- ↑ Anderson, Donald Mark; Wall, David (1978). "Potential Importance of Benthic Cysts of Gonyaulax Tamarensis and G. Excavata in Initiating Toxic Dinoflagellate Blooms1, 2, 3". Journal of Phycology 14 (2): 224–234. doi:10.1111/j.1529-8817.1978.tb02452.x. Bibcode: 1978JPcgy..14..224A.
- ↑ Uchida, Takuji; Yoshikawa, Koji; Arai, Akemi; Arai, Shogo (1991). "Life-Cycle and Its Control of Sargassum muticum (Phaeophyta) in Batch Cultures". Nippon Suisan Gakkaishi 57 (12): 2249–2253. doi:10.2331/suisan.57.2249.
- ↑ Uchida, Takuji; Matsuyama, Yukihiko; Yamaguchi, Mineo; Honjo, Tsuneo (1996). "The life cycle of Gyrodinium instriatum (Dinophyceae) in culture". Phycological Research 44 (3): 119–123. doi:10.1111/j.1440-1835.1996.tb00040.x.
- ↑ Figueroa, Rosa Isabel; Bravo, Isabel (2005). "Sexual Reproduction and Two Different Encystment Strategies of Lingulodinium Polyedrum (Dinophyceae) in Culture1". Journal of Phycology 41 (2): 370–379. doi:10.1111/j.1529-8817.2005.04150.x. Bibcode: 2005JPcgy..41..370F.
- ↑ Figueroa, Rosa Isabel; Bravo, Isabel; Garcés, Esther (2006). "Multiple Routes of Sexuality in Alexandrium Taylori (Dinophyceae) in Culture". Journal of Phycology 42 (5): 1028–1039. doi:10.1111/j.1529-8817.2006.00262.x. Bibcode: 2006JPcgy..42.1028F.
- ↑ Kremp, Anke; Parrow, Matthew W. (2006). "Evidence for Asexual Resting Cysts in the Life Cycle of the Marine Peridinoid Dinoflagellate, Scrippsiella Hangoei1". Journal of Phycology 42 (2): 400–409. doi:10.1111/j.1529-8817.2006.00205.x. Bibcode: 2006JPcgy..42..400K.
- ↑ Parrow, Matthew W.; Burkholder, Joann M. (2004). "The Sexual Life Cycles of Pfiesteria Piscicida and Cryptoperidiniopsoids (Dinophyceae)1". Journal of Phycology 40 (4): 664–673. doi:10.1111/j.1529-8817.2004.03202.x. Bibcode: 2004JPcgy..40..664P.
- ↑ Beam, Carl A.; Himes, Marion (2012-12-02). Ch. 8: Dinoflagellate genetics. Academic Press. pp. 263–298. ISBN 978-0-3231-3813-0. https://books.google.com/books?id=qCcgFximE8oC&pg=PA263. Retrieved 2016-03-05. In Spector 1984
- ↑ "The genome of Symbiodinium kawagutii illuminates dinoflagellate gene expression and coral symbiosis.". Science 350 (6261): 691–694. 2015. doi:10.1126/science.aad0408. PMID 26542574.
- ↑ "Comparative genomics reveals two major bouts of gene retroposition coinciding with crucial periods of Symbiodinium evolution.". Genome Biology and Evolution 9 (8): 2037–2047. 2017. doi:10.1093/gbe/evx144. PMID 28903461.
- ↑ "Genome size-dependent PCNA gene copy number in dinoflagellates and molecular evidence of retroposition as a major evolutionary mechanism.". Journal of Phycology 55 (1): 37–46. 2018. doi:10.1111/jpy.12815. PMID 30468510.
- ↑ "Understanding relationship break-ups to protect the reef". https://www.sciencedaily.com/releases/2019/05/190513100546.htm.
- ↑ "The mitochondrial genome and transcriptome of the basal dinoflagellate Hematodinium sp.: character evolution within the highly derived mitochondrial genomes of dinoflagellates". Genome Biology and Evolution 4 (1): 59–72. 2012. doi:10.1093/gbe/evr122. PMID 22113794.
- ↑ John, Uwe; Lu, Yameng; Wohlrab, Sylke; Groth, Marco; Janouškovec, Jan; Kohli, Gurjeet S.; Mark, Felix C.; Bickmeyer, Ulf et al. (April 2019). "An aerobic eukaryotic parasite with functional mitochondria that likely lacks a mitochondrial genome" (in en). Science Advances 5 (4): eaav1110. doi:10.1126/sciadv.aav1110. ISSN 2375-2548. PMID 31032404. Bibcode: 2019SciA....5.1110J.
- ↑ "Widespread and extensive editing of mitochondrial mRNAs in dinoflagellates.". Journal of Molecular Biology 320 (4): 727–739. 2002. doi:10.1016/S0022-2836(02)00468-0. PMID 12095251.
- ↑ Smith, H., ed (2008). "RNA editing in dinoflagellates and its implications for the evolutionary history of the editing machinery". RNA and DNA editing: Molecular Mechanisms and Their Integration into Biological Systems. Wiley. pp. 280–309. ISBN 978-0-470-26225-2. https://books.google.com/books?id=DyFZFqG-d0QC&pg=PA280.
- ↑ Imanian, B.; Pombert, J. F.; Dorrell, R. G.; Burki, F.; Keeling, P. J. (2012). "The endosymbiotic origin, diversification and fate of plastids". PLOS ONE 7 (8): e43763. doi:10.1371/journal.pone.0043763. PMID 22916303. Bibcode: 2012PLoSO...743763I.
- ↑ Das, Biplab (2019). "Marine parasite survives without key genes". Nature Middle East. doi:10.1038/nmiddleeast.2019.63.
- ↑ "Plastid-derived single gene minicircles of the dinoflagellate Ceratium horridum are localized in the nucleus". Molecular Biology and Evolution 21 (7): 1318–22. July 2004. doi:10.1093/molbev/msh127. PMID 15034134.
- ↑ "Evaluating the ribosomal internal transcribed spacer (ITS) as a candidate dinoflagellate barcode marker". PLOS ONE 7 (8): e42780. 2012. doi:10.1371/journal.pone.0042780. PMID 22916158. Bibcode: 2012PLoSO...742780S.
- ↑ "Recognizing dinoflagellate species using ITS rDNA sequences". J. Phycol. 43 (2): 344–355. April 2007. doi:10.1111/j.1529-8817.2007.00320.x. Bibcode: 2007JPcgy..43..344W.
- ↑ Wang, Lu; Zhuang, Yunyun; Zhang, Huan; Lin, Xin; Lin, Senjie (2014). "DNA barcoding species in Alexandrium tamarense complex using ITS and proposing designation of five species.". Harmful Algae 31: 100–113. doi:10.1016/j.hal.2013.10.013. PMID 28040099.
- ↑ Stephens, Timothy G.; Ragan, MA; Bhattacharya, D; Chan, CX (Nov 21, 2018). "Core genes in diverse dinoflagellate lineages include a wealth of conserved dark genes with unknown functions". Scientific Reports 8 (1): 17175. doi:10.1038/s41598-018-35620-z. PMID 30464192. Bibcode: 2018NatSR...817175S.
- ↑ 125.0 125.1 "Fossil dinoflagellate diversity, originations, and extinctions and their significance". Can. J. Bot. 74 (11): 1687–94. 1996. doi:10.1139/b96-205. ISSN 0008-4026.
- ↑ "Biogeochemical evidence for dinoflagellate ancestors in the Early Cambrian". Science 281 (5380): 1168–70. August 1998. doi:10.1126/science.281.5380.1168. PMID 9712575. Bibcode: 1998Sci...281.1168M.
- ↑ "Chemostratigraphic reconstruction of biofacies: molecular evidence linking cyst-forming dinoflagellates with Pre-Triassic ancestors". Geology 24 (2): 159–162. February 1996. doi:10.1130/0091-7613(1996)024<0159:CROBME>2.3.CO;2. Bibcode: 1996Geo....24..159M.
- ↑ "Affinities of early Cambrian acritarchs studied by using microscopy, fluorescence flow cytometry and biomarkers". Rev. Palaeobot. Palynol. 108 (1–2): 37–53. January 2000. doi:10.1016/S0034-6667(99)00032-9. Bibcode: 2000RPaPa.108...37T.
- ↑ 129.0 129.1 129.2 Fensome, Robert (8 June 2022). "Dinoflagellates". https://palynology.org/what-is-palynology/palynomorphs/dinoflagellates/?preview_id=40&preview_nonce=48130c1f50&_thumbnail_id=-1&preview=true.[yes|permanent dead link|dead link}}]
- ↑ Fensome, R. A.; MacRae, R. A.; Moldowan, J. M.; Taylor, F. J. R.; Williams, G. L. (1996). "The Early Mesozoic Radiation of Dinoflagellates". Paleobiology 22 (3): 329–338. doi:10.1017/S0094837300016316. ISSN 0094-8373. Bibcode: 1996Pbio...22..329F.
- ↑ Chacón, J.; Gottschling, M. (2020). "Dawn of the dinophytes: A first attempt to date origin and diversification of harmful algae". Harmful Algae 97: 101871. doi:10.1016/j.hal.2020.101871. PMID 32732051.
- ↑ Saldarriaga J; Taylor MFJR; Cavalier-Smith T; Menden-Deuer S; Keeling PJ (2004). "Molecular data and the evolutionary history of dinoflagellates". Eur J Protistol 40 (1): 85–111. doi:10.1016/j.ejop.2003.11.003.
- ↑ "Dinoflagellate phylogeny revisited: reconciling morphological and molecular based phylogenies". Grana 38 (2–3): 66–80. 1999. doi:10.1080/00173139908559216. Bibcode: 1999Grana..38...66F.
- ↑ "Phylogenetic placement of environmental sequences using taxonomically reliable databases helps to rigorously assess dinophyte biodiversity in Bavarian lakes (Germany)". Freshwater Biology 65 (2): 193–208. 2020. doi:10.1111/fwb.13413. Bibcode: 2020FrBio..65..193G.
- ↑ "The phylogenetic position of Amoebophrya sp. infecting Gymnodinium sanguineum". The Journal of Eukaryotic Microbiology 46 (2): 194–7. 1999. doi:10.1111/j.1550-7408.1999.tb04603.x. PMID 10361739.
"Multiple strains of the parasitic dinoflagellate Amoebophrya exist in Chesapeake Bay". The Journal of Eukaryotic Microbiology 49 (6): 469–74. 2002. doi:10.1111/j.1550-7408.2002.tb00230.x. PMID 12503682. - ↑ "Unexpected diversity of small eukaryotes in deep-sea Antarctic plankton". Nature 409 (6820): 603–7. February 2001. doi:10.1038/35054537. PMID 11214316. Bibcode: 2001Natur.409..603L.
- ↑ "Oceanic 18S rDNA sequences from picoplankton reveal unsuspected eukaryotic diversity". Nature 409 (6820): 607–10. February 2001. doi:10.1038/35054541. PMID 11214317. Bibcode: 2001Natur.409..607M.
- ↑ "Dinoflagellate nuclear SSU rRNA phylogeny suggests multiple plastid losses and replacements". Journal of Molecular Evolution 53 (3): 204–13. September 2001. doi:10.1007/s002390010210. PMID 11523007. Bibcode: 2001JMolE..53..204S.
- ↑ "A common red algal origin of the apicomplexan, dinoflagellate, and heterokont plastids". Proceedings of the National Academy of Sciences of the United States of America 107 (24): 10949–54. June 2010. doi:10.1073/pnas.1003335107. PMID 20534454. Bibcode: 2010PNAS..10710949J.
- ↑ "Endosymbiosis undone by stepwise elimination of the plastid in a parasitic dinoflagellate". Proceedings of the National Academy of Sciences of the United States of America 112 (18): 5767–72. May 2015. doi:10.1073/pnas.1423400112. PMID 25902514. Bibcode: 2015PNAS..112.5767G.
- ↑ Dorrell, R. G.; Howe, C. J. (2015). "Integration of plastids with their hosts: Lessons learned from dinoflagellates". Proceedings of the National Academy of Sciences of the United States of America 112 (33): 10247–10254. doi:10.1073/pnas.1421380112. PMID 25995366. Bibcode: 2015PNAS..11210247D.
- ↑ Tørresen, Ole Kristian (2010). Investigating the plastid replacement in the green dinoflagellate Lepidodinium chlorophorum (Master's thesis). University of Oslo. hdl:10852/11559.
- ↑ Garcia-Cuetos, L.; Moestrup, Ø.; Hansen, P.J.; Daugbjerg, N. (2010). "The toxic dinoflagellate Dinophysis acuminata harbors permanent chloroplasts of cryptomonad origin, not kleptochloroplasts". Harmful Algae 9 (1): 25–38. doi:10.1016/j.hal.2009.07.002.
- ↑ Kretschmann, J.; Žerdoner Čalasan, A.; Gottschling, M. (2018). "Molecular phylogenetics of dinophytes harboring diatoms as endosymbionts (Kryptoperidiniaceae, Peridiniales), with evolutionary interpretations and a focus on the identity of Durinskia oculata from Prague". Molecular Phylogenetics and Evolution 118: 392–402. doi:10.1016/j.ympev.2017.10.011. PMID 29066288.
- ↑ Imanian, B.; Keeling, P. J. (2007). "The dinoflagellates Durinskia baltica and Kryptoperidinium foliaceum retain functionally overlapping mitochondria from two evolutionarily distinct lineages". BMC Evolutionary Biology 7 (1): 172. doi:10.1186/1471-2148-7-172. PMID 17892581. Bibcode: 2007BMCEE...7..172I..
- ↑ Gast, R. J.; Moran, D. M.; Dennett, M. R.; Caron, D. A. (2007). "Kleptoplasty in an Antarctic dinoflagellate: Caught in evolutionary transition?". Environmental Microbiology 9 (1): 39–45. doi:10.1111/j.1462-2920.2006.01109.x. PMID 17227410. Bibcode: 2007EnvMi...9...39G.
- ↑ "On dinoflagellate evolution". Bio Systems 13 (1–2): 65–108. 1980. doi:10.1016/0303-2647(80)90006-4. PMID 7002229. Bibcode: 1980BiSys..13...65T.
- ↑ "They are young, and they are many: Dating freshwater lineages in unicellular dinophytes". Environmental Microbiology 21 (11): 4125–4135. 2019. doi:10.1111/1462-2920.14766. PMID 31369197. Bibcode: 2019EnvMi..21.4125Z.
- ↑ Kim, M.; Choi, D. H.; Park, M. G. (2021). "Cyanobiont genetic diversity and host specificity of cyanobiont-bearing dinoflagellate Ornithocercus in temperate coastal waters". Scientific Reports 11 (1): 9458. doi:10.1038/s41598-021-89072-z. PMID 33947914. Bibcode: 2021NatSR..11.9458K.
- ↑ Nakayama, Takuro; Nomura, Mami; Takano, Yoshihito; Tanifuji, Goro; Shiba, Kogiku; Inaba, Kazuo; Inagaki, Yuji; Kawata, Masakado (2019). "Single-cell genomics unveiled a cryptic cyanobacterial lineage with a worldwide distribution hidden by a dinoflagellate host". Proceedings of the National Academy of Sciences 116 (32): 15973–15978. doi:10.1073/pnas.1902538116. PMID 31235587. Bibcode: 2019PNAS..11615973N.
- ↑ "Living fossils in the Indo-Pacific warm pool: A refuge for thermophilic dinoflagellates during glaciations". Geology 42 (6): 531–534. 2014. doi:10.1130/G35456.1. Bibcode: 2014Geo....42..531M.
- ↑ Montresor, M.; Janofske, D.; Willems, H. (1997). "The cyst-theca relationship in Calciodinellum operosum emend. (Peridiniales, Dinophyceae) and a new approach for the study of calcareous cysts". Journal of Phycology 33 (1): 122–131. doi:10.1111/j.0022-3646.1997.00122.x. Bibcode: 1997JPcgy..33..122M.
- ↑ Gu, H.; Kirsch, M.; Zinßmeister, C.; Söhner, S.; Meier, K.J.S.; Liu, T.; Gottschling, M. (2013). "Waking the dead: Morphological and molecular characterization of extant †Posoniella tricarinelloides (Thoracosphaeraceae, Dinophyceae)". Protist 164 (5): 583–597. doi:10.1016/j.protis.2013.06.001. PMID 23850812.
Bibliography
- Spector, D.L. (1984). Dinoflagellates. Academic Press. ISBN 978-0-3231-3813-0. https://books.google.com/books?id=qCcgFximE8oC. Retrieved 2016-03-05.
- Taylor, F.J.R. (1987). The Biology of Dinoflagellates. Botanical monographs. 21. Blackwell Scientific. ISBN 978-0-6320-0915-2. https://archive.org/details/biologyofdinofla0000unse.
External links
- International Society for the Study of Harmful Algae
- Classic dinoflagellate monographs
- Japanese dinoflagellate site
- Noctiluca scintillans—Guide to the Marine Zooplankton of south eastern Australia, Tasmanian Aquaculture & Fisheries Institute
- Tree of Life Dinoflagellates
- Centre of Excellence for Dinophyte Taxonomy CEDiT
- Dinoflagellates
- Judson O (5 January 2010). "A Tale of Two Flagella". New York Times. http://opinionator.blogs.nytimes.com/2010/01/05/a-tale-of-two-flagella/.
Wikidata ☰ {{{from}}} entry
![]() | Original source: https://en.wikipedia.org/wiki/Dinoflagellate.
Read more |