Earth:Late Pleistocene extinctions
{{Multiple issues|
![]() | This page may be unbalanced towards certain viewpoints. (January 2023) |

The Late Pleistocene to the beginning of the Holocene saw numerous extinctions of predominantly megafaunal (large) animal species (the Pleistocene megafauna), which resulted in a collapse in faunal density and diversity across the globe.[1] The extinctions during the Late Pleistocene are differentiated from previous extinctions by the widespread absence of ecological succession to replace these extinct megafaunal species,[2] and the regime shift of previously established faunal relationships and habitats as a consequence. The timing and severity of the extinctions varied by region and are thought to have been driven by varying combinations of human and climatic factors.[2] Human impact on megafauna populations is thought to have been driven by hunting ("overkill"),[3][4] as well as possibly environmental alteration.[5] The relative importance of human vs climatic factors in the extinctions has been the subject of long-running controversy.[2]
Major extinctions occurred in Australia-New Guinea (Sahul) beginning approximately 50,000 years ago and in the Americas about 13,000 years ago, coinciding in time with the early human migrations into these regions.[6] Extinctions in northern Eurasia were staggered over tens of thousands of years between 50,000 and 10,000 years ago,[1] while extinctions in the Americas were virtually simultaneous, spanning only 3000 years at most.[3][7] Overall, during Late Pleistocene about 65% of all megafaunal species worldwide became extinct,[8] rising to 72% in North America, 83% in South America and 88% in Australia, with Africa, South Asia and Southeast Asia having much lower extinctions than other regions.[9]
Extinctions by biogeographic realm
Summary
Biogeographic realm | Giants (over 1,000 kg) |
Very large (400–1,000 kg) |
Large (150–400 kg) |
Moderately large (50–150 kg) |
Medium (10–50 kg) |
Total | Regions included | ||||||||||||
---|---|---|---|---|---|---|---|---|---|---|---|---|---|---|---|---|---|---|---|
Start | Loss | % | Start | Loss | % | Start | Loss | % | Start | Loss | % | Start | Loss | % | Start | Loss | % | ||
Afrotropic | 6 | −1 | 16.6% | 4 | −1 | 25% | 25 | −3 | 12% | 32 | 0 | 0% | 69 | −2 | 2.9% | 136 | -7 | 5.1% | Trans-Saharan Africa and Arabia |
Indomalaya | 5 | −2 | 40% | 6 | −1 | 16.7% | 10 | −1 | 10% | 20 | −3 | 15% | 56 | −1 | 1.8% | 97 | -8 | 8.2% | Indian subcontinent, Southeast Asia, and southern China |
Palearctic | 8 | −8 | 100% | 10 | −5 | 50% | 14 | −5 | 35.7% | 23 | −3 | 15% | 41 | −1 | 2.4% | 96 | -22 | 22.9% | Eurasia and North Africa |
Nearctic | 5 | −5 | 100% | 10 | −8 | 80% | 26 | −22 | 84.6% | 20 | −13 | 65% | 25 | −9 | 36% | 86 | -57 | 66% | North America |
Neotropic | 9 | −9 | 100% | 12 | −12 | 100% | 17 | −14 | 82% | 20 | −11 | 55% | 35 | −5 | 14.3% | 93 | -51 | 54% | South America, Central America, South Florida, and the Caribbean |
Australasia | 4 | −4 | 100% | 5 | −5 | 100% | 6 | −6 | 100% | 16 | −13 | 81.2% | 25 | −10 | 40% | 56 | -38 | 67% | Australia , New Guinea, New Zealand, and neighbouring islands. |
Global | 33 | −26 | 78.8% | 46 | −31 | 67.4% | 86 | −47 | 54.7% | 113 | −41 | 36.3% | 215 | −23 | 10.1% | 493 | -168 | 34% |
Introduction
The Late Pleistocene saw the extinction of many mammals weighing more than 40 kilograms (88 lb). The proportion of megafauna extinctions is progressively larger the further the human migratory distance from Africa, with the highest extinction rates in Australia, and North and South America.
The increased extent of extinction mirrors the migration pattern of modern humans: the further away from Africa, the more recently humans inhabited the area, the less time those environments (including its megafauna) had to become accustomed to humans (and vice versa).
There are two main hypotheses to explain this extinction:
- Climate change associated with the advance and retreat of major ice caps or ice sheets causing reduction in favorable habitat.
- Human hunting causing attrition of megafauna populations, commonly known as "overkill".[11]
There are some inconsistencies between the current available data and the prehistoric overkill hypothesis. For instance, there are ambiguities around the timing of Australian megafauna extinctions.[11] Evidence supporting the prehistoric overkill hypothesis includes the persistence of megafauna on some islands for millennia past the disappearance of their continental cousins. For instance, ground sloths survived on the Antilles long after North and South American ground sloths were extinct, woolly mammoths died out on remote Wrangel Island 6,000 years after their extinction on the mainland, while Steller's sea cows persisted off the isolated and uninhabited Commander Islands for thousands of years after they had vanished from the continental shores of the north Pacific.[12] The later disappearance of these island species correlates with the later colonization of these islands by humans.
The original debates as to whether human arrival times or climate change constituted the primary cause of megafaunal extinctions necessarily were based on paleontological evidence coupled with geological dating techniques. Recently, genetic analyses of surviving megafaunal populations have contributed new evidence, leading to the conclusion: "The inability of climate to predict the observed population decline of megafauna, especially during the past 75,000 years, implies that human impact became the main driver of megafauna dynamics around this date."[13]
An alternative hypothesis to the theory of human responsibility is climate change associated with the last glacial period. Discredited explanations include the Younger Dryas impact hypothesis[14] and Tollmann's hypothesis that extinctions resulted from bolide impacts.
Recent research indicates that each species responded differently to environmental changes, and no one factor by itself explains the large variety of extinctions. The causes may involve the interplay of climate change, competition between species, unstable population dynamics, and human predation.[15]
Africa
Although Africa was one of the least affected regions, the region still suffered extinctions, particularly around the Late Pleistocene-Holocene transition. These extinctions were likely predominantly climatically driven by changes to grassland habitats.[16]
- Ungulates
- Even-Toed Ungulates
- Odd-toed Ungulates
- Rhinoceros (Rhinocerotidae).
- Stephanorhinus hemitoechus (North Africa)
- Ceratotherium mauritanicum
- Wild Equus spp.
- Caballine horses
- Equus algericus (North Africa)
- Subgenus Asinus (asses)
- Equus melkiensis (North Africa)
- Zebras
- Equus capensis
- Saharan zebra[17] (Equus mauritanicus)
- Caballine horses
- Rhinoceros (Rhinocerotidae).
- Proboscidea
- Elephantidae (elephants)
- Palaeoloxodon iolensis? (other authors suggest that this taxon went extinct at the end of the Middle Pleistocene)
- Elephantidae (elephants)
- Rodentia
- Paraethomys filfilae?
South Asia, Southeast Asia and East Asia
The timing of extinctions on the Indian subcontinent is uncertain due to a lack of reliable dating.[18] Similar issues have been reported for Chinese sites, though there is no evidence for any of the megafaunal taxa having survived into the Holocene in that region.[19] Extinctions in Southeast Asia and South China have been proposed to be the result of environmental shift from open to closed forested habitats.[20]
- Ungulates
- Even-Toed Ungulates
- Several Bovidae spp.
- Bos palaesondaicus (ancestor to the banteng)
- Bison hanaizumiensis[21][22][23]
- Cebu tamaraw (Bubalus cebuensis)
- Bubalus grovesi[24]
- Bubalus wansijocki
- Short-horned water buffalo (Bubalus mephistopheles)
- Bubalus palaeokerabau
- Cervidae
- Sinomegaceros spp. (including Sinomegaceros yabei in Japan, and Sinomegaceros ordosianus in China).[25]
- Hippopotamidae
- Hexaprotodon (Indian subcontinent)[26]
- Several Bovidae spp.
- Odd-toed Ungulates
- Equus spp.
- Equus namadicus (Indian subcontinent)
- Yunnan horse (Equus yunanensis)
- Giant tapir (Tapirus augustus, Southeast Asia and Southern China)
- Merck's rhinoceros (Stephanorhinus kirchbergensis Eastern Asia)
- Equus spp.
- Even-Toed Ungulates
- Pholidota
- Giant Asian pangolin (Manis palaeojavanica)
- Carnivora
- Caniformia
- Arctoidea
- Bears
- Ailuropoda baconi (ancestor to the giant panda)
- Bears
- Arctoidea
- Caniformia
- Afrotheria
- Afroinsectiphilia
- Orycteropodidae/Tubulidentata
- Aardvark (Orycteropus afer; extirpated in South Asia circa 13,000 BCE)[27][28]
- Orycteropodidae/Tubulidentata
- Paenungulata
- Tethytheria
- Proboscideans
- Stegodontidae
- Stegodon spp. (including Stegodon florensis on Flores, Stegodon orientalis in East and Southeast Asia, and Stegodon sp. in the Indian subcontinent)
- Palaeoloxodon spp.
- Palaeoloxodon namadicus (Indian subcontinent, possibly also Southeast Asia)
- Naumann's elephant (Japan)
- Palaeoloxodon huaihoensis (China)
- Stegodontidae
- Proboscideans
- Tethytheria
- Afroinsectiphilia
- Birds
- Japanese flightless duck (Shiriyanetta hasegawai)[29]
- Leptoptilos robustus
- Reptiles
- Primates
- Several simian (Simiiformes) spp.
- Pongo (orangutans)
- Pongo weidenreichi (South China)
- Various Homo spp. (archaic humans)
- Homo erectus (Java)
- Homo floresiensis (Flores)
- Homo luzonensis (Luzon, Philippines)
- Denisovans (Homo sp.)
- Pongo (orangutans)
- Several simian (Simiiformes) spp.
Europe and northern Asia
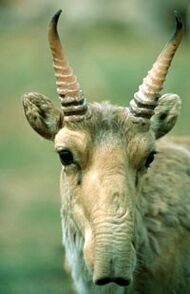

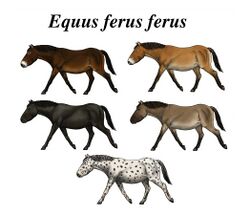
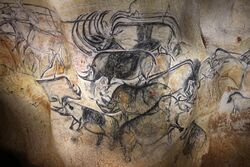

The Palearctic realm spans the entirety of the European continent and stretches into northern Asia, through the Caucasus and central Asia to northern China, Siberia and Beringia. Extinctions were more severe in Northern Eurasia than in Africa or South and Southeast Asia. These extinctions were staggered over tens of thousands of years, spanning from around 50,000 years Before Present (BP) to around 10,000 years BP, with temperate adapted species like the straight-tusked elephant and the narrow-nosed rhinoceros generally going extinct earlier than cold adapted species like the woolly mammoth and woolly rhinoceros. Climate change has been considered a probable major factor in the extinctions, possibly in combination with human hunting.[1]
- Ungulates
- Even-Toed Hoofed Mammals
- Various Bovidae spp.
- Steppe bison (Bison priscus)
- Baikal yak (Bos baikalensis)[30]
- European water buffalo (Bubalus murrensis)
- European tahr (Hemitragus cedrensis)[31][32]
- Giant muskox (Praeovibos priscus)[33]
- Northern saiga antelope (Saiga borealis)[34]
- Twisted-horned antelope (Spirocerus kiakhtensis)[35][36]
- Goat-horned antelope (Parabubalis capricornis)[35][36]
- Various deer (Cervidae) spp.
- Broad-fronted moose (Cervalces latifrons)
- Giant deer (Megaloceros giganteus)
- Praemegaceros savini
- Cretan deer (Candiacervus)
- Haploidoceros mediterraneus[37][38]
- All native Hippopotamus spp.[39]
- Hippopotamus amphibius (European range)
- Maltese dwarf hippopotamus (Hippopotamus melitensis)
- Cyprus dwarf hippopotamus (Hippopotamus minor)
- Sicilian dwarf hippopotamus (Hippopotamus pentlandi)
- Camelus knoblochi[40] and other Camelus spp.
- Various Bovidae spp.
- Odd-Toed Hoofed Mammals
- Various Equus spp. e.g.
- Wild horse (Equus ferus ssp.)
- Equus cf. gallicus[41][42]
- European wild ass (Equus hydruntinus)
- Equus cf. latipes[36][41][43]
- Equus lenensis[36][44]
- Equus cf. uralensis[41]
- All native Rhinoceros (Rhinocerotidae) spp.
- Elasmotherium
- Woolly rhinoceros (Coelodonta antiquitatis)
- Stephanorhinus spp.
- Merck's rhinoceros (Stephanorhinus kirchbergensis)
- Narrow-nosed rhinoceros (Stephanorhinus hemiotoechus)
- Various Equus spp. e.g.
- Even-Toed Hoofed Mammals
- Carnivora
- Caniformia
- Canidae
- Caninae
- Wolves
- Dholes
- European dhole (Cuon alpinus europaeus)
- Sardinian dhole (Cynotherium sardous)
- Caninae
- Arctoidea
- Various Ursus spp.
- Steppe brown bear (Ursus arctos "priscus")[46]
- Gamssulzen cave bear (Ursus ingressus)[47]
- Pleistocene small cave bear (Ursus rossicus)
- Cave bear (Ursus spelaeus)
- Giant polar bear (Ursus maritimus tyrannus)
- Musteloidea
- Mustelidae
- Several otter (Lutrinae) spp.
- Robust Pleistocene European otter (Cyrnaonyx)
- Algarolutra
- Sardinian giant otter (Megalenhydris barbaricina)
- Sardinian dwarf otter (Sardolutra)
- Cretan otter (Lutrogale cretensis)
- Several otter (Lutrinae) spp.
- Mustelidae
- Various Ursus spp.
- Canidae
- Feliformia
- Various Felidae spp.
- Herpestoidea
- Cave hyena (Crocuta crocuta spelaea)
- hyaena prisca
- Caniformia
- All native Elephant (Elephantidae) spp.
- Mammoths
- Woolly mammoth (Mammuthus primigenius)
- Dwarf Sardinian mammoth (Mammuthus lamarmorai)
- Straight-tusked elephant (Palaeoloxodon antiquus) (Europe)
- Dwarf elephant
- Palaeoloxodon creutzburgi (Crete)
- Cyprus dwarf elephant (Palaeoloxodon cypriotes)
- Palaeoloxodon mnaidriensis (Sicily)
- Mammoths
- Rodents
- Allocricetus bursae
- Cricetus major (alternatively Cricetus cricetus major)
- Dicrostonyx gulielmi (ancestor to the Arctic lemming)
- Giant Eurasian porcupine (Hystrix refossa)
- Leithia spp. (Maltese and Sicilian giant dormouse)[49]
- Marmota paleocaucasica
- Microtus grafi
- Mimomys spp.
- M. pyrenaicus
- M. chandolensis
- Pliomys lenki
- Spermophilus citelloides
- Spermophilus severskensis
- Spermophilus superciliosus
- Trogontherium cuiveri
- Lagomorpha
- Lepus tanaiticus (alternatively Lepus timidus tanaiticus)
- Pika (Ochotona) spp. e.g.
- Giant pika (Ochotona whartoni)
- Tonomochota spp.
- T. khasanensis
- T. sikhotana
- T. major
- Birds
- Asian ostrich (Struthio asiaticus)
- Yakutian goose (Anser djuktaiensis)
- Various European crane spp. (Genus Grus)
- Grus primigenia
- Grus melitensis
- Cretan owl (Athene cretensis)
- Primates
- Homo
- Denisovans (Homo sp.)
- Neanderthals (Homo (sapiens) neanderthalensis; survived until about 40,000 years ago on the Iberian peninsula)
- Homo
- Reptiles
North America
- Younger Dryas cooling period, as well as the emergence of the hunter-gatherer Clovis culture. The relative importance of human and climactic factors in the North American extinctions has been the subject of significant controversy. Extinctions totalled around 35 genera.[3] The radiocarbon record for North America south of the Alaska-Yukon region has been described as "inadequate" to construct a reliable chronology.[50] Extinctions in North America were concentrated at the end of the Late Pleistocene, around 13,800–11,400 years Before Present, which were coincident with the onset of the
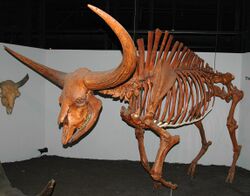
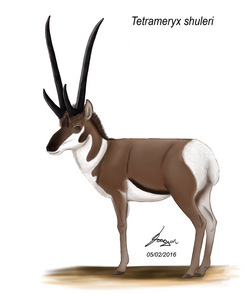
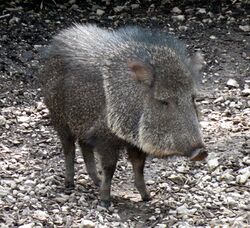
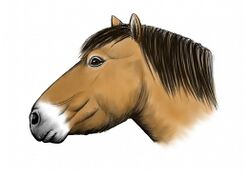
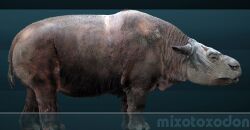
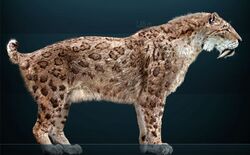
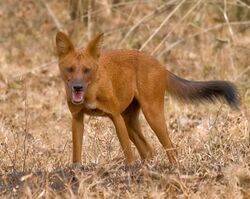
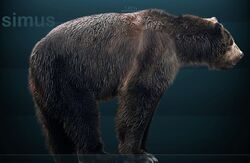
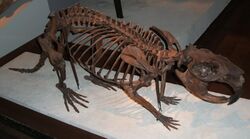
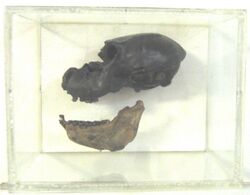
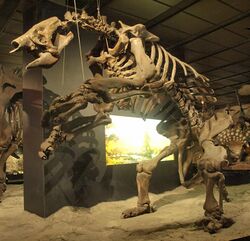

North American extinctions (noted as herbivores (H) or carnivores (C)) included:
- Ungulates
- Even-Toed Hoofed Mammals
- Various Bovidae spp.
- Most forms of Pleistocene bison (only Bison bison in North America, and Bison bonasus in Eurasia, survived)
- Ancient bison (Bison antiquus) (H)
- Long-horned/Giant bison (Bison latifrons) (H)
- Steppe bison (Bison priscus) (H)
- Bison occidentalis (H)
- Several members of Caprinae (the muskox survived)
- Giant muskox (Praeovibos priscus) (H)
- Shrub-ox (Euceratherium collinum) (H)
- Harlan's muskox (Bootherium bombifrons) (H)
- Soergel's ox (Soergelia mayfieldi) (H)
- Harrington's mountain goat (Oreamnos harringtoni; smaller and more southern distribution than its surviving relative) (H)
- Saiga antelope (Saiga tatarica; extirpated) (H)
- Most forms of Pleistocene bison (only Bison bison in North America, and Bison bonasus in Eurasia, survived)
- Deer
- Stag-moose (Cervalces scotti) (H)
- American mountain deer (Odocoileus lucasi) (H)
- Torontoceros hypnogeos (H)
- Various Antilocapridae genera (pronghorns survived)
- Capromeryx (H)
- Stockoceros (H)
- Tetrameryx (H)
- Pacific pronghorn (Antilocapra pacifica) (H)
- Several peccary (Tayassuidae) spp.
- Flat-headed peccary (Platygonus) (H)
- Long-nosed peccary (Mylohyus) (H)
- Collared peccary (Dicotyles tajacu; extirpated, range semi-recolonised) (H) (Muknalia minimus is a junior synonym)
- Various members of Camelidae
- Western camel (Camelops hesternus) (H)
- Stilt legged llamas (Hemiauchenia ssp.) (H)
- Stout legged llamas (Palaeolama ssp.) (H)
- Various Bovidae spp.
- Odd-Toed Hoofed Mammals
- All native forms of Equidae
- Caballine true horses (Equus cf. ferus) from the Late Pleistocene of North America have historically been assigned to many different species, including Equus fraternus, Equus scotti and Equus lambei, but the taxonomy of these horses is unclear, and many of these species may be synonymous with each other, perhaps only representing a single species.[51][52][53]
- Stilt-legged horse (Haringtonhippus francisci / Equus francisci; (H)
- Tapirs (Tapirus; three species)
- California tapir (Tapirus californicus) (H)
- Merriam's tapir (Tapirus merriami) (H)
- Vero tapir (Tapirus veroensis) (H)
- All native forms of Equidae
- †Order Notoungulata
- Even-Toed Hoofed Mammals
- Carnivora
- Feliformia
- Several Felidae spp.
- Saber-Tooths
- North American saber-toothed cat (Smilodon fatalis) (C)
- North American scimitar cat (Homotherium serum) (C)
- American cheetah (Miracinonyx; not true cheetah)
- Miracinonyx trumani (C)
- Cougar (Puma concolor; megafaunal ecomorph extirpated from North America, South American populations recolonised former range) (C)
- Jaguarundi (Herpailurus yagouaroundi; extirpated, range semi-recolonised) (C)
- Margay (Leopardus weidii; extirpated) (C)
- Ocelot (Leopardus pardalis; extirpated, range marginally recolonised) (C)
- Eurasian lynx (Lynx lynx; extirpated)[56] (C)
- Jaguars
- Pleistocene North American jaguar (Panthera onca augusta; range semi-recolonised by other subspecies) (C)
- North America Jaguar
- Panthera balamoides (dubious, suggested to be a junior synonym of the short faced bear Arctotherium)
- Lions
- American lion (Panthera atrox; endemic to North America after 340,000 BP) (C)
- Cave/steppe lion (Panthera spelaea; present only as far as modern day Yukon) (C)
- Saber-Tooths
- Several Felidae spp.
- Caniformia
- Canidae
- Dire wolf (Aenocyon dirus) (C)
- Pleistocene coyote (Canis latrans orcutti) (C)
- Megafaunal wolf e.g.
- Beringian wolf (Canis lupus ssp.) (C)
- Dhole (Cuon alpinus; extirpated) (C)
- Protocyon troglodytes[57] (C)
- Arctoidea
- Musteloidea
- Mephitidae
- Short-faced skunk (Brachyprotoma obtusata)[58] (C)
- Mustelidae
- Steppe polecat (Mustela eversmanii; extirpated)[59] (C)
- Mephitidae
- Various bear (Ursidae) spp.
- Arctodus simus (C)
- Florida spectacled bear (Tremarctos floridanus) (C)
- South American short-faced bear (Arctotherium wingei)[60][57] (C)
- Giant polar bear (Ursus maritimus tyrannus; a possible inhabitant) (C)
- Musteloidea
- Canidae
- Feliformia
- Afrotheria
- Afroinsectiphilia
- Orycteropodidae/Tubulidentata
- Giant anteater (Myrmecophaga tridactyla; extirpated, range partially recolonised)[61][62] (C)
- Orycteropodidae/Tubulidentata
- Paenungulata
- Tethytheria
- All native spp. of Proboscidea
- Mastodons
- American mastodon (Mammut americanum) (H)
- Pacific mastodon (Mammut pacificus) (H) (validity uncertain)
- Gomphotheriidae spp.
- Cuvieronius[63] (H)
- Mammoth (Mammuthus) spp.
- Columbian mammoth (Mammuthus columbi) (H)
- Pygmy mammoth (Mammuthus exilis) (H)
- Woolly mammoth (Mammuthus primigenius) (H)
- Mastodons
- Sirenia
- Dugongidae
- Steller's sea cow (Hydrodamalis gigas; extirpated from North America, survived in Beringia into 18th century) (H)
- Dugongidae
- All native spp. of Proboscidea
- Tethytheria
- Afroinsectiphilia
- Euarchontoglires
- Bats
- Stock's vampire bat (Desmodus stocki) (C)
- Pristine mustached bat (Pteronotus (Phyllodia) pristinus) (C)
- Rodents
- Giant beaver (Castoroides) spp.
- Klein's porcupine (Erethizon kleini)[64] (H)
- Giant island deer mouse (Peromyscus nesodytes) (C)
- Neochoerus spp. e.g.
- Pinckney's capybara (Neochoerus pinckneyi) (H)
- Neochoerus aesopi (H)
- Neotoma findleyi
- Neotoma pygmaea
- Synaptomys australis
- All giant hutia (Heptaxodontidae) spp.
- Blunt-toothed giant hutia (Amblyrhiza inundata; could grow as large as an American black bear) (H)
- Plate-toothed giant hutia (Elasmodontomys obliquus) (H)
- Twisted-toothed mouse (Quemisia gravis) (H)
- Osborn's key mouse (Clidomys osborn's) (H)
- Xaymaca fulvopulvis (H)
- Lagomorphs
- Aztlan rabbit (Aztlanolagus sp.) (H)
- Giant pika (Ochotona whartoni) (H)
- Bats
- Eulipotyphla
- Xenarthrana
- All remaining ground sloth spp.
- Eremotherium (megatheriid giant ground sloth) (H)
- Nothrotheriops (nothrotheriid ground sloth) (H)
- Megalonychid ground sloth spp.
- Megalonyx (H)
- Nohochichak[65][66] (H)
- Xibalbaonyx[67][68] (H)
- Meizonyx
- Mylodontid ground sloth spp.
- Paramylodon (H)
- All members of Glyptodontidae
- Glyptotherium[69] (H)
- (H)
- Beautiful armadillo (Dasypus bellus)[70] (H)
- Pachyarmatherium
- All Pampatheriidae spp.
- Holmesina (H)
- Pampatherium (H)
- All remaining ground sloth spp.
- Birds
- Water Fowl
- Ducks
- Bermuda flightless duck (Anas pachyscelus) (H)
- Californian flightless sea duck (Chendytes lawi) (C)
- Mexican stiff-tailed duck (Oxyura zapatima)[60] (H)
- Ducks
- Turkey (Meleagris) spp.
- Californian turkey (Meleagris californica) (H)
- Meleagris crassipes[60] (H)
- Various Gruiformes spp.
- All cave rail (Nesotrochis) spp. e.g.
- Antillean cave rail (Nesotrochis debooyi) (C)
- Barbados rail (Incertae sedis) (C)
- Cuban flightless crane (Antigone cubensis) (H)
- La Brea crane (Grus pagei) (H)
- All cave rail (Nesotrochis) spp. e.g.
- Various flamingo (Phoenicopteridae) spp.
- Minute flamingo (Phoenicopterus minutus)[71] (C)
- Cope's flamingo (Phoenicopterus copei)[72] (C)
- Dow's puffin (Fratercula dowi) (C)
- Pleistocene Mexican diver spp.
- Storks
- La Brea/Asphalt stork (Ciconia maltha)[60] (C)
- Wetmore's stork (Mycteria wetmorei)[60] (C)
- Pleistocene Mexican cormorants spp. (genus Phalacrocorax)[60]
- Phalacrocorax goletensis (C)
- Phalacrocorax chapalensis (C)
- All remaining teratorn (Teratornithidae) spp.
- Several New World vultures (Cathartidae) spp.
- Pleistocene black vulture (Coragyps occidentalis ssp.) (C)
- Megafaunal Californian condor (Gymnogyps amplus) (C)
- Clark's condor (Breagyps clarki) (C)
- Cuban condor (Gymnogyps varonai) (C)
- Several Accipitridae spp.
- American neophrone vulture (Neophrontops americanus)[60][73] (C)
- Woodward's eagle (Amplibuteo woodwardi) (C)
- Cuban great hawk (Buteogallus borrasi) (C)
- Daggett's eagle (Buteogallus daggetti) (C)
- Fragile eagle (Buteogallus fragilis) (C)
- Cuban giant hawk (Gigantohierax suarezi)[74][75] (C)
- Errant eagle (Neogyps errans) (C)
- Grinnell's crested eagle (Spizaetus grinnelli)[60] (C)
- Willett's hawk-eagle (Spizaetus willetti)[60] (C)
- Caribbean titan hawk (Titanohierax) (C)
- Several owl (Strigiformes) spp.
- Brea miniature owl (Asphaltoglaux) (C)
- Kurochkin's pygmy owl (Glaucidium kurochkini) (C)
- Brea owl (Oraristix brea) (C)
- Cuban giant owl (Ornimegalonyx) (C)
- Bermuda flicker (Colaptes oceanicus) (C)
- Several caracara (Caracarinae) spp.
- Bahaman terrestrial caracara (Caracara sp.) (C)
- Puerto Rican terrestrial caracara (Caracara sp.) (C)
- Jamaican caracara (Carcara tellustris) (C)
- Cuban caracara (Milvago sp.) (C)
- Hispaniolan caracara (Milvago sp.) (C)
- Psittacopasserae
- Psittaciformes
- Mexican thick-billed parrot (Rhynchopsitta phillipsi)[60] (H)
- Psittaciformes
- Water Fowl
- Several giant tortoise spp.
- Hesperotestudo (H)
- Gopherus spp.
- Gopherus donlaloi (H)
- Chelonoidis spp.
- Chelonoidis marcanoi (H)
- Chelonoidis alburyorum (H)
The survivors are in some ways as significant as the losses: bison (H), grey wolf (C), lynx (C), grizzly bear (C), American black bear (C), deer (e.g. caribou, moose, wapiti (elk), Odocoileus spp.) (H), pronghorn (H), white-lipped peccary (H), muskox (H), bighorn sheep (H), and mountain goat (H); the list of survivors also include species which were extirpated during the Quaternary extinction event, but recolonised at least part of their ranges during the mid-Holocene from South American relict populations, such as the cougar (C), jaguar (C), giant anteater (C), collared peccary (H), ocelot (C) and jaguarundi (C). All save the pronghorns and giant anteaters were descended from Asian ancestors that had evolved with human predators.[76] Pronghorns are the second-fastest land mammal (after the cheetah), which may have helped them elude hunters. More difficult to explain in the context of overkill is the survival of bison, since these animals first appeared in North America less than 240,000 years ago and so were geographically removed from human predators for a sizeable period of time.[77][78][79] Because ancient bison evolved into living bison,[80][81] there was no continent-wide extinction of bison at the end of the Pleistocene (although the genus was regionally extirpated in many areas). The survival of bison into the Holocene and recent times is therefore inconsistent with the overkill scenario. By the end of the Pleistocene, when humans first entered North America, these large animals had been geographically separated from intensive human hunting for more than 200,000 years. Given this enormous span of geologic time, bison would almost certainly have been very nearly as naive as native North American large mammals.
The culture that has been connected with the wave of extinctions in North America is the paleo-American culture associated with the Clovis people (q.v.), who were thought to use spear throwers to kill large animals. The chief criticism of the "prehistoric overkill hypothesis" has been that the human population at the time was too small and/or not sufficiently widespread geographically to have been capable of such ecologically significant impacts. This criticism does not mean that climate change scenarios explaining the extinction are automatically to be preferred by default, however, any more than weaknesses in climate change arguments can be taken as supporting overkill. Some form of a combination of both factors could be plausible, and overkill would be a lot easier to achieve large-scale extinction with an already stressed population due to climate change.
South America
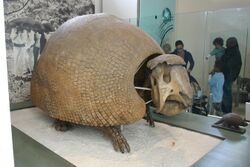
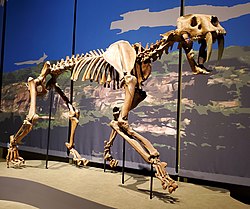
South America suffered among the worst losses of the continents, with around 83% of its megafauna going extinct.[9] Extinctions are thought to have occurred in the interval 13,000–10,000 years Before Present, coincident with the end of the Antarctic Cold Reversal (a cooling period earlier and less severe than the Northern Hemisphere Younger Dryas) and the emergence of Fishtail projectile points, which became widespread across South America. Fishtail projectile points are thought to have been used in big game hunting, though direct evidence of exploitation of extinct megafauna by humans is rare. Fishtail points rapidly disappeared after the extinction of the megafauna, and were replaced by other styles more suited to hunting smaller prey. Humans have traditionally been less cited as a causal factor in the extinctions than in North America, though some recent scholarship is beginning to challenge this.[82] Rock art shows that humans coexisted with South America's megafauna,[83] a finding also confirmed by anthropogenic interaction with megafaunal remains.[84]
- Ungulates
- Even-Toed Hoofed Mammals
- Several Cervidae spp.
- Morenelaphus
- Antifer
- Agalmaceros blicki[85][86]
- Odocoileus salinae[87][88]
- Various Camelidae spp.
- Eulamaops
- Stilt legged llama Hemiauchenia
- Stout legged llama Palaeolama
- Several Cervidae spp.
- Odd-Toed Hoofed Mammals
- All remaining Meridiungulata genera
- Order Litopterna
- Order Notoungulata
- Toxodontidae
- Piauhytherium (Some authors regard this taxon as synonym of Trigodonops)
- Mixotoxodon
- Toxodon
- Trigodonops
- Toxodontidae
- Even-Toed Hoofed Mammals
- Carnivora
- Feliformia
- Several Felidae spp.
- Saber-toothed cat (Smilodon) spp.[95]
- North American saber-toothed cat (Smilodon fatalis)
- South American saber-toothed cat (Smilodon populator)
- Patagonian jaguar (Panthera onca mesembrina) (some authors have suggested that these remains actually belong to the American lion instead[96])
- Saber-toothed cat (Smilodon) spp.[95]
- Several Felidae spp.
- Caniformia
- Canidae
- Dire wolf (Aenocyon dirus)
- Nehring's wolf (Canis nehringi)
- Protocyon spp.[97]
- Protocyon trogolodytes
- Protocyon tarijense
- Dusicyon avus
- Pleistocene bush dog (Speothos pacivorus)
- Arctoidea
- South American short-faced bear (Arctotherium spp.)
- Arctotherium bonairense
- Arctotherium tarijense
- Arctotherium wingei
- South American short-faced bear (Arctotherium spp.)
- Canidae
- Feliformia
- Rodents
- Bats
- Giant vampire bat (Desmodus draculae)
- All remaining Gomphotheridae spp.
- Xenarthrans
- All remaining ground sloth genera
- All remaining Glyptodontinae spp.
- Several Dasypodidae spp.
- Beautiful armadillo (Dasypus bellus)
- Eutatus
- Pachyarmatherium
- Propaopus[39][88]
- All Pampatheriidae spp.
- Holmesina (et 'Chlamytherium occidentale')[107][108]
- Pampatherium[109]
- Tonnicinctus[109]
- Birds
- Psilopterus (small terror bird remains dated to the Late Pleistocene,[110][111] but these are disputed)[112]
- Various Caracarinae spp.
- Various Cathartidae spp.
- Pampagyps imperator
- Geronogyps reliquus
- Wingegyps cartellei
- Pleistovultur nevesi
- Crocs & Gators
- Caiman venezuelensis
- Chelonoidis lutzae (Argentina)
Sahul (Australia-New Guinea) and the Pacific

A scarcity of reliably dated megafaunal bone deposits has made it difficult to construct timelines for megafaunal extinctions in certain areas, leading to a divide among researches about when and how megafaunal species went extinct.[116][117]
There are at least three hypotheses regarding the extinction of the Australian megafauna:
- that they went extinct with the arrival of the Aboriginal Australians on the continent,
- that they went extinct due to natural climate change.
This theory is based on evidence of megafauna surviving until 40,000 years ago, a full 30,000 years after homo sapiens first landed in Australia, and thus that the two groups coexisted for a long time. Evidence of these animals existing at that time come from fossil records and ocean sediment. To begin with, sediment core drilled in the Indian Ocean off the SW coast of Australia indicate the existence of a fungus called Sporormiella, which survived off the dung of plant-eating mammals. The abundance of these spores in the sediment prior to 45,000 years ago indicates that many large mammals existed in the southwest Australian landscape until that point. The sediment data also indicates that the megafauna population collapsed within a few thousand years, around the 45,000 years ago, suggesting a rapid extinction event.[118] In addition, fossils found at South Walker Creek, which is the youngest megafauna site in northern Australia, indicate that at least 16 species of megafauna survived there until 40,000 years ago. Furthermore, there is no firm evidence of homo sapiens living at South Walker Creek 40,000 years ago, therefore no human cause can be attributed to the extinction of these megafauna. However, there is evidence of major environmental deterioration of South Water Creek 40,000 years ago, which may have caused the extinct event. These changes include increased fire, reduction in grasslands, and the loss of fresh water.[119] The same environmental deterioration is seen across Australia at the time, further strengthening the climate change argument. Australia's climate at the time could best be described as an overall drying of the landscape due to lower precipitation, resulting in less fresh water availability and more drought conditions. Overall, this led to changes in vegetation, increased fires, overall reduction in grasslands, and a greater competition for already scarce fresh water.[120] These environmental changes proved to be too much for the Australian megafauna to cope with, causing the extinction of 90% of megafauna species.
- The third hypothesis shared by some scientists is that human impacts and natural climate changes led to the extinction of Australian megafauna. About 75% of Australia is semi-arid or arid, so it makes sense that megafauna species used the same fresh water resources as humans. This competition could have led to more hunting of megafauna.[121] Furthermore, Homo sapiens used fire agriculture[clarification needed] to burn impassable[clarification needed] land. This further diminished the already disappearing grassland which contained plants that were a key dietary component of herbivorous megafauna. While there is no scientific consensus on this, it is plausible that homo sapiens and natural climate change had a combined impact. Overall, there is a great deal of evidence for humans being the culprit, but by ruling out climate change completely as a cause of the Australian megafauna extinction we are not getting the whole picture. The climate change in Australia 45,000 years ago destabilized the ecosystem, making it particularly vulnerable to hunting and fire agriculture by humans; this is probably what led to the extinction of the Australian megafauna.
Several studies provide evidence that climate change caused megafaunal extinction during the Pleistocene in Australia. One group of researchers analyzed fossilized teeth found at Cuddie Springs in southeastern Australia. By analyzing oxygen isotopes, they measured aridity, and by analyzing carbon isotopes and dental microwear texture analysis, they assessed megafaunal diets and vegetation. During the middle Pleistocene, southeastern Australia was dominated by browsers, including fauna that consumed C4 plants. By the late Pleistocene, the C4 plant dietary component had decreased considerably. This shift may have been caused by increasingly arid conditions, which may have caused dietary restrictions. Other isotopic analyses of eggshells and wombat teeth also point to a decline of C4 vegetation after 45 Ka. This decline in C4 vegetation is coincident with increasing aridity. Increasingly arid conditions in southeastern Australia during the late Pleistocene may have stressed megafauna, and contributed to their decline.[122]

In Sahul (a former continent composed of Australia and New Guinea), the sudden and extensive spate of extinctions occurred earlier than in the rest of the world.[123][124][125][126] Most evidence points to a 20,000 year period after human arrival circa 63,000 BCE,[127] but scientific argument continues as to the exact date range.[128] In the rest of the Pacific (other Australasian islands such as New Caledonia, and Oceania) although in some respects far later, endemic fauna also usually perished quickly upon the arrival of humans in the late Pleistocene and early Holocene.
- Marsupials
- Various members of Diprotodontidae
- Diprotodon
- Hulitherium tomasetti
- Maokopia ronaldi
- Zygomaturus
- Palorchestes ("marsupial tapir")
- Various members of Vombatidae
- Lasiorhinus angustidens (giant wombat)
- Phascolonus (giant wombat)
- Ramasayia magna (giant wombat)
- Vombatus hacketti (Hackett's wombat)
- Warendja wakefieldi (dwarf wombat)
- Sedophascolomys (giant wombat)
- Phascolarctos stirtoni (giant koala)
- Marsupial lion (Thylacoleo carnifex)
- Various members of Macropodidae
- Procoptodon (short-faced kangaroos) e.g.
- Procoptodon goliah
- Sthenurus (giant kangaroo)
- Simosthenurus (giant kangaroo)
- Various Macropus (giant kangaroo) spp. e.g.
- Protemnodon (giant wallaby)
- Troposodon (wallaby)[123][124][129][130][131][132]
- Bohra (giant tree kangaroo)
- Propleopus oscillans (omnivorous, giant musky rat-kangaroo)
- Nombe
- Congruus
- Procoptodon (short-faced kangaroos) e.g.
- Various forms of Sarcophilus (Tasmanian devil)
- Sarcophilus laniarius (25% larger than modern species, unclear if it is actually a distinct species from living Tasmanian devil[133])
- Sarcophilus moornaensis
- Various members of Diprotodontidae
- Monotremes: egg-laying mammals.
- Echidna
- Murrayglossus hacketti (giant echidna)
- Megalibgwilia ramsayi
- Echidna
- Birds
- Pygmy Cassowary (Casuarius lydekkeri)
- Genyornis (a two-meter-tall (6.6 ft) dromornithid
- Giant malleefowl (Progura gallinacea)
- Cryptogyps lacertosus
- Dynatoaetus gaffae
- Several Phoenicopteridae spp.
- Xenorhynchopsis spp. (Australian flamingo)[134]
- Xenorhynchopsis minor
- Xenorhynchopsis tibialis
- Reptiles
- Crocs & Gators
- Ikanogavialis (the last fully marine crocodilian)
- Pallimnarchus (Australian freshwater mekosuchine crocodiian)
- Quinkana (Australian terrestrial mekosuchine crocodilian, apex predator)
- Volia (a two-to-three meter long mekosuchine crocodylian, apex predator of Pleistocene Fiji)
- Mekosuchus
- Mekosuchus inexpectatus (New Caledonian land crocodile)
- Mekosuchus kalpokasi (Vanuatu land crocodile)
- Varanus sp. (Pleistocene and Holocene New Caledonia)
- Megalania (Varanus pricus) (a giant predatory monitor lizard comparable or larger than the Komodo dragon)
- Snakes
- Wonambi (a five-to-six-metre-long Australian constrictor snake)
- Several spp. of Meiolaniidae (giant armoured turtles)
- Crocs & Gators
Relationship to later extinctions
There is no general agreement on where the Quaternary extinction event ends, and the Holocene, or anthropogenic, extinction begins, or if they should be considered separate events at all.[135][136] Some have suggested that anthropogenic extinctions may have begun as early as when the first modern humans spread out of Africa between 100,000 and 200,000 years ago, which is supported by rapid megafaunal extinction following recent human colonisation in Australia, New Zealand and Madagascar,[137] in a similar way that any large, adaptable predator moving into a new ecosystem would. In many cases, it is suggested even minimal hunting pressure was enough to wipe out large fauna, particularly on geographically isolated islands.[138][139] Only during the most recent parts of the extinction have plants also suffered large losses.[140]
Overall, the Holocene extinction can be characterised by the human impact on the environment. The Holocene extinction continues into the 21st century, with overfishing, ocean acidification and the amphibian crisis being a few broader examples of an almost universal, cosmopolitan decline of biodiversity.
Causes
Human activity
Hunting
The hunting hypothesis suggests that humans hunted megaherbivores to extinction, which in turn caused the extinction of carnivores and scavengers which had preyed upon those animals.[141][142][143] This hypothesis holds Pleistocene humans responsible for the megafaunal extinction. One variant, known as blitzkrieg, portrays this process as relatively quick. Some of the direct evidence for this includes: fossils of some megafauna found in conjunction with human remains, embedded arrows and tool cut marks found in megafaunal bones, and European cave paintings that depict such hunting. Biogeographical evidence is also suggestive: the areas of the world where humans evolved currently have more of their Pleistocene megafaunal diversity (the elephants and rhinos of Asia and Africa) compared to other areas such as Australia , the Americas, Madagascar and New Zealand without the earliest humans. The overkill hypothesis, a variant of the hunting hypothesis, was proposed in 1966 by Paul S. Martin,[144] Professor of Geosciences Emeritus at the Desert Laboratory of the University of Arizona.[145]
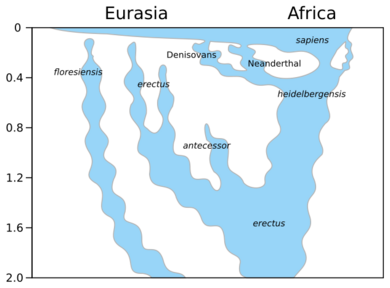
Circumstantially, the close correlation in time between the appearance of humans in an area and extinction there provides weight for this scenario.[146][8][2] Radiocarbon dating has supported the plausibility of this correlation being reflective of causation.[147] The megafaunal extinctions covered a vast period of time and highly variable climatic situations. The earliest extinctions in Australia were complete approximately 50,000 BP, well before the Last Glacial Maximum and before rises in temperature. The most recent extinction in New Zealand was complete no earlier than 500 BP and during a period of cooling. In between these extremes megafaunal extinctions have occurred progressively in such places as North America, South America and Madagascar with no climatic commonality. The only common factor that can be ascertained is the arrival of humans.[148][149] This phenomenon appears even within regions. The mammal extinction wave in Australia about 50,000 years ago coincides not with known climatic changes, but with the arrival of humans. In addition, large mammal species like the giant kangaroo Protemnodon appear to have succumbed sooner on the Australian mainland than on Tasmania, which was colonised by humans a few thousand years later.[150][151] A study published in 2015 supported the hypothesis further by running several thousand scenarios that correlated the time windows in which each species is known to have become extinct with the arrival of humans on different continents or islands. This was compared against climate reconstructions for the last 90,000 years. The researchers found correlations of human spread and species extinction indicating that the human impact was the main cause of the extinction, while climate change exacerbated the frequency of extinctions. The study, however, found an apparently low extinction rate in the fossil record of mainland Asia.[152][153] A 2020 study published in Science Advances found that human population size and/or specific human activities, not climate change, caused rapidly rising global mammal extinction rates during the past 126,000 years. Around 96% of all mammalian extinctions over this time period are attributable to human impacts. According to Tobias Andermann, lead author of the study, "these extinctions did not happen continuously and at constant pace. Instead, bursts of extinctions are detected across different continents at times when humans first reached them. More recently, the magnitude of human driven extinctions has picked up the pace again, this time on a global scale."[154][155] On a related note, the population declines of still extant megafauna during the Pleistocene have also been shown to correlate with human expansion rather than climate change.[13]
The extinction's extreme bias towards larger animals further supports a relationship with human activity rather than climate change.[156] There is evidence that the average size of mammalian fauna declined over the course of the Quaternary,[157] a phenomenon that was likely linked to disproportionate hunting of large animals by humans.[4]
Extinction through human hunting has been supported by archaeological finds of mammoths with projectile points embedded in their skeletons, by observations of modern naive animals allowing hunters to approach easily[158][159][160] and by computer models by Mosimann and Martin,[161] and Whittington and Dyke,[162] and most recently by Alroy.[163]
Major objections have been raised regarding the hunting hypothesis. Notable among them is the sparsity of evidence of human hunting of megafauna.[164][165][166] There is no archeological evidence that in North America megafauna other than mammoths, mastodons, gomphotheres and bison were hunted, despite the fact that, for example, camels and horses are very frequently reported in fossil history.[167] Overkill proponents, however, say this is due to the fast extinction process in North America and the low probability of animals with signs of butchery to be preserved.[168] The majority of North American taxa have too sparse a fossil record to accurately assess the frequency of human hunting of them.[9] A study by Surovell and Grund concluded "archaeological sites dating to the time of the coexistence of humans and extinct fauna are rare. Those that preserve bone are considerably more rare, and of those, only a very few show unambiguous evidence of human hunting of any type of prey whatsoever."[169] Eugene S. Hunn points out that the birthrate in hunter-gatherer societies is generally too low, that too much effort is involved in the bringing down of a large animal by a hunting party, and that in order for hunter-gatherers to have brought about the extinction of megafauna simply by hunting them to death, an extraordinary amount of meat would have had to have been wasted.[170]
Second-order predation
The Second-Order Predation Hypothesis says that as humans entered the New World they continued their policy of killing predators, which had been successful in the Old World but because they were more efficient and because the fauna, both herbivores and carnivores, were more naive, they killed off enough carnivores to upset the ecological balance of the continent, causing overpopulation, environmental exhaustion, and environmental collapse. The hypothesis accounts for changes in animal, plant, and human populations.
The scenario is as follows:
- After the arrival of H. sapiens in the New World, existing predators must share the prey populations with this new predator. Because of this competition, populations of original, or first-order, predators cannot find enough food; they are in direct competition with humans.
- Second-order predation begins as humans begin to kill predators.
- Prey populations are no longer well controlled by predation. Killing of nonhuman predators by H. sapiens reduces their numbers to a point where these predators no longer regulate the size of the prey populations.
- Lack of regulation by first-order predators triggers boom-and-bust cycles in prey populations. Prey populations expand and consequently overgraze and over-browse the land. Soon the environment is no longer able to support them. As a result, many herbivores starve. Species that rely on the slowest recruiting food become extinct, followed by species that cannot extract the maximum benefit from every bit of their food.
- Boom-bust cycles in herbivore populations change the nature of the vegetative environment, with consequent climatic impacts on relative humidity and continentality. Through overgrazing and overbrowsing, mixed parkland becomes grassland, and climatic continentality increases.
The second-order predation hypothesis has been supported by a computer model, the Pleistocene extinction model (PEM), which, using the same assumptions and values for all variables (herbivore population, herbivore recruitment rates, food needed per human, herbivore hunting rates, etc.) other than those for hunting of predators. It compares the overkill hypothesis (predator hunting = 0) with second-order predation (predator hunting varied between 0.01 and 0.05 for different runs). The findings are that second-order predation is more consistent with extinction than is overkill[171][172] (results graph at left). The Pleistocene extinction model is the only test of multiple hypotheses and is the only model to specifically test combination hypotheses by artificially introducing sufficient climate change to cause extinction. When overkill and climate change are combined they balance each other out. Climate change reduces the number of plants, overkill removes animals, therefore fewer plants are eaten. Second-order predation combined with climate change exacerbates the effect of climate change.[173] (results graph at right). The second-order predation hypothesis is further supported by the observation above that there was a massive increase in bison populations.[174]
However, this hypothesis has been criticised on the grounds that the multispecies model produces a mass extinction through indirect competition between herbivore species: small species with high reproductive rates subsidize predation on large species with low reproductive rates.[163] All prey species are lumped in the Pleistocene extinction model. Also, the control of population sizes by predators is not fully supported by observations of modern ecosystems.[175] The hypothesis further assumes decreases in vegetation due to climate change, but deglaciation doubled the habitable area of North America. Any vegetational changes that did occur failed to cause almost any extinctions of small vertebrates, and they are more narrowly distributed on average, which detractors cite as evidence against the hypothesis.
Competition for water
In southeastern Australia, the scarcity of water during the interval in which humans arrived in Australia suggests that human competition with megafauna for precious water sources may have played a role in the extinction of the latter.[121]
Landscape alteration
One consequence of the colonisation by humans of lands previously uninhabited by them may have been the introduction of new fire regimes because of extensive fire use by humans.[6] There is evidence that anthropogenic fire use had major impacts on the local environments in both Australia[5] and North America.[176]
Climate change
At the end of the 19th and beginning of the 20th centuries, when scientists first realized that there had been glacial and interglacial ages, and that they were somehow associated with the prevalence or disappearance of certain animals, they surmised that the termination of the Pleistocene ice age might be an explanation for the extinctions.
The most obvious change associated with the termination of an ice age is the increase in temperature. Between 15,000 BP and 10,000 BP, a 6 °C increase in global mean annual temperatures occurred. This was generally thought to be the cause of the extinctions. According to this hypothesis, a temperature increase sufficient to melt the Wisconsin ice sheet could have placed enough thermal stress on cold-adapted mammals to cause them to die. Their heavy fur, which helps conserve body heat in the glacial cold, might have prevented the dumping of excess heat, causing the mammals to die of heat exhaustion. Large mammals, with their reduced surface area-to-volume ratio, would have fared worse than small mammals. A study covering the past 56,000 years indicates that rapid warming events with temperature changes of up to 16 °C (29 °F) had an important impact on the extinction of megafauna. Ancient DNA and radiocarbon data indicates that local genetic populations were replaced by others within the same species or by others within the same genus. Survival of populations was dependent on the existence of refugia and long distance dispersals, which may have been disrupted by human hunters.[177]
Other scientists have proposed that increasingly extreme weather—hotter summers and colder winters—referred to as "continentality", or related changes in rainfall caused the extinctions. It has been shown that vegetation changed from mixed woodland-parkland to separate prairie and woodland.[178][179][180] This may have affected the kinds of food available. Shorter growing seasons may have caused the extinction of large herbivores and the dwarfing of many others. In this case, as observed, bison and other large ruminants would have fared better than horses, elephants and other monogastrics, because ruminants are able to extract more nutrition from limited quantities of high-fiber food and better able to deal with anti-herbivory toxins.[181][182][183] So, in general, when vegetation becomes more specialized, herbivores with less diet flexibility may be less able to find the mix of vegetation they need to sustain life and reproduce, within a given area. Increased continentality resulted in reduced and less predictable rainfall limiting the availability of plants necessary for energy and nutrition.[184][185][186] It has been suggested that this change in rainfall restricted the amount of time favorable for reproduction.[187][188] This could disproportionately harm large animals, since they have longer, more inflexible mating periods, and so may have produced young at unfavorable seasons (i.e., when sufficient food, water, or shelter was unavailable because of shifts in the growing season). In contrast, small mammals, with their shorter life cycles, shorter reproductive cycles, and shorter gestation periods, could have adjusted to the increased unpredictability of the climate, both as individuals and as species which allowed them to synchronize their reproductive efforts with conditions favorable for offspring survival. If so, smaller mammals would have lost fewer offspring and would have been better able to repeat the reproductive effort when circumstances once more favored offspring survival.[189] A study looking at the environmental conditions across Europe, Siberia and the Americas from 25,000 to 10,000 YBP found that prolonged warming events leading to deglaciation and maximum rainfall occurred just prior to the transformation of the rangelands that supported megaherbivores into widespread wetlands that supported herbivore-resistant plants. The study proposes that moisture-driven environmental change led to the megafaunal extinctions and that Africa's trans-equatorial position allowed rangeland to continue to exist between the deserts and the central forests, therefore fewer megafauna species became extinct there.[177]
Evidence in Southeast Asia, in contrast to Europe, Australia, and the Americas, suggests that climate change and an increasing sea level were significant factors in the extinction of several herbivorous species. Alterations in vegetation growth and new access routes for early humans and mammals to previously isolated, localized ecosystems were detrimental to select groups of fauna.[190]
Some evidence from Europe also suggests climatic changes were responsible for extinctions there, as the individuals extinctions tended to occur during times of environmental change and did not correlate particularly well with human migrations.[1]
In Australia, some studies have suggested that extinctions of megafauna began before the peopling of the continent, favouring climate change as the driver.[191]
In Beringia, megafauna may have gone extinct because of particularly intense paludification and because the land connection between Eurasia and North America flooded before the Cordilleran Ice Sheet retreated far enough to reopen the corridor between Beringia and the remainder of North America.[192] Woolly mammoths became extirpated from Beringia because of climatic factors, although human activity also played a synergistic role in their decline.[193] In North America, a Radiocarbon-dated Event-Count (REC) modelling study found that megafaunal declines in North America correlated with climatic changes instead of human population expansion.[194]
In the North American Great Lakes region, the population declines of mastodons and mammoths have been found to correlate with climatic fluctuations during the Younger Dryas rather than human activity.[195]
In the Argentine Pampas, the flooding of vast swathes of the once much larger Pampas grasslands may have played a role in the extinctions of its megafaunal assemblages.[7]
Critics object that since there were multiple glacial advances and withdrawals in the evolutionary history of many of the megafauna, it is rather implausible that only after the last glacial maximum would there be such extinctions. Proponents of climate change as the extinction event's cause like David J. Meltzer suggest that the last deglaciation may have been markedly different from previous ones.[196] Also, one study suggests that the Pleistocene megafaunal composition may have differed markedly from that of earlier interglacials, making the Pleistocene populations particularly vulnerable to changes in their environment.[197]
Studies propose that the annual mean temperature of the current interglacial that we have seen for the last 10,000 years is no higher than that of previous interglacials, yet most of the same large mammals survived similar temperature increases.[198][199][200] In addition, numerous species such as mammoths on Wrangel Island and St. Paul Island survived in human-free refugia despite changes in climate.[201] This would not be expected if climate change were responsible (unless their maritime climates offered some protection against climate change not afforded to coastal populations on the mainland). Under normal ecological assumptions island populations should be more vulnerable to extinction due to climate change because of small populations and an inability to migrate to more favorable climes.[citation needed]
Critics have also identified a number of problems with the continentality hypotheses. Megaherbivores have prospered at other times of continental climate. For example, megaherbivores thrived in Pleistocene Siberia, which had and has a more continental climate than Pleistocene or modern (post-Pleistocene, interglacial) North America.[202][203][204] The animals that became extinct actually should have prospered during the shift from mixed woodland-parkland to prairie, because their primary food source, grass, was increasing rather than decreasing.[205][204][206] Although the vegetation did become more spatially specialized, the amount of prairie and grass available increased, which would have been good for horses and for mammoths, and yet they became extinct. This criticism ignores the increased abundance and broad geographic extent of Pleistocene bison at the end of the Pleistocene, which would have increased competition for these resources in a manner not seen in any earlier interglacials.[197] Although horses became extinct in the New World, they were successfully reintroduced by the Spanish in the 16th century—into a modern post-Pleistocene, interglacial climate. Today there are feral horses still living in those same environments. They find a sufficient mix of food to avoid toxins, they extract enough nutrition from forage to reproduce effectively and the timing of their gestation is not an issue. Of course, this criticism ignores the obvious fact that present-day horses are not competing for resources with ground sloths, mammoths, mastodons, camels, llamas, and bison. Similarly, mammoths survived the Pleistocene Holocene transition on isolated, uninhabited islands in the Mediterranean Sea until 4,000 to 7,000 years ago,[207] as well as on Wrangel Island in the Siberian Arctic.[208] Additionally, large mammals should have been able to migrate, permanently or seasonally, if they found the temperature too extreme, the breeding season too short, or the rainfall too sparse or unpredictable.[209] Seasons vary geographically. By migrating away from the equator, herbivores could have found areas with growing seasons more favorable for finding food and breeding successfully. Modern-day African elephants migrate during periods of drought to places where there is apt to be water.[210] Large animals also store more fat in their bodies than do medium-sized animals and this should have allowed them to compensate for extreme seasonal fluctuations in food availability.[211]
Some evidence weighs against climate change as a valid hypothesis as applied to Australia. It has been shown that the prevailing climate at the time of extinction (40,000–50,000 BP) was similar to that of today, and that the extinct animals were strongly adapted to an arid climate. The evidence indicates that all of the extinctions took place in the same short time period, which was the time when humans entered the landscape. The main mechanism for extinction was probably fire (started by humans) in a then much less fire-adapted landscape. Isotopic evidence shows sudden changes in the diet of surviving species, which could correspond to the stress they experienced before extinction.[212][213][214]
Some evidence obtained from analysis of the tusks of mastodons from the American Great Lakes region appears inconsistent with the climate change hypothesis. Over a span of several thousand years prior to their extinction in the area, the mastodons show a trend of declining age at maturation. This is the opposite of what one would expect if they were experiencing stresses from deteriorating environmental conditions, but is consistent with a reduction in intraspecific competition that would result from a population being reduced by human hunting.[215]
It may be observed that neither the overkill nor the climate change hypotheses can fully explain events: browsers, mixed feeders and non-ruminant grazer species suffered most, while relatively more ruminant grazers survived.[216] However, a broader variation of the overkill hypothesis may predict this, because changes in vegetation wrought by either Second Order Predation (see below)[173][217] or anthropogenic fire preferentially selects against browse species.[citation needed]
Disease
The hyperdisease hypothesis, as advanced by Ross D. E. MacFee and Preston A. Marx, attributes the extinction of large mammals during the late Pleistocene to indirect effects of the newly arrived aboriginal humans.[218][219][220] In more recent times, disease has driven many vulnerable species to extinction; the introduction of avian malaria and avipoxvirus, for example, has greatly decreased the populations of the endemic birds of Hawaii, with some going extinct.[221] The hyperdisease hypothesis proposes that humans or animals traveling with them (e.g., chickens or domestic dogs) introduced one or more highly virulent diseases into vulnerable populations of native mammals, eventually causing extinctions. The extinction was biased toward larger-sized species because smaller species have greater resilience because of their life history traits (e.g., shorter gestation time, greater population sizes, etc.). Humans are thought to be the cause because other earlier immigrations of mammals into North America from Eurasia did not cause extinctions.[218] A similar suggestion is that pathogens were transmitted by the expanding humans via the domesticated dogs they brought with them.[222] A related theory proposes that a highly contagious prion disease similar to chronic wasting disease or scrapie that was capable of infecting a large number of species was the culprit. Animals weakened by this "superprion" would also have easily become reservoirs of viral and bacterial diseases as they succumbed to neurological degeneration from the prion, causing a cascade of different diseases to spread among various mammal species. This theory could potentially explain the prevalence of heterozygosity at codon 129 of the prion protein gene in humans, which has been speculated to be the result of natural selection against homozygous genotypes that were more susceptible to prion disease and thus potentially a tell-tale of a major prion pandemic that affected humans of or younger than reproductive age far in the past and disproportionately killed before they could reproduce those with homozygous genotypes at codon 129.[223]
If a disease was indeed responsible for the end-Pleistocene extinctions, then there are several criteria it must satisfy (see Table 7.3 in MacPhee & Marx 1997). First, the pathogen must have a stable carrier state in a reservoir species. That is, it must be able to sustain itself in the environment when there are no susceptible hosts available to infect. Second, the pathogen must have a high infection rate, such that it is able to infect virtually all individuals of all ages and sexes encountered. Third, it must be extremely lethal, with a mortality rate of c. 50–75%. Finally, it must have the ability to infect multiple host species without posing a serious threat to humans. Humans may be infected, but the disease must not be highly lethal or able to cause an epidemic.[citation needed]
As with other hypotheses, a number of counterarguments to the hyperdisease hypothesis have been put forth. Generally speaking, disease has to be very virulent to kill off all the individuals in a genus or species. Even such a virulent disease as West Nile fever is unlikely to have caused extinction.[224] The disease would need to be implausibly selective while being simultaneously implausibly broad. Such a disease needs to be capable of killing off wolves such as Canis dirus or goats such as Oreamnos harringtoni while leaving other very similar species (Canis lupus and Oreamnos americanus, respectively) unaffected. It would need to be capable of killing off flightless birds while leaving closely related flighted species unaffected. Yet while remaining sufficiently selective to afflict only individual species within genera it must be capable of fatally infecting across such clades as birds, marsupials, placentals, testudines, and crocodilians. No disease with such a broad scope of fatal infectivity is known, much less one that remains simultaneously incapable of infecting numerous closely related species within those disparate clades. On the other hand, this objection does not account for the possibility of a variety of different diseases being introduced around the same era.[citation needed] Numerous species including wolves, mammoths, camelids, and horses had emigrated continually between Asia and North America over the past 100,000 years. For the disease hypothesis to be applicable there it would require that the population remain immunologically naive despite this constant transmission of genetic and pathogenic material.[citation needed] The dog-specific hypothesis in particular cannot account for several major extinction events, notably the Americas (for reasons already covered) and Australia. Dogs did not arrive in Australia until approximately 35,000 years after the first humans arrived there, and approximately 30,000 years after the Australian megafaunal extinction was complete.[citation needed]
Extraterrestrial impact
An extraterrestrial impact, which has occasionally been proposed as a cause of the Younger Dryas,[225] is sometimes suggested as a potential cause of the extinction of North America's megafauna due to the temporal proximity between a proposed date for such an impact and the following megafaunal extinctions.[226][3] However, the Younger Dryas impact hypothesis is widely considered a fringe hypothesis that is unsupported by evidence.[227][228]
Geomagnetic field weakening
A weakening of the Earth's magnetic field may have caused increased flux of UV-B radiation and has been suggested as a cause of megafaunal extinctions in the Late Quaternary.[229]
Effects
The extinction of the megafauna could have caused the disappearance of the mammoth steppe rather than the other way around. Alaska now has low nutrient soil unable to support bison, mammoths, and horses. R. Dale Guthrie has claimed this as a cause of the extinction of the megafauna there; however, he may be interpreting it backwards. The loss of large herbivores to break up the permafrost allows the cold soils that are unable to support large herbivores today. Today, in the arctic, where trucks have broken the permafrost grasses and diverse flora and fauna can be supported.[230][231] In addition, Chapin (Chapin 1980) showed that simply adding fertilizer to the soil in Alaska could make grasses grow again like they did in the era of the mammoth steppe. Possibly, the extinction of the megafauna and the corresponding loss of dung is what led to low nutrient levels in modern-day soil and therefore is why the landscape can no longer support megafauna.
Megafauna play a significant role in the lateral transport of mineral nutrients in an ecosystem, tending to translocate them from areas of high to those of lower abundance. They do so by their movement between the time they consume the nutrient and the time they release it through elimination (or, to a much lesser extent, through decomposition after death).[232] In South America's Amazon Basin, it is estimated that such lateral diffusion was reduced over 98% following the megafaunal extinctions that occurred roughly 12,500 years ago.[233][234] Given that phosphorus availability is thought to limit productivity in much of the region, the decrease in its transport from the western part of the basin and from floodplains (both of which derive their supply from the uplift of the Andes) to other areas is thought to have significantly impacted the region's ecology, and the effects may not yet have reached their limits.[234] The extinction of the mammoths allowed grasslands they had maintained through grazing habits to become birch forests.[235] The new forest and the resulting forest fires may have induced climate change.[235] Such disappearances might be the result of the proliferation of modern humans.[236][237]
Large populations of megaherbivores have the potential to contribute greatly to the atmospheric concentration of methane, which is an important greenhouse gas. Modern ruminant herbivores produce methane as a byproduct of foregut fermentation in digestion, and release it through belching or flatulence. Today, around 20% of annual methane emissions come from livestock methane release. In the Mesozoic, it has been estimated that sauropods could have emitted 520 million tons of methane to the atmosphere annually,[238] contributing to the warmer climate of the time (up to 10 °C warmer than at present).[238][239] This large emission follows from the enormous estimated biomass of sauropods, and because methane production of individual herbivores is believed to be almost proportional to their mass.[238]
Recent studies have indicated that the extinction of megafaunal herbivores may have caused a reduction in atmospheric methane. One study examined the methane emissions from the bison that occupied the Great Plains of North America before contact with European settlers. The study estimated that the removal of the bison caused a decrease of as much as 2.2 million tons per year.[240] Another study examined the change in the methane concentration in the atmosphere at the end of the Pleistocene epoch after the extinction of megafauna in the Americas. After early humans migrated to the Americas about 13,000 BP, their hunting and other associated ecological impacts led to the extinction of many megafaunal species there. Calculations suggest that this extinction decreased methane production by about 9.6 million tons per year. This suggests that the absence of megafaunal methane emissions may have contributed to the abrupt climatic cooling at the onset of the Younger Dryas. The decrease in atmospheric methane that occurred at that time, as recorded in ice cores, was 2–4 times more rapid than any other decrease in the last half million years, suggesting that an unusual mechanism was at work.[241]
The extermination of megafauna left many niches vacant, which has been cited as an explanation for the vulnerability and fragility of many ecosystems to destruction in the later Holocene extinction. The comparative lack of megafauna in modern ecosystems has reduced high-order interactions among surviving species, reducing ecological complexity.[242] This depauperate, post-megafaunal ecological state has been associated with diminished ecological resilience to stressors.[243] Many extant species of plants have adaptations that were advantageous in the presence of megafauna but are now useless in their absence.[244] The demise of megafaunal ecosystem engineers in the Arctic that maintained open grassland environments has been highly detrimental to shorebirds of the genus Numenius.[245]
Human perception
Analysis of Maori oral traditions has found that the extinction of New Zealand's megafauna during the Holocene extinction had significant social and linguistic impacts on their culture, suggesting that similar phenomena may have occurred in other societies that lived through megafaunal extinctions.[246]
See also
- Earth:Holocene extinction – Ongoing extinction event caused by human activity
- Biology:List of quaternary mammalian fauna of China
- Biology:Megafauna – Large animals
- Biology:Pleistocene megafauna – Large animals that lived during the Pleistocene
- Biology:Pleistocene rewilding – Ecological practice
- Earth:Toba catastrophe theory – Supereruption 74,000 years ago that may have caused a global volcanic winter
References
- ↑ 1.0 1.1 1.2 1.3 Stuart, Anthony John (1999), MacPhee, Ross D. E., ed., "Late Pleistocene Megafaunal Extinctions: A European Perspective" (in en), Extinctions in Near Time (Boston, MA: Springer US): pp. 257–269, doi:10.1007/978-1-4757-5202-1_11, ISBN 978-1-4419-3315-7, http://link.springer.com/10.1007/978-1-4757-5202-1_11, retrieved 2023-05-28
- ↑ 2.0 2.1 2.2 2.3 Sandom, Christopher; Faurby, Søren; Sandel, Brody; Svenning, Jens-Christian (22 July 2014). "Global late Quaternary megafauna extinctions linked to humans, not climate change" (in en). Proceedings of the Royal Society B: Biological Sciences 281 (1787): 20133254. doi:10.1098/rspb.2013.3254. ISSN 0962-8452. PMID 24898370.
- ↑ 3.0 3.1 3.2 3.3 Faith, J. Tyler; Surovell, Todd A. (8 December 2009). "Synchronous extinction of North America's Pleistocene mammals" (in en). Proceedings of the National Academy of Sciences of the United States of America 106 (49): 20641–20645. doi:10.1073/pnas.0908153106. ISSN 0027-8424. PMID 19934040. Bibcode: 2009PNAS..10620641F.
- ↑ 4.0 4.1 Dembitzer, Jacob; Barkai, Ran; Ben-Dor, Miki; Meiri, Shai (15 January 2022). "Levantine overkill: 1.5 million years of hunting down the body size distribution". Quaternary Science Reviews 276: 107316. doi:10.1016/j.quascirev.2021.107316. Bibcode: 2022QSRv..27607316D.
- ↑ 5.0 5.1 Bird, Michael I.; Hutley, Lindsay B.; Lawes, Michael J.; Lloyd, Jon; Luly, Jon G.; Ridd, Peter V.; Roberts, Richard G.; Ulm, Sean et al. (July 2013). "Humans, megafauna and environmental change in tropical Australia" (in en). Journal of Quaternary Science 28 (5): 439–452. doi:10.1002/jqs.2639.
- ↑ 6.0 6.1 Koch, Paul L.; Barnosky, Anthony D. (1 January 2006). "Late Quaternary Extinctions: State of the Debate". Annual Review of Ecology, Evolution, and Systematics 37 (1): 215–250. doi:10.1146/annurev.ecolsys.34.011802.132415.
- ↑ 7.0 7.1 Prado, José L.; Martinez-Maza, Cayetana; Alberdi, María T. (May 2015). "Megafauna extinction in South America: A new chronology for the Argentine Pampas" (in en). Palaeogeography, Palaeoclimatology, Palaeoecology 425: 41–49. doi:10.1016/j.palaeo.2015.02.026. Bibcode: 2015PPP...425...41P.
- ↑ 8.0 8.1 Frédérik Saltré; Marta Rodríguez-Rey; Barry W. Brook; Christopher N. Johnson (2016). "Climate change not to blame for late Quaternary megafauna extinctions in Australia". Nature Communications 7: 10511. doi:10.1038/ncomms10511. PMID 26821754. Bibcode: 2016NatCo...710511S.
- ↑ 9.0 9.1 9.2 Anthony D. Barnosky; Paul L. Koch; Robert S. Feranec; Scott L. Wing; Alan B. Shabel (2004). "Assessing the Causes of Late Pleistocene Extinctions on the Continents". Science 306 (5693): 70–75. doi:10.1126/science.1101476. PMID 15459379. Bibcode: 2004Sci...306...70B.
- ↑ Putshkov, P. V. (1997). "Were the Mammoths killed by the warming? (Testing of the climatic versions of the Wurm extinctions)". Vestnik Zoologii (Supplement No.4).
- ↑ 11.0 11.1 Gillespie, Richard (2008). "Updating Martin's global extinction model". Quaternary Science Reviews 27 (27–28): 2522–2529. doi:10.1016/j.quascirev.2008.09.007. Bibcode: 2008QSRv...27.2522G.
- ↑ Anderson, Paul K. (July 1995). "Competition, Predation, and the Evolution and Extinction of Steller's Sea Cow, Hydrodamalis Gigas". Marine Mammal Science 11 (3): 391–4. doi:10.1111/j.1748-7692.1995.tb00294.x. Bibcode: 1995MMamS..11..391A.
- ↑ 13.0 13.1 Bergman, Juraj; Pedersen, Rasmus Ø; Lundgren, Erick J.; Lemoine, Rhys T.; Monsarrat, Sophie; Pearce, Elena A.; Schierup, Mikkel H.; Svenning, Jens-Christian (24 November 2023). "Worldwide Late Pleistocene and Early Holocene population declines in extant megafauna are associated with Homo sapiens expansion rather than climate change" (in en). Nature Communications 14 (1): 7679. doi:10.1038/s41467-023-43426-5. ISSN 2041-1723.
- ↑ Holliday, Vance T.; Bartlein, Patrick J.; Scott, Andrew C.; Marlon, Jennifer R., Wikidata Q91978737
- ↑ Lan, Tianying; Lindqvist, Charlotte (2018). "Paleogenomics: Genome-Scale Analysis of Ancient DNA and Population and Evolutionary Genomic Inferences". in Lindqvist, C.; Rajora, O.. Population Genomics. pp. 323–360. doi:10.1007/13836_2017_7. ISBN 978-3-030-04587-6.
- ↑ Faith, J. Tyler (January 2014). "Late Pleistocene and Holocene mammal extinctions on continental Africa" (in en). Earth-Science Reviews 128: 105–121. doi:10.1016/j.earscirev.2013.10.009. Bibcode: 2014ESRv..128..105F.
- ↑ Heinrich, Earl (31 October 2013). "Ancient Nubia". Cambridge Online Histories. http://www.earlheinrich.com/Ancient%20Nubia/_Private/Ch%203%20excerpt.pdf.
- ↑ Turvey, Samuel T.; Sathe, Vijay; Crees, Jennifer J.; Jukar, Advait M.; Chakraborty, Prateek; Lister, Adrian M. (January 2021). "Late Quaternary megafaunal extinctions in India: How much do we know?" (in en). Quaternary Science Reviews 252: 106740. doi:10.1016/j.quascirev.2020.106740. Bibcode: 2021QSRv..25206740T.
- ↑ Turvey, Samuel T.; Tong, Haowen; Stuart, Anthony J.; Lister, Adrian M. (September 2013). "Holocene survival of Late Pleistocene megafauna in China: a critical review of the evidence" (in en). Quaternary Science Reviews 76: 156–166. doi:10.1016/j.quascirev.2013.06.030. Bibcode: 2013QSRv...76..156T.
- ↑ Louys, Julien; Roberts, Patrick (2020-10-15). "Environmental drivers of megafauna and hominin extinction in Southeast Asia" (in en). Nature 586 (7829): 402–406. doi:10.1038/s41586-020-2810-y. ISSN 0028-0836. PMID 33029012. Bibcode: 2020Natur.586..402L. https://www.nature.com/articles/s41586-020-2810-y.
- ↑ Hasegawa, Y.; Okumura, Y.; Tatsukawa, H. (2009). "First record of Late Pleistocene Bison from the fissure deposits of the Kuzuu Limestone, Yamasuge,Sano-shi,Tochigi Prefecture, Japan". Gunma Museum of Natural History and Kuzuu Fossil Museum 13: 47–52. http://www.gmnh.pref.gunma.jp/wp-content/uploads/bulletin13_5.pdf.
- ↑ Kurosawa, Y.. "モノが語る牛と人間の文化 – ② 岩手の牛たち". LIAJ News No.109- Oshu City Cattle Museum p. 29-31. http://liaj.lin.gr.jp/japanese/liajnews/liaj10909.pdf.
- ↑ Bison hanaizumiensis - TrekGEO
- ↑ Rozzi, Roberto (2017-02-01). "A new extinct dwarfed buffalo from Sulawesi and the evolution of the subgenus Anoa: An interdisciplinary perspective". Quaternary Science Reviews 157: 188–205. doi:10.1016/j.quascirev.2016.12.011. Bibcode: 2017QSRv..157..188R.
- ↑ Geist, Valerius (1998). Deer of the World: Their Evolution, Behaviour, and Ecology. Stackpole Books. ISBN 9780811704960. https://books.google.com/books?id=bcWZX-IMEVkC&q=sinomegaceros&pg=PA125.
- ↑ Jukar, Advait M.; Patnaik, Rajeev; Chauhan, Parth R.; Li, Hong-Chun; Lin, Jih-Pai (2019-09-10). "The youngest occurrence of Hexaprotodon Falconer and Cautley, 1836 (Hippopotamidae, Mammalia) from South Asia with a discussion on its extinction". Quaternary International. AMS 14C Applications II 528: 130–137. doi:10.1016/j.quaint.2019.01.005. ISSN 1040-6182. Bibcode: 2019QuInt.528..130J.
- ↑ "Rock paintings show species that roamed India". http://www.newindianexpress.com/nation/article76865.ece?service=print.
- ↑ Feldhamer, George A.; Drickamer, Lee C.; Vessey, Stephen H.; Merritt, Joseph F.; Krajewski, Carey (2015). Mammalogy: Adaptation, Diversity, Ecology. JHU Press. ISBN 9781421415888. https://books.google.com/books?id=Ugq5BgAAQBAJ&q=aardvark+indian+fossils&pg=PA349.
- ↑ Watanabe, Junya; Matsuoka, Hiroshige (2015-11-02). "Flightless diving duck (Aves, Anatidae) from the Pleistocene of Shiriya, northeast Japan". Journal of Vertebrate Paleontology 35 (6): e994745. doi:10.1080/02724634.2014.994745. Bibcode: 2015JVPal..35E4745W.
- ↑ Hoffecker, John F.; Elias, Scott A. (2012-05-29). Human Ecology of Beringia. Columbia University Press. ISBN 9780231503884. https://books.google.com/books?id=DQkpVuA7_6UC&q=baikal+yak&pg=PA56.
- ↑ Rivals, Florent (2006). "Découverte de Capra caucasica et d'Hemitragus cedrensis (Mammalia, Bovidae) dans les niveaux du Pléistocène supérieur de la Caune de l'Arago (Tautavel, France): Implication biochronologique dans le contexte du Bassin Méditerranéen". Geobios 39 (1): 85–102. doi:10.1016/j.geobios.2004.08.004. Bibcode: 2006Geobi..39...85R.
- ↑ Creégut-Bonnoure, Evelyne (12 March 2009). "Biochronologie et grands mammifères au Pléistocène moyen et supérieur en Europe occidentale: l'Apport des genres hemitragus et capra" (in fr). Quaternaire 20: 481–508. doi:10.4000/quaternaire.5345.
- ↑ Kurtén, Björn (2008). Pleistocene mammals of Europe. Aldine Transaction. ISBN 9780202309538. OCLC 751413776.
- ↑ Baryshnikov, G.; Tikhonov, A. (1994-10-01). "Notes on skulls of Pleistocene Saiga of Northern Eurasia". Historical Biology 8 (1–4): 209–234. doi:10.1080/10292389409380478.
- ↑ 35.0 35.1 35.2 35.3 Hoffecker, John F.; Elias, Scott A. (2012-05-29). Human Ecology of Beringia. Columbia University Press. ISBN 9780231503884. https://books.google.com/books?id=DQkpVuA7_6UC.
- ↑ 36.0 36.1 36.2 36.3 Vereshchagin, N. K.; Baryshnikov, G. F. (1991-01-01). "The ecological structure of the "Mammoth Fauna" in Eurasia". Annales Zoologici Fennici 28 (3/4): 253–259.
- ↑ Sanz, Montserrat; Daura, Joan; Brugal, Jean-Philip (2014-01-01). "First occurrence of the extinct deer Haploidoceros in the Iberian Peninsula in the Upper Pleistocene of the Cova del Rinoceront (Castelldefels, Barcelona)". Comptes Rendus Palevol 13 (1): 27–40. doi:10.1016/j.crpv.2013.06.005. Bibcode: 2014CRPal..13...27S.
- ↑ Rivals, Florent; Sanz, Montserrat; Daura, Joan (2016-05-01). "First reconstruction of the dietary traits of the Mediterranean deer (Haploidoceros mediterraneus) from the Cova del Rinoceront (NE Iberian Peninsula)". Palaeogeography, Palaeoclimatology, Palaeoecology 449: 101–107. doi:10.1016/j.palaeo.2016.02.014. Bibcode: 2016PPP...449..101R.
- ↑ 39.0 39.1 Elias, Scott; Mock, Cary (2013-03-25). Encyclopedia of Quaternary Science. Newnes. ISBN 9780444536426. https://books.google.com/books?id=TSTnzG6yetsC.
- ↑ "Habitat conditions for Camelus knoblochi and factors in its extinction by Vadim V. Titov". http://www.paleorostov.narod.ru/Titov_Camelus_knoblochi.pdf.
- ↑ 41.0 41.1 41.2 Foronova, I. (2006). "Late quaternary equids (genus Equus) of South-western and South-central Siberia". in M. Mashkour. Equids in time and space. Papers in honour of Véra Eisenmann. Proceedings of the 9th conference of the International Council of Archaeozoology, Durham, August 2002. Oxbow Books. pp. 20–30.
- ↑ Chase, Philip G. (2009). The Cave of Fontéchevade: Recent Excavations and Their Paleoanthropological Implications. Cambridge University Press. ISBN 9780521898447. https://books.google.com/books?id=XTPbVI5Gf6MC&q=equus+gallicus&pg=PA146.
- ↑ Yanko-Hombach, Valentina; Gilbert, Allan S.; Panin, Nicolae; Dolukhanov, Pavel M. (2006-11-15). The Black Sea Flood Question: Changes in Coastline, Climate and Human Settlement. Springer Science & Business Media. ISBN 9781402053023. https://books.google.com/books?id=sDYXosqZpegC&q=equus+latipes&pg=PA288.
- ↑ Hopkins, David M.; Matthews, John V.; Schweger, Charles E. (2013). Paleoecology of Beringia. Elsevier Science. p. 349. ISBN 9781483273402. https://books.google.com/books?id=ZxolBQAAQBAJ&q=equus+lenensis&pg=PA349.
- ↑ Lu, Dan; Yang, Yangheshan; Li, Qiang; Ni, Xijun (2021-07-30). "A late Pleistocene fossil from Northeastern China is the first record of the dire wolf (Carnivora: Canis dirus) in Eurasia". Quaternary International. Cave Deposits from Luotuo Hill, Northeast China: A Geochronologically Calibrated Mammalian Biostratigraphic Standard for the Quaternary of Eastern Asia 591: 87–92. doi:10.1016/j.quaint.2020.09.054. ISSN 1040-6182. Bibcode: 2021QuInt.591...87L.
- ↑ Marciszak, Adrian; Schouwenburg, Charles; Lipecki, Grzegorz; Talamo, Sahra; Shpansky, Andrey; Malikov, Dmitriy; Gornig, Wiktoria (2019-12-01). "Steppe brown bear Ursus arctos "priscus" from the Late Pleistocene of Europe". Quaternary International. Quaternary Stratigraphy and Hominids Around Europe:: SEQS 2017 Meeting 534: 158–170. doi:10.1016/j.quaint.2019.02.042. ISSN 1040-6182. Bibcode: 2019QuInt.534..158M.
- ↑ Münzel, Susanne C.; Rivals, Florent; Pacher, Martina; Döppes, Doris; Rabeder, Gernot; Conard, Nicholas J.; Bocherens, Hervé (2014-08-07). "Behavioural ecology of Late Pleistocene bears (Ursus spelaeus, Ursus ingressus): Insight from stable isotopes (C, N, O) and tooth microwear". Quaternary International. Fossil remains in karst and their role in reconstructing Quaternary paleoclimate and paleoenvironments 339–340: 148–163. doi:10.1016/j.quaint.2013.10.020. Bibcode: 2014QuInt.339..148M.
- ↑ Ghezzo, Elena; Boscaini, Alberto; Madurell-Malapeira, Joan; Rook, Lorenzo (2014-12-16). "Lynx remains from the Pleistocene of Valdemino cave (Savona, Northwestern Italy), and the oldest occurrence of Lynx spelaeus (Carnivora, Felidae)". Rendiconti Lincei 26 (2): 87–95. doi:10.1007/s12210-014-0363-4.
- ↑ "Search for images at Natural History Museum Picture Library". http://piclib.nhm.ac.uk/results.asp.
- ↑ Stuart, Anthony John (May 2015). "Late Quaternary megafaunal extinctions on the continents: a short review: LATE QUATERNARY MEGAFAUNAL EXTINCTIONS" (in en). Geological Journal 50 (3): 338–363. doi:10.1002/gj.2633.
- ↑ Vershinina, Alisa O.; Heintzman, Peter D.; Froese, Duane G.; Zazula, Grant; Cassatt‐Johnstone, Molly; Dalén, Love; Der Sarkissian, Clio; Dunn, Shelby G. et al. (December 2021). "Ancient horse genomes reveal the timing and extent of dispersals across the Bering Land Bridge" (in en). Molecular Ecology 30 (23): 6144–6161. doi:10.1111/mec.15977. ISSN 0962-1083. PMID 33971056. Bibcode: 2021MolEc..30.6144V.
- ↑ Cirilli, Omar; Machado, Helena; Arroyo-Cabrales, Joaquin; Barrón-Ortiz, Christina I.; Davis, Edward; Jass, Christopher N.; Jukar, Advait M.; Landry, Zoe et al. (2022-08-24). "Evolution of the Family Equidae, Subfamily Equinae, in North, Central and South America, Eurasia and Africa during the Plio-Pleistocene" (in en). Biology 11 (9): 1258. doi:10.3390/biology11091258. ISSN 2079-7737. PMID 36138737.
- ↑ Mitchell, Kieren J.; Bover, Pere; Salis, Alexander T.; Mudge, Caitlin; Heiniger, Holly; Thompson, Mary; Hockett, Bryan; Weyrich, Laura S. et al. (2023-02-20). "Evidence for Pleistocene gene flow through the ice-free corridor from extinct horses and camels from Natural Trap Cave, Wyoming" (in en). Quaternary International 647-648: 71–80. doi:10.1016/j.quaint.2021.11.017.
- ↑ Lundelius, Ernest L; Bryant, Vaughn M; Mandel, Rolfe; Thies, Kenneth J; Thoms, Alston (2013). "The First Occurrence of a Toxodont (Mammalia, Notoungulata) in the United States". Journal of Vertebrate Paleontology 33 (1): 229–232. doi:10.1080/02724634.2012.711405. Bibcode: 2013JVPal..33..229L. https://www.researchgate.net/publication/260140036. Retrieved 2016-01-23.
- ↑ Rodriguez-de la Rosa, Ruben A.; Guzman Gutierrez, Jose Ruben; Mendoza, Carlos (December 2001). "A New Occurrence of Toxodonts in the Pleistocene of México". Current Research in the Pleistocene 28: 29–30. https://www.researchgate.net/publication/230820008. Retrieved 2016-01-23.
- ↑ "Fossilworks: Lynx lynx". http://www.fossilworks.org/cgi-bin/bridge.pl?a=taxonInfo&taxon_no=160980.
- ↑ 57.0 57.1 Schubert, Blaine W.; Chatters, James C.; Arroyo-Cabrales, Joaquin; Samuels, Joshua X.; Soibelzon, Leopoldo H.; Prevosti, Francisco J.; Widga, Christopher; Nava, Alberto et al. (2019-05-31). "Yucatán carnivorans shed light on the Great American Biotic Interchange". Biology Letters 15 (5): 20190148. doi:10.1098/rsbl.2019.0148. PMID 31039726.
- ↑ Youngman, Phillip M. (1986-03-01). "The extinct short-faced skunk Brachyprotoma obtusata (Mammalia, Carnivora): first records for Canada and Beringia". Canadian Journal of Earth Sciences 23 (3): 419–424. doi:10.1139/e86-043. Bibcode: 1986CaJES..23..419Y.
- ↑ Anderson, E. (1973). "Ferrets from the pleistocene of central Alaska". J. Mammal. 54 (3): 778–779. doi:10.2307/1378982.
- ↑ 60.00 60.01 60.02 60.03 60.04 60.05 60.06 60.07 60.08 60.09 60.10 Sanz, Nuria (31 December 2015). Human origin sites and the World Heritage Convention in Americas. 1. Paris: UNESCO. ISBN 9789231001406. OCLC 1002234186.
- ↑ Ceballos, Gerardo; Arroyo-Cabrales, Joaquín; Ponce, Eduardo (2010). "Effects of Pleistocene environmental changes on the distribution and community structure of the mammalian fauna of Mexico". Quaternary Research 73 (3): 464–473. doi:10.1016/j.yqres.2010.02.006. Bibcode: 2010QuRes..73..464C. https://www.researchgate.net/publication/220048614.
- ↑ Lucas, Spencer G.; Morgan, Gary S.; Spielmann, Justin A.; Prothero, Donald R. (2008). Neogene Mammals: Bulletin 44. New Mexico Museum of Natural History and Science. https://books.google.com/books?id=vW_aBwAAQBAJ&q=giant+anteater+late+pleistocene+honduras&pg=PA119.
- ↑ Sanchez, Guadalupe; Holliday, Vance T.; Gaines, Edmund P.; Arroyo-Cabrales, Joaquín; Martínez-Tagüeña, Natalia; Kowler, Andrew; Lange, Todd; Hodgins, Gregory W. L. et al. (2014-07-29). "Human (Clovis)–gomphothere (Cuvieronius sp.) association ~13,390 calibrated yBP in Sonora, Mexico". Proceedings of the National Academy of Sciences 111 (30): 10972–10977. doi:10.1073/pnas.1404546111. PMID 25024193. Bibcode: 2014PNAS..11110972S.
- ↑ "Fossilworks: Erethizon kleini". http://www.fossilworks.org/cgi-bin/bridge.pl?a=taxonInfo&taxon_no=46322.
- ↑ McDonald, H. Gregory; Chatters, James C.; Gaudin, Timothy J. (2017-05-04). "A new genus of megalonychid ground sloth (Mammalia, Xenarthra) from the late Pleistocene of Quintana Roo, Mexico". Journal of Vertebrate Paleontology 37 (3): e1307206. doi:10.1080/02724634.2017.1307206. ISSN 0272-4634. Bibcode: 2017JVPal..37E7206M.
- ↑ "Ice Age Predators Found Alongside Oldest Human in Americas". 2017-08-25. http://news.nationalgeographic.com/2017/08/ice-age-fossils-underwater-cave-bears-humans-science/.
- ↑ Stinnesbeck, Sarah R.; Frey, Eberhard; Olguín, Jerónimo Avíles; Stinnesbeck, Wolfgang; Zell, Patrick; Mallison, Heinrich; González, Arturo González; Núñez, Eugenio Aceves et al. (2017-06-01). "Xibalbaonyx oviceps, a new megalonychid ground sloth (Folivora, Xenarthra) from the Late Pleistocene of the Yucatán Peninsula, Mexico, and its paleobiogeographic significance". PalZ 91 (2): 245–271. doi:10.1007/s12542-017-0349-5. ISSN 0031-0220.
- ↑ "Ancient Giant Sloth Fossil Found in Underwater Cave". 2017-08-18. http://news.nationalgeographic.com/2017/08/ancient-giant-sloth-new-species-mexico-cave-spd/.
- ↑ Debus, Allen (June 2002). Dinosaur Memories. iUniverse. ISBN 9780595229888. https://books.google.com/books?id=oVl_ILVlbgIC&q=Doedicurus+habitat&pg=PA196.
- ↑ McDonough, Colleen M.; Loughry, W. J. (2013-03-18). The Nine-Banded Armadillo: A Natural History. University of Oklahoma Press. ISBN 9780806189215. https://books.google.com/books?id=h4UFvagbbogC.
- ↑ Zicha, Ondrej. "BioLib: Biological library". https://www.biolib.cz/en/taxon/id476718/.
- ↑ "Fossilworks: Phoenicopterus copei". http://www.fossilworks.org/cgi-bin/bridge.pl?a=taxonInfo&taxon_no=335545.
- ↑ Feduccia, Alan (1999). The Origin and Evolution of Birds. Yale University Press. ISBN 978-0300078619. https://books.google.com/books?id=8QRKV7eSqmIC&q=Neophrontops&pg=PA300.
- ↑ Gillespie, Rosemary G.; Clague, D. A. (2009). Encyclopedia of Islands. University of California Press. ISBN 9780520256491. https://books.google.com/books?id=g9ZogGs_fz8C&q=Gigantohierax+suarezi&pg=PA321.
- ↑ Turvey, Sam (2009-05-28). Holocene Extinctions. Oxford: Oxford University Press. ISBN 9780199535095. https://books.google.com/books?id=4QIUDAAAQBAJ&q=Gigantohierax+suarezi&pg=PA74.
- ↑ MacPhee, R.D.E. (1999). Extinctions in Near Time: Causes, Contexts, and Consequences. Kluwer Academic Publishers. ISBN 978-0-306-46092-0.[page needed]
- ↑ Bell, C.J. (2004). "The Blancan, Irvingtonian, and Rancholabrean mammal ages". in Woodburne, M.O.. Late Cretaceous and Cenozoic Mammals of North America: Biostratigraphy and Geochronology. New York: Columbia Univ. Press. pp. 232–314. ISBN 978-0-231-13040-0.
- ↑ Scott, E.; Cox, S.M. (2008). "Late Pleistocene distribution of Bison (Mammalia; Artiodactyla) in the Mojave Desert of Southern California and Nevada". in Wang, X.. Geology and Vertebrate Paleontology of Western and Southern North America. Los Angeles: Natural History Museum of Los Angeles County. pp. 359–382.
- ↑ Sanders, A.E.; R.E. Weems; L.B. Albright III (2009). "Formalization of the mid-Pleistocene "Ten Mile Hill beds" in South Carolina with evidence for placement of the Irvingtonian–Rancholabrean boundary". in Albright III, L.B.. Papers on Geology, Vertebrate Paleontology, and Biostratigraphy in Honor of Michael O. Woodburne. Flagstaff: Museum of Northern Arizona. pp. 369–375.
- ↑ Shapiro, B. (2004). "Rise and Fall of the Beringian Steppe Bison". Science 306 (5701): 1561–1565. doi:10.1126/science.1101074. PMID 15567864. Bibcode: 2004Sci...306.1561S. http://summit.sfu.ca/item/15088.
- ↑ Wilson, M.C.; L.V. Hills; B. Shapiro (2008). "Late Pleistocene northward-dispersing Bos antiquus from the Bighill Creek Formation, Gallelli Gravel Pit, Alberta, Canada, and the fate of Bison occidentalis". Canadian Journal of Earth Sciences 45 (7): 827–859. doi:10.1139/E08-027. Bibcode: 2008CaJES..45..827W.
- ↑ Prates, Luciano; Perez, S. Ivan (12 April 2021). "Late Pleistocene South American megafaunal extinctions associated with rise of Fishtail points and human population" (in en). Nature Communications 12 (1): 2175. doi:10.1038/s41467-021-22506-4. ISSN 2041-1723. PMID 33846353. Bibcode: 2021NatCo..12.2175P.
- ↑ Iriarte, José; Ziegler, Michael J.; Outram, Alan K.; Robinson, Mark; Roberts, Patrick; Aceituno, Francisco J.; Morcote-Ríos, Gaspar; Keesey, T. Michael (25 April 2022). "Ice Age megafauna rock art in the Colombian Amazon?" (in en). Philosophical Transactions of the Royal Society B: Biological Sciences 377 (1849). doi:10.1098/rstb.2020.0496. ISSN 0962-8436. PMID 35249392. PMC 8899627. https://royalsocietypublishing.org/doi/10.1098/rstb.2020.0496. Retrieved 2 January 2024.
- ↑ Dantas, Mário André Trindade; Santos, Daivisson Batista; Liparini, Alexandre; Queiroz, Albérico Nogueira de; Carvalho, Olivia Alexandre de; Castro, Érika Sousa Vieira de; Cherkinsky, Alexander (26 November 2014). "Dated evidence of the interaction between humans and megafauna in the late Pleistocene of Sergipe state, northeastern Brazil". Quaternary International. Quaternary Investigations from Antarctica across South America to the North Atlantic 352: 197–199. doi:10.1016/j.quaint.2014.09.040. ISSN 1040-6182. https://www.sciencedirect.com/science/article/pii/S1040618214006922. Retrieved 2 January 2024.
- ↑ "Fossilworks: Agalmaceros". http://www.fossilworks.org/cgi-bin/bridge.pl?a=taxonInfo&taxon_no=247645.
- ↑ "Fossilworks: Agalmaceros blicki". http://www.fossilworks.org/cgi-bin/bridge.pl?a=taxonInfo&taxon_no=247647.
- ↑ "Fossilworks: Odocoileus salinae". http://www.fossilworks.org/cgi-bin/bridge.pl?a=taxonInfo&taxon_no=247644.
- ↑ 88.0 88.1 Turvey, Samuel T. (2009-05-28). Holocene Extinctions. Oxford University Press. ISBN 9780191579981. https://books.google.com/books?id=mbU-F42JU1AC&q=Propaopus&pg=PA349.
- ↑ 89.0 89.1 MacFadden, Bruce J. (2013-03-20). "Dispersal of Pleistocene Equus (Family Equidae) into South America and Calibration of GABI 3 Based on Evidence from Tarija, Bolivia". PLOS ONE 8 (3): e59277. doi:10.1371/journal.pone.0059277. ISSN 1932-6203. PMID 23527150. Bibcode: 2013PLoSO...859277M.
- ↑ Pereira, Jamil Corrêa; Lopes, Renato Pereira; Kerber, Leonardo (2012). "New remains of Late Pleistocene mammals from the Chuí Creek, Southern Brazil". Revista Brasileira de Paleontologia 15 (2): 228–239. doi:10.4072/rbp.2012.2.10.
- ↑ Ransom, Jason I.; Kaczensky, Petra (2016-05-15). Wild Equids: Ecology, Management, and Conservation. JHU Press. ISBN 9781421419107. https://books.google.com/books?id=2vL-CwAAQBAJ&q=hippidion+california&pg=PA109.
- ↑ "Late Pleistocene vertebrates from Touro Passo Creek (Touro Passo Formation), southern Brazil: A review". Revista Mexicana de Ciencias Geológicas 31 (2): 248–259. https://www.researchgate.net/publication/265013705. Retrieved 2017-09-02.
- ↑ "Fossilworks: Macraucheniopsis". http://www.fossilworks.org/cgi-bin/bridge.pl?a=taxonInfo&taxon_no=43498.
- ↑ Gaudioso, Pablo Javier; Gasparini, Germán M.; Herbst, Rafael; Barquez, Rubén Mario (2017-03-16). "First record of the Neolicaphrium recens Frenguelli, 1921 (Mammalia, Litopterna) in the Pleistocene of Santiago del Estero Province, Argentina". Papéis Avulsos de Zoologia 57 (3): 23–29. doi:10.11606/0031-1049.2017.57.03. ISSN 1807-0205.
- ↑ Kurtén, Björn; Werdelin, Lars (1990). "Relationships between North and South American Smilodon". Journal of Vertebrate Paleontology 10 (2): 158–169. doi:10.1080/02724634.1990.10011804. Bibcode: 1990JVPal..10..158K.
- ↑ Chimento, Nicolás R.; Agnolin, Federico L. (November 2017). "The fossil American lion ( Panthera atrox ) in South America: Palaeobiogeographical implications" (in en). Comptes Rendus Palevol 16 (8): 850–864. doi:10.1016/j.crpv.2017.06.009. Bibcode: 2017CRPal..16..850C.
- ↑ Prevosti, F. J.; Tonni, E. P.; Bidegain, J. C. (2009-12-01). "Stratigraphic range of the large canids (Carnivora, Canidae) in South America, and its relevance to quaternary biostratigraphy". Quaternary International. The Ensenadan Stage/Age in southern South America 210 (1): 76–81. doi:10.1016/j.quaint.2009.06.034. ISSN 1040-6182. Bibcode: 2009QuInt.210...76P.
- ↑ "Fossilworks: Valgipes". http://www.fossilworks.org/cgi-bin/bridge.pl?a=taxonInfo&taxon_no=137597.
- ↑ Haynes, Gary (2008-12-23). American Megafaunal Extinctions at the End of the Pleistocene. Springer Science & Business Media. ISBN 9781402087936. https://books.google.com/books?id=iq6qZXUkWo0C&q=Arctotherium+bonariense&pg=PA126.
- ↑ Prothero, Donald R. (2016-11-15). The Princeton Field Guide to Prehistoric Mammals. Princeton University Press. ISBN 9781400884452. https://books.google.com/books?id=eiftDAAAQBAJ&q=Doedicurus+habitat&pg=PA54.
- ↑ Eisenberg, John F.; Redford, Kent H. (2000-05-15). Mammals of the Neotropics, Volume 3: Ecuador, Bolivia, Brazil. University of Chicago Press. ISBN 9780226195421. https://books.google.com/books?id=p2MDAzCeQQoC&q=Doedicurus+habitat&pg=PA17.
- ↑ Fariña, Richard A.; Vizcaíno, Sergio F.; Iuliis, Gerry De (2013-05-22). Megafauna: Giant Beasts of Pleistocene South America. Indiana University Press. ISBN 978-0253007193. https://books.google.com/books?id=kUAKgNfiAvoC&q=Doedicurus+habitat&pg=PA167.
- ↑ 103.0 103.1 103.2 Oliveira, Édison V.; Porpino, Kleberson O.; Barreto, Alcina F. (2010). "On the presence of Glyptotherium in the Late Pleistocene of Northeastern Brazil, and the status of "Glyptodon" and "Chlamydotherium". Paleobiogeographic implications". Neues Jahrbuch für Geologie und Paläontologie - Abhandlungen 258 (3): 353–363. doi:10.1127/0077-7749/2010/0116.
- ↑ Martin, Paul Schultz (2005). Twilight of the Mammoths: Ice Age Extinctions and the Rewilding of America. University of California Press. ISBN 9780520231412. https://archive.org/details/twilightofmammot00paul.
- ↑ "Fossilworks: Neuryurus". http://www.fossilworks.org/cgi-bin/bridge.pl?a=taxonInfo&taxon_no=43582.
- ↑ "Fossilworks: Parapanochthus". http://www.fossilworks.org/cgi-bin/bridge.pl?a=taxonInfo&taxon_no=188888.
- ↑ Martin, Robert A.; Martin, Robert Allen; Barnosky, Anthony D. (2005-10-06). Morphological Change in Quaternary Mammals of North America. Cambridge University Press. ISBN 9780521020817. https://books.google.com/books?id=heAoqlZldWoC&q=chlamytherium+occidentale&pg=PA135.
- ↑ Turvey, Sam (2009-05-28). Holocene Extinctions. Oxford: Oxford University Press. ISBN 9780199535095. https://books.google.com/books?id=4QIUDAAAQBAJ&q=chlamytherium+occidentale&pg=PA341.
- ↑ 109.0 109.1 Góis, Flávio; Ruiz, Laureano Raúl González; Scillato-Yané, Gustavo Juan; Soibelzon, Esteban (2015-06-17). "A Peculiar New Pampatheriidae (Mammalia: Xenarthra: Cingulata) from the Pleistocene of Argentina and Comments on Pampatheriidae Diversity". PLOS ONE 10 (6): e0128296. doi:10.1371/journal.pone.0128296. PMID 26083486. Bibcode: 2015PLoSO..1028296G.
- ↑ Jones, Washington; Rinderknecht, Andrés; Alvarenga, Herculano; Montenegro, Felipe; Ubilla, Martín (2017-12-30). "The last terror birds (Aves, Phorusrhacidae): new evidence from the late Pleistocene of Uruguay". PalZ 92 (2): 365–372. doi:10.1007/s12542-017-0388-y. ISSN 0031-0220.
- ↑ Alvarenga, Herculano; Jones, Washington; Rinderknecht, Andrés (2010-05-01). "The youngest record of phorusrhacid birds (Aves, Phorusrhacidae) from the late Pleistocene of Uruguay". Neues Jahrbuch für Geologie und Paläontologie, Abhandlungen 256 (2): 229–234. doi:10.1127/0077-7749/2010/0052.
- ↑ Agnolin, Federico (2013). "La posición sistemática de Hermosiornis (Aves, Phororhacoidea) y sus implicancias filogenéticas". Revista del Museo Argentino de Ciencias Naturales. Nueva Series 15 (1): 39–60. doi:10.22179/revmacn.15.167. ISSN 1853-0400.
- ↑ Jones, Washington; Rinderknecht, Andrés; Migotto, Rafael; Blanco, R. Ernesto (2013). "Body Mass Estimations and Paleobiological Inferences on a New Species of Large Caracara (Aves, Falconidae) from the Late Pleistocene of Uruguay". Journal of Paleontology 87 (1): 151–158. doi:10.1666/12-026R.1. Bibcode: 2013JPal...87..151J. https://www.researchgate.net/publication/260104736.
- ↑ Suárez, William; Olson, Storrs L. (2014-09-01). "A new fossil species of small crested caracara (Aves: Falconidae: Caracara) from the Pacific lowlands of western South America". Proceedings of the Biological Society of Washington 127 (2): 299–310. doi:10.2988/0006-324X-127.2.299. ISSN 0006-324X.
- ↑ "Fossilworks: Milvago brodkorbi". http://www.fossilworks.org/cgi-bin/bridge.pl?a=taxonInfo&taxon_no=289782.
- ↑ David, Bruno; Arnold, Lee J.; Delannoy, Jean-Jacques; Fresløv, Joanna; Urwin, Chris; Petchey, Fiona; McDowell, Matthew C.; Mullett, Russell et al. (2021-02-01). "Late survival of megafauna refuted for Cloggs Cave, SE Australia: Implications for the Australian Late Pleistocene megafauna extinction debate" (in en). Quaternary Science Reviews 253: 106781. doi:10.1016/j.quascirev.2020.106781. ISSN 0277-3791. Bibcode: 2021QSRv..25306781D.
- ↑ Price, Gilbert J.; Zhao, Jian-xin; Feng, Yue-Xing; Hocknull, Scott A. (2009-02-01). "New U/Th ages for Pleistocene megafauna deposits of southeastern Queensland, Australia" (in en). Journal of Asian Earth Sciences 34 (2): 190–197. doi:10.1016/j.jseaes.2008.04.008. ISSN 1367-9120. Bibcode: 2009JAESc..34..190P.
- ↑ "Humans, not climate change, wiped out Australian megafauna". 2017-01-20. https://www.colorado.edu/today/2017/01/20/humans-not-climate-change-wiped-out-australian-megafauna.
- ↑ Wilson, Lito Vilisoni (2020-05-18). "Mysteries of megafauna extinction unlocked". https://about.unimelb.edu.au/newsroom/news/2020/may/mysteries-of-megafauna-extinction-unlocked.
- ↑ Hocknull, Scott A.; Lewis, Richard; Arnold, Lee J.; Pietsch, Tim; Joannes-Boyau, Renaud; Price, Gilbert J.; Moss, Patrick; Wood, Rachel et al. (2020-05-18). "Extinction of eastern Sahul megafauna coincides with sustained environmental deterioration". Nature Communications 11 (1): 2250. doi:10.1038/s41467-020-15785-w. ISSN 2041-1723. PMID 32418985. Bibcode: 2020NatCo..11.2250H.
- ↑ 121.0 121.1 Saltré, Frédérik; Chadoeuf, Joël; Peters, Katharina J.; McDowell, Matthew C.; Friedrich, Tobias; Timmermann, Axel; Ulm, Sean; Bradshaw, Corey J. A. (2019-11-22). "Climate-human interaction associated with southeast Australian megafauna extinction patterns". Nature Communications 10 (1): 5311. doi:10.1038/s41467-019-13277-0. ISSN 2041-1723. PMID 31757942. Bibcode: 2019NatCo..10.5311S.
- ↑ DeSantis, Larisa R. G.; Field, Judith H.; Wroe, Stephen; Dodson, John R. (2017-01-26). "Dietary responses of Sahul (Pleistocene Australia–New Guinea) megafauna to climate and environmental change". Paleobiology 43 (2): 181–195. doi:10.1017/pab.2016.50. ISSN 0094-8373. Bibcode: 2017Pbio...43..181D.
- ↑ 123.0 123.1 Lourandos, Harry (1997-02-28). Continent of Hunter-Gatherers: New Perspectives in Australian Prehistory. Cambridge University Press. ISBN 9780521359467. https://books.google.com/books?id=tTy-I8no1MwC&q=Phascolomys+medius&pg=PA99.
- ↑ 124.0 124.1 MacPhee, R.D.E. (1999). Extinctions in Near Time: Causes, Contexts, and Consequences. Kluwer Academic Publishers. p. 251. ISBN 978-0-306-46092-0. https://books.google.com/books?id=UZLuF1YXYTcC&pg=PA251.
- ↑ Long, John A.; Archer, Michael (2002). Prehistoric Mammals of Australia and New Guinea: One Hundred Million Years of Evolution. UNSW Press. ISBN 9780868404356. https://books.google.com/books?id=LwMkO0M1mPQC&q=Warendja+wakefieldi&pg=PA116.
- ↑ Tyndale-Biscoe, Hugh (2005-04-22). Life of Marsupials. Csiro Publishing. ISBN 9780643099210. https://books.google.com/books?id=YALY-B6s7IEC&q=Warendja+wakefieldi&pg=PA269.
- ↑ Marwick, Ben. "Buried tools and pigments tell a new history of humans in Australia for 65,000 years". The Conversation. https://theconversation.com/buried-tools-and-pigments-tell-a-new-history-of-humans-in-australia-for-65-000-years-81021.
- ↑ Webb, Steve (2013-02-27). Corridors to Extinction and the Australian Megafauna. Newnes. ISBN 9780124078406. https://books.google.com/books?id=C0blLvmdp04C&q=Phascolomys+medius&pg=PA279.
- ↑ "Megafauna". http://austhrutime.com/marsupial_megafauna.htm.
- ↑ "Anaspides.net". http://www.anaspides.net/earth_life_sciences/megafauna_extinction.html.
- ↑ Webb, Steve (2013-02-27). Corridors to Extinction and the Australian Megafauna. Newnes. ISBN 9780124078406. https://books.google.com/books?id=C0blLvmdp04C&q=Warendja+wakefieldi&pg=PA279.
- ↑ Long, John A.; Archer, Michael (2002). Prehistoric Mammals of Australia and New Guinea: One Hundred Million Years of Evolution. UNSW Press. ISBN 9780868404356. https://books.google.com/books?id=LwMkO0M1mPQC&q=Troposodon+minor&pg=PA163.
- ↑ White, Lauren C.; Saltré, Frédérik; Bradshaw, Corey J. A.; Austin, Jeremy J. (January 2018). "High-quality fossil dates support a synchronous, Late Holocene extinction of devils and thylacines in mainland Australia" (in en). Biology Letters 14 (1): 20170642. doi:10.1098/rsbl.2017.0642. ISSN 1744-9561. PMID 29343562.
- ↑ Bayly, I. A. E. (1993). "The fauna of athalassic saline waters in Australia and the Altiplano of South America: Comparisons and historical perspectives". in Hurlbert, Stuart H.. Saline Lakes V. Developments in Hydrobiology. 87. Springer Netherlands. pp. 225–231. doi:10.1007/978-94-011-2076-0_18. ISBN 9789401049214.
- ↑ Doughty, C. E.; Wolf, A.; Field, C. B. (2010). "Biophysical feedbacks between the Pleistocene megafauna extinction and climate: The first human‐induced global warming?". Geophys. Res. Lett. 37 (15): L15703. doi:10.1029/2010GL043985. Bibcode: 2010GeoRL..3715703D.
- ↑ Grayson, Donald K.; Meltzer, David J. (December 2012). "Clovis Hunting and Large Mammal Extinction: A Critical Review of the Evidence". Journal of World Prehistory 16 (4): 313–359. doi:10.1023/A:1022912030020.
- ↑ Kolbert, Elizabeth (2014). The Sixth Extinction: An Unnatural History. Bloomsbury Publishing. ISBN 9781408851210.
- ↑ Perry, George L. W.; Wheeler, Andrew B.; Wood, Jamie R.; Wilmshurst, Janet M. (2014-12-01). "A high-precision chronology for the rapid extinction of New Zealand moa (Aves, Dinornithiformes)". Quaternary Science Reviews 105: 126–135. doi:10.1016/j.quascirev.2014.09.025. Bibcode: 2014QSRv..105..126P.
- ↑ Crowley, Brooke E. (2010-09-01). "A refined chronology of prehistoric Madagascar and the demise of the megafauna". Quaternary Science Reviews. Special Theme: Case Studies of Neodymium Isotopes in Paleoceanography 29 (19–20): 2591–2603. doi:10.1016/j.quascirev.2010.06.030. Bibcode: 2010QSRv...29.2591C.
- ↑ Li, Sophia (2012-09-20). "Has Plant Life Reached Its Limits?". http://green.blogs.nytimes.com/2012/09/20/has-plant-life-reached-its-limits/?_r=0.
- ↑ Martin, P. S. (1963). The last 10,000 years: A fossil pollen record of the American Southwest. Tucson, AZ: Univ. Ariz. Press. ISBN 978-0-8165-1759-6.
- ↑ Martin, P. S. (1967). "Prehistoric overkill". in Martin, P. S.. Pleistocene extinctions: The search for a cause. New Haven: Yale Univ. Press. ISBN 978-0-300-00755-8.
- ↑ Martin, P. S. (1989). "Prehistoric overkill: A global model". in Martin, P.S.. Quaternary extinctions: A prehistoric revolution. Tucson, AZ: Univ. Arizona Press. pp. 354–404. ISBN 978-0-8165-1100-6.
- ↑ Weston, Phoebe (25 November 2022). "Humans v nature: our long and destructive journey to the age of extinction". The Guardian. https://www.theguardian.com/environment/2022/nov/25/cop15-humans-v-nature-our-long-and-destructive-journey-to-the-age-of-extinction-aoe.
- ↑ Martin, Paul S. (1966). "Africa and Pleistocene overkill". Nature 212 (5060): 339–342. doi:10.1038/212339a0. Bibcode: 1966Natur.212..339M. http://max2.ese.u-psud.fr/epc/conservation/PDFs/HIPE/Martin1966.pdf. Retrieved 2019-07-31.
- ↑ Barnosky, Anthony D.; Lindsey, Emily L. (15 April 2010). "Timing of Quaternary megafaunal extinction in South America in relation to human arrival and climate change". Quaternary International. Faunal Dynamics and Extinction in the Quaternary: Studies in Honor of Ernest L. Lundelius, Jr. 217 (1): 10–29. doi:10.1016/j.quaint.2009.11.017. ISSN 1040-6182. https://www.sciencedirect.com/science/article/pii/S1040618209004236. Retrieved 2 January 2024.
- ↑ Surovell, Todd A.; Pelton, Spencer R.; Anderson-Sprecher, Richard; Myers, Adam D. (26 January 2016). "Test of Martin’s overkill hypothesis using radiocarbon dates on extinct megafauna" (in en). Proceedings of the National Academy of Sciences of the United States of America 113 (4): 886–891. doi:10.1073/pnas.1504020112. ISSN 0027-8424. PMID 26504205. PMC 4743775. https://pnas.org/doi/full/10.1073/pnas.1504020112. Retrieved 2 January 2024.
- ↑ Martin, P. S. (2005). Twilight of the Mammoths: Ice Age Extinctions and the Rewilding of America. University of California Press. ISBN 978-0-520-23141-2. https://archive.org/details/twilightofmammot00paul.
- ↑ Burney, D. A.; Flannery, T. F. (July 2005). "Fifty millennia of catastrophic extinctions after human contact". Trends in Ecology & Evolution 20 (7): 395–401. doi:10.1016/j.tree.2005.04.022. PMID 16701402. http://www.anthropology.hawaii.edu/Fieldschools/Kauai/Publications/Publication%204.pdf.
- ↑ "Palaeontology: The last giant kangaroo". Nature 454 (7206): 835–6. 2008. doi:10.1038/454835a. PMID 18704074. Bibcode: 2008Natur.454..835D.
- ↑ "Late-surviving megafauna in Tasmania, Australia, implicate human involvement in their extinction". Proc. Natl. Acad. Sci. U.S.A. 105 (34): 12150–3. 26 August 2008. doi:10.1073/pnas.0801360105. PMID 18719103. Bibcode: 2008PNAS..10512150T.
- ↑ "Humans responsible for demise of gigantic ancient mammals". University of Exeter. 13 August 2015. https://www.exeter.ac.uk/news/featurednews/title_465673_en.html.
- ↑ Lewis J. Bartlett; David R. Williams; Graham W. Prescott; Andrew Balmford; Rhys E. Green; Anders Eriksson; Paul J. Valdes; Joy S. Singarayer et al. (2016). "Robustness despite uncertainty: regional climate data reveal the dominant role of humans in explaining global extinctions of Late Quaternary megafauna". Ecography 39 (2): 152–161. doi:10.1111/ecog.01566. Bibcode: 2016Ecogr..39..152B. https://research-information.bristol.ac.uk/en/publications/robustness-despite-uncertainty(94b9d1da-e339-47d9-803e-fa5a64cff28c).html.
- ↑ "Humans, not climate, have driven rapidly rising mammal extinction rate". phys.org. https://phys.org/news/2020-09-humans-climate-driven-rapidly-mammal.html.
- ↑ Andermann, Tobias; Faurby, Søren; Turvey, Samuel T.; Antonelli, Alexandre; Silvestro, Daniele (September 2020). "The past and future human impact on mammalian diversity". Science Advances 6 (36): eabb2313. doi:10.1126/sciadv.abb2313. ISSN 2375-2548. PMID 32917612. Bibcode: 2020SciA....6.2313A.
Text and images are available under a Creative Commons Attribution 4.0 International License.
- ↑ Lemoine, Rhys Taylor; Buitenwerf, Robert; Svenning, Jens-Christian (1 December 2023). "Megafauna extinctions in the late-Quaternary are linked to human range expansion, not climate change". Anthropocene 44: 100403. doi:10.1016/j.ancene.2023.100403. ISSN 2213-3054.
- ↑ Smith, Felisa A. (April 20, 2018). "Body size downgrading of mammals over the late Quaternary". Science 360 (6386): 310–313. doi:10.1126/science.aao5987. PMID 29674591. Bibcode: 2018Sci...360..310S.
- ↑ The future eaters: an ecological history of the Australasian lands and people. New York: Grove/Atlantic, Inc.. 2002-10-16. ISBN 978-0-8021-3943-6. OCLC 32745413. http://google.com/books?id=eIW5aktgo0IC&printsec=frontcover.
- ↑ Diamond, J. (1984). "Historic extinctions: a Rosetta stone for understanding prehistoric extinctions". in Martin, P.S.. Quaternary extinctions: A prehistoric revolution. Tucson, AZ: Univ. Arizona Press. pp. 824–62. ISBN 978-0-8165-1100-6.
- ↑ Diamond, J. (1997). Guns, germs, and steel; the fates of human societies. New York: Norton. ISBN 978-0-393-31755-8.
- ↑ Mossiman, J. E.; Martin, P. S. (1975). "Simulating Overkill by Paleoindians". American Scientist 63 (3): 304–13. Bibcode: 1975AmSci..63..304M.
- ↑ Whittington, S. L.; Dyke, B. (1984). "Simulating overkill: experiment with the Mossiman and Martin model". in Martin, P.S.. Quaternary extinctions: A prehistoric revolution. Tucson, AZ: Univ. Arizona Press. pp. 451–66. ISBN 978-0-8165-1100-6.
- ↑ 163.0 163.1 Alroy, J. (2001). "A multispecies overkill simulation of the end-Pleistocene megafaunal mass extinction". Science 292 (5523): 1893–6. doi:10.1126/science.1059342. PMID 11397940. Bibcode: 2001Sci...292.1893A. http://www.nceas.ucsb.edu/~alroy/pdfs/2001-Science-292-1893.pdf. Retrieved 2008-08-09.
- ↑ Grayson, Donald K.; Meltzer, David J. (1 April 2015). "Revisiting Paleoindian exploitation of extinct North American mammals". Journal of Archaeological Science. Scoping the Future of Archaeological Science: Papers in Honour of Richard Klein 56: 177–193. doi:10.1016/j.jas.2015.02.009. ISSN 0305-4403. https://www.sciencedirect.com/science/article/pii/S0305440315000473. Retrieved 2 January 2024.
- ↑ Grayson, Donald K.; Meltzer, David J.; Breslawski, Ryan P. (1 April 2021). "Overkill and the North American archaeological record—not guilty by association? A comment on Wolfe and Broughton (2020)". Journal of Archaeological Science 128: 105312. doi:10.1016/j.jas.2020.105312. ISSN 0305-4403. https://www.sciencedirect.com/science/article/pii/S0305440320302326. Retrieved 2 January 2024.
- ↑ Meltzer, David J. (21 October 2015). "Pleistocene Overkill and North American Mammalian Extinctions" (in en). Annual Review of Anthropology 44 (1): 33–53. doi:10.1146/annurev-anthro-102214-013854. ISSN 0084-6570.
- ↑ Grayson, D.K.; Meltzer, D.J. (2003). "A requiem for North American overkill". Journal of Archaeological Science 30 (5): 585–593. doi:10.1016/s0305-4403(02)00205-4. Bibcode: 2003JArSc..30..585G.
- ↑ Fiedel, S.; Haynes, G. (2004). "A premature burial: comments on Grayson and Meltzer's Requiem for overkill". Journal of Archaeological Science 31 (1): 121–131. doi:10.1016/j.jas.2003.06.004. Bibcode: 2004JArSc..31..121F.
- ↑ Surovell, Todd A; Brigid S Grund (2012). "The associational critique of Quaternary overkill". American Antiquity 77 (4): 673–688. doi:10.7183/0002-7316.77.4.672.
- ↑ Nadasdy, Paul (2006). "Transcending the Debate over the Ecologically Noble Indian: Indigenous Peoples and Environmentalism". Ethnohistory 52 (2): 291–331. doi:10.1215/00141801-52-2-291.
- ↑ Whitney-Smith, E. (2004). "Late Pleistocene extinctions through second-order predation". in Barton, C.M.. Settlement of the American Continents: A Multidisciplinary Approach to Human Biogeography. Tucson, AZ: University of Arizona Press. ISBN 978-0-8165-2323-8.
- ↑ Whitney-Smith, E. (2009). The Second-Order Predation Hypothesis of Pleistocene Extinctions: A System Dynamics Model. Saarbruken, Germany: VDM Verlag. ISBN 978-3-639-11579-6.
- ↑ 173.0 173.1 Whitney-Smith, E. (2006). Clovis and Extinctions – Overkill, Second Order Predation, Environmental Degradation in a Non-equilibrium Ecosystem "Clovis Age Continent". University of New Mexico Press.
- ↑ Scott, E. (2010). "Extinctions, scenarios, and assumptions: Changes in latest Pleistocene large herbivore abundance and distribution in western North America". Quat. Int.
- ↑ Fløjgaard, Camilla; Pedersen, Pil Birkefeldt Møller; Sandom, Christopher J.; Svenning, Jens‐Christian; Ejrnæs, Rasmus (January 2022). "Exploring a natural baseline for large‐herbivore biomass in ecological restoration". Journal of Applied Ecology 59 (1): 18–24. doi:10.1111/1365-2664.14047. ISSN 0021-8901.
- ↑ O’Keefe, F. Robin; Dunn, Regan E.; Weitzel, Elic M.; Waters, Michael R.; Martinez, Lisa N.; Binder, Wendy J.; Southon, John R.; Cohen, Joshua E. et al. (18 August 2023). "Pre–Younger Dryas megafaunal extirpation at Rancho La Brea linked to fire-driven state shift" (in en). Science 381 (6659). doi:10.1126/science.abo3594. ISSN 0036-8075. https://www.science.org/doi/10.1126/science.abo3594. Retrieved 2 January 2024.
- ↑ 177.0 177.1 Rabanus-Wallace, M. Timothy; Wooller, Matthew J.; Zazula, Grant D.; Shute, Elen; Jahren, A. Hope; Kosintsev, Pavel; Burns, James A.; Breen, James et al. (2017). "Megafaunal isotopes reveal role of increased moisture on rangeland during late Pleistocene extinctions". Nature Ecology & Evolution 1 (5): 0125. doi:10.1038/s41559-017-0125. PMID 28812683.
- ↑ Birks, H.H. (1973). "Modern macrofossil assemblages in lake sediments in Minnesota". in Birks, H.J.B.. Oxford: Blackwell Scientific. ISBN 0-632-09120-7.
- ↑ Birks, H.J.B.; Birks, H.H. (1980). Quaternary paleoecology. Baltimore: Univ. Park Press. ISBN 978-1-930665-56-9.
- ↑ Davis, M. B. (1976). "Pleistocene biogeography of temperate deciduous forests". Geoscience and man: Ecology of the Pleistocene. 13. Baton Rouge: School of Geoscience, Louisiana State Univ..
- ↑ Guthrie, R. D. (1988). Frozen Fauna of the Mammoth Steppe: The Story of Blue Babe. University Of Chicago Press. ISBN 978-0-226-31122-7.
- ↑ Guthrie, R. D. (1989). "Mosaics, allochemics, and nutrients: an ecological theory of Late Pleistocene megafaunal extinctions". in Martin, P.S.. Quaternary extinctions: A prehistoric revolution. Tucson, AZ: Univ. Arizona Press. pp. 259–99. ISBN 978-0-8165-1100-6.
- ↑ Hoppe, P.P. (1978). "Rumen fermentation in African ruminants". Atlanta.
- ↑ Bryson, R.A.; Baerreis, D.A.; Wendland, W.M. (1970). "The character of late-glacial and post-glacial climatic changes". in Dort Jr., W.. Pleistocene and recent environments of the central Great Plains. Lawrence: Univ. Press Kan. Univ. Kan. Spec. Pub. 3. ISBN 978-0-7006-0063-2. https://archive.org/details/pleistocenerecen0000symp.
- ↑ Graham, R.W.; Lundelius, E.L. (1989). "Coevolutionary disequilibrium and Pleistocene extinctions". in Martin, P.S.. Quaternary extinctions: A prehistoric revolution. Tucson, AZ: Univ. Arizona Press. pp. 354–404. ISBN 978-0-8165-1100-6.
- ↑ King, J.E.; Saunders, J.J. (1989). "Environmental insularity and the extinction of the American mastodont". in Martin, P.S.. Quaternary extinctions: A prehistoric revolution. Tucson, AZ: Univ. Arizona Press. pp. 354–404. ISBN 978-0-8165-1100-6.
- ↑ Axelrod, D. I. (1967). "Quaternary extinctions of large mammals". University of California Publications in Geological Sciences 74: 1–42. ASIN B0006BX8LG.
- ↑ Slaughter, B. H. (1967). "Animal ranges as a clue to late-Pleistocene extinction". in Martin, P.S.. Pleistocene extinctions: The search for a cause. New Haven: Yale Univ. Press. ISBN 978-0-300-00755-8.
- ↑ Kilti, R. A. (1988). "Seasonality, gestation time, and large mammal extinctions". in Martin, P.S.. Quaternary extinctions: A prehistoric revolution. Tucson, AZ: Univ. Arizona Press. pp. 354–404. ISBN 978-0-8165-1100-6.
- ↑ Louys, Julien; Curnoe, D.; Tong, H. (2007). "Characteristics of Pleistocene megafauna extinctions in Southeast Asia". Palaeogeography, Palaeoclimatology, Palaeoecology 243 (1–2): 152–173. doi:10.1016/j.palaeo.2006.07.011. Bibcode: 2007PPP...243..152L. http://doc.rero.ch/record/16043/files/PAL_E3832.pdf.
- ↑ Wroe, Stephen; Field, Judith H.; Archer, Michael; Grayson, Donald K.; Price, Gilbert J.; Louys, Julien; Faith, J. Tyler; Webb, Gregory E. et al. (28 May 2013). "Climate change frames debate over the extinction of megafauna in Sahul (Pleistocene Australia-New Guinea)" (in en). Proceedings of the National Academy of Sciences of the United States of America 110 (22): 8777–8781. doi:10.1073/pnas.1302698110. ISSN 0027-8424. PMID 23650401. PMC 3670326. https://pnas.org/doi/full/10.1073/pnas.1302698110. Retrieved 2 January 2024.
- ↑ Mann, Daniel H.; Groves, Pamela; Reanier, Richard E.; Gaglioti, Benjamin V.; Kunz, Michael L.; Shapiro, Beth (17 November 2015). "Life and extinction of megafauna in the ice-age Arctic" (in en). Proceedings of the National Academy of Sciences of the United States of America 112 (46): 14301–14306. doi:10.1073/pnas.1516573112. ISSN 0027-8424. PMID 26578776.
- ↑ MacDonald, G. M.; Beilman, D. W.; Kuzmin, Y. V.; Orlova, L. A.; Kremenetski, K. V.; Shapiro, B.; Wayne, R. K.; Van Valkenburgh, B. (12 June 2012). "Pattern of extinction of the woolly mammoth in Beringia" (in en). Nature Communications 3 (1): 893. doi:10.1038/ncomms1881. ISSN 2041-1723.
- ↑ Stewart, Mathew; Carleton, W. Christopher; Groucutt, Huw S. (16 February 2021). "Climate change, not human population growth, correlates with Late Quaternary megafauna declines in North America" (in en). Nature Communications 12 (1): 965. doi:10.1038/s41467-021-21201-8. ISSN 2041-1723.
- ↑ Broughton, Jack M.; Weitzel, Elic M. (21 December 2018). "Population reconstructions for humans and megafauna suggest mixed causes for North American Pleistocene extinctions" (in en). Nature Communications 9 (1). doi:10.1038/s41467-018-07897-1. ISSN 2041-1723. PMID 30575758. PMC 6303330. https://www.nature.com/articles/s41467-018-07897-1. Retrieved 2 January 2024.
- ↑ Meltzer, David J. (17 November 2020). "Overkill, glacial history, and the extinction of North America’s Ice Age megafauna" (in en). Proceedings of the National Academy of Sciences of the United States of America 117 (46): 28555–28563. doi:10.1073/pnas.2015032117. ISSN 0027-8424. PMID 33168739. PMC 7682371. https://pnas.org/doi/full/10.1073/pnas.2015032117. Retrieved 2 January 2024.
- ↑ 197.0 197.1 Scott, E. (2010). "Extinctions, scenarios, and assumptions: Changes in latest Pleistocene large herbivore abundance and distribution in western North America". Quaternary International 217 (1–2): 225–239. doi:10.1016/j.quaint.2009.11.003. Bibcode: 2010QuInt.217..225S.
- ↑ Andersen, S. T. (1973). "The differential pollen productivity of trees and its significance for the interpretation of a pollen diagram from a forested region". in Birks, H.J.B.. Oxford: Blackwell Scientific. ISBN 0-632-09120-7.
- ↑ Ashworth, C.A. (1980). "Environmental implications of a beetle assemblage from the Gervais formation (Early Wisconsinian?), Minnesota". Quat. Res. 13 (2): 200–12. doi:10.1016/0033-5894(80)90029-0. Bibcode: 1980QuRes..13..200A.
- ↑ Bradley, R. S. (1985). Quaternary Paleoclimatology: Methods of Paleoclimatic Reconstruction. Winchester, MA: Allen & Unwin. ISBN 978-0-04-551068-9. https://archive.org/details/quaternarypaleoc0000unse.
- ↑ "Radiocarbon dating evidence for mammoths on Wrangel Island, Arctic Ocean, until 2000 BC". Radiocarbon 37 (1): 1–6. 1995. doi:10.1017/S0033822200014703. Bibcode: 1995Radcb..37....1V. https://journals.uair.arizona.edu/index.php/radiocarbon/article/viewFile/1640/1644.
- ↑ Flereov, C.C. (1967). "On the origin of the mammalian fauna of Canada". in Hopkins, D.M.. The Bering Land Bridge. Palo Alto: Stanford Univ. Press. pp. 271–80. ISBN 978-0-8047-0272-0. https://archive.org/details/beringlandbridge0000hopk.
- ↑ Frenzel, B. (1968). "The Pleistocene vegetation of northern Eurasia". Science 161 (3842): 637–49. doi:10.1126/science.161.3842.637. PMID 17801456. Bibcode: 1968Sci...161..637F.
- ↑ 204.0 204.1 McDonald, J. (1989). "The reordered North American selection regime and late Quaternary megafaunal extinctions". in Martin, P.S.. Quaternary extinctions: A prehistoric revolution. Tucson, AZ: Univ. Arizona Press. pp. 354–404. ISBN 978-0-8165-1100-6.
- ↑ Birks, H.J.B.; West, R.G. (1973). "A Symposium of the British Ecological Society". Oxford: Blackwell Scientific. ISBN 0-632-09120-7.
- ↑ McDonald, J. (1981). North American Bison: Their classification and evolution. Berkeley: Univ. Calif. Press. ISBN 978-0-520-04002-1.
- ↑ Burney, D. A. (1993). "Recent animal extinctions: recipes for disaster". American Scientist 81 (6): 530–41. Bibcode: 1993AmSci..81..530B.
- ↑ Vartanyan, S.L.; Garutt, V.E.; Sher, A.V. (1993). "Holocene dwarf mammoths from Wangel Island in the Siberian Arctic". Nature 362 (6418): 337–40. doi:10.1038/362337a0. PMID 29633990. Bibcode: 1993Natur.362..337V.
- ↑ Pennycuick, C.J. (1979). "Energy costs of locomotion and the concept of "Foraging radius"". Serengetti: Dynamics of an Ecosystem. Chicago: Univ. Chicago Press. pp. 164–85. ISBN 978-0-226-76029-2.
- ↑ Wing, L.D.; Buss, I.O. (1970). "Elephants and Forests". Wildl. Mong. (19).
- ↑ Owen-Smith, R.N. (1992). Megaherbivores: The influence of very large body size on ecology. Cambridge studies in ecology. Cambridge: Cambridge Univ. Press. ISBN 978-0-521-42637-4.
- ↑ Presenter: Willis, Paul; Producer: Bryce, Clay; Producer: Searle, Mike (17 August 2006). "Thylacoleo "The Beast of the Nullarbor"". Catalyst. Australian Broadcasting Commission. Archived from the original on 2020-09-30.
- ↑ "An arid-adapted middle Pleistocene vertebrate fauna from south-central Australia". Nature 445 (7126): 422–425. January 2007. doi:10.1038/nature05471. PMID 17251978. Bibcode: 2007Natur.445..422P.
- ↑ "New ages for the last Australian megafauna: continent-wide extinction about 46,000 years ago". Science 292 (5523): 1888–92. June 2001. doi:10.1126/science.1060264. PMID 11397939. Bibcode: 2001Sci...292.1888R.
- ↑ Fisher, Daniel C. (2009). "Paleobiology and Extinction of Proboscideans in the Great Lakes Region of North America". in Haynes, Gary. American Megafaunal Extinctions at the End of the Pleistocene. Vertebrate Paleobiology and Paleoanthropology. Springer. pp. 55–75. doi:10.1007/978-1-4020-8793-6_4. ISBN 978-1-4020-8792-9.
- ↑ Whitney-Smith, Elin (2008). "The Evolution of an Ecosystem: Pleistocene Extinctions". Unifying themes in complex systems IV proceedings of the Fourth International Conference on Complex Systems. Springer. ISBN 978-3-540-73849-7. https://www.researchgate.net/publication/237242493.
- ↑ Whitney-Smith, Elin (2012). "Creating the tiniest bison: A system dynamics model of ecological evolution". Ruminants: Anatomy, Behavior, and Diseases. Nova Biomedical. ISBN 9781620810644. https://www.researchgate.net/publication/286116200.
- ↑ 218.0 218.1 MacFee, Ross D. E.; Marx, Preston A. (1997). "Humans, hyperdisease and first-contact extinctions". in Goodman, S.; Patterson, B. D.. Natural Change and Human Impact in Madagascar. Washington DC: Smithsonian Press. pp. 169–217. ISBN 978-1-56098-683-6.
- ↑ MacFee, Ross D. E.; Marx, Preston A. (1998). "Lightning Strikes Twice: Blitzkrieg, Hyperdisease, and Global Explanations of the Late Quaternary Catastrophic Extinctions". American Museum of Natural History. http://www.amnh.biz/science/biodiversity/extinction/Day1/bytes/MacPheePres.html.
- ↑ MacFee, Ross D. E.; Marx, Preston A. (1997). "The 40,000-year Plague: Humans, Hyperdisease, and First-contact Extinctions". Washington DC: Smithsonian Institution Press. pp. 169–217.
- ↑ Lapointe, D. A.; Atkinson, C. T.; Samuel, M. D. (2012). "Ecology and conservation biology of avian malaria". Annals of the New York Academy of Sciences 1249 (1): 211–26. doi:10.1111/j.1749-6632.2011.06431.x. PMID 22320256. Bibcode: 2012NYASA1249..211L. https://zenodo.org/record/1230770.
- ↑ Fiedel, S. (2005). "Man's best friend: mammoth's worst enemy?". World Archaeology 37: 11–35. doi:10.1080/0043824042000329540. https://zenodo.org/record/895416.
- ↑ Nyström, Sofie; Hammarström, Per (7 January 2014). "Is the prevalent human prion protein 129M/V mutation a living fossil from a Paleolithic panzootic superprion pandemic?". Prion 8 (1): 2–10. doi:10.4161/pri.27601. PMID 24398570.
- ↑ Lyons, K.; Smith, F. A.; Wagner, P. J.; White, E. P.; Brown, J. H. (2004). "Was a 'hyperdisease' responsible for the late Pleistocene megafaunal extinction?". Ecology Letters 7 (9): 859–68. doi:10.1111/j.1461-0248.2004.00643.x. Bibcode: 2004EcolL...7..859K. http://biology.unm.edu/fasmith/Web_Page_PDFs/Lyons_et_al_2004_WN.pdf.
- ↑ Bunch, Ted E.; Hermes, Robert E.; Moore, Andrew M.T.; Kennett, Douglas J.; Weaver, James C.; Wittke, James H.; DeCarli, Paul S.; Bischoff, James L. et al. (10 July 2012). "Very high-temperature impact melt products as evidence for cosmic airbursts and impacts 12,900 years ago" (in en). Proceedings of the National Academy of Sciences of the United States of America 109 (28). doi:10.1073/pnas.1204453109. ISSN 0027-8424. PMID 22711809. PMC 3396500. https://pnas.org/doi/full/10.1073/pnas.1204453109. Retrieved 2 January 2024.
- ↑ Firestone, R. B.; West, A.; Kennett, J. P.; Becker, L.; Bunch, T. E.; Revay, Z. S.; Schultz, P. H.; Belgya, T. et al. (9 October 2007). "Evidence for an extraterrestrial impact 12,900 years ago that contributed to the megafaunal extinctions and the Younger Dryas cooling" (in en). Proceedings of the National Academy of Sciences of the United States of America 104 (41): 16016–16021. doi:10.1073/pnas.0706977104. ISSN 0027-8424. PMID 17901202. PMC 1994902. https://pnas.org/doi/full/10.1073/pnas.0706977104. Retrieved 2 January 2024.
- ↑ Pinter, Nicholas; Scott, Andrew C.; Daulton, Tyrone L.; Podoll, Andrew; Koeberl, Christian; Anderson, R. Scott; Ishman, Scott E. (1 June 2011). "The Younger Dryas impact hypothesis: A requiem". Earth-Science Reviews 106 (3): 247–264. doi:10.1016/j.earscirev.2011.02.005. ISSN 0012-8252. https://www.sciencedirect.com/science/article/pii/S0012825211000262. Retrieved 2 January 2024.
- ↑ Boslough, Mark (March 2023). "Apocalypse!". Skeptic Magazine 28 (1): 51–59. https://www.skeptic.com/reading_room/graham-hancocks-ancient-apocalypse-hypothesis-put-to-test/.
- ↑ Channell, J. E. T.; Vigliotti, L. (29 May 2019). "The Role of Geomagnetic Field Intensity in Late Quaternary Evolution of Humans and Large Mammals" (in en). Reviews of Geophysics 57 (3): 709–738. doi:10.1029/2018RG000629. ISSN 8755-1209. https://agupubs.onlinelibrary.wiley.com/doi/10.1029/2018RG000629. Retrieved 2 January 2024.
- ↑ Kershaw, G.P. (1984). "Tundra plant communities of the Mackenzie mountains, Northwest Territories; floristic characteristics of long term surface disturbances". in Olson, R.. Northern Ecology and Resource Management: Memorial Essays honoring Don Gill. Edmonton, Canada: Univ. Alberta Press. pp. 239–311. ISBN 978-0-88864-047-5. https://archive.org/details/northernecologyr0000unse/page/239.
- ↑ Webber, P.J.; Miller, P.C.; Chapin, F.S. III; MacCown, B.H. (1980). "The vegetation: pattern and succession". in Brown, J.. An Arctic ecosystem: the coastal tundra at Barrow, Alaska. US/IBP Synthesis. Stroudsburg, PA: Dowden Hutchinson & Ross. pp. 186–219. 12.
- ↑ Wolf, A.; Doughty, C. E.; Malhi, Y. (2013). "Lateral Diffusion of Nutrients by Mammalian Herbivores in Terrestrial Ecosystems". PLoS ONE 8 (8): e71352. doi:10.1371/journal.pone.0071352. PMID 23951141. Bibcode: 2013PLoSO...871352W.
- ↑ Marshall, M. (2013-08-11). "Ecosystems still feel the pain of ancient extinctions". New Scientist. https://www.newscientist.com/article/dn24026-ecosystems-still-feel-the-pain-of-ancient-extinctions.html. Retrieved 12 August 2013.
- ↑ 234.0 234.1 Doughty, C. E.; Wolf, A.; Malhi, Y. (2013). "The legacy of the Pleistocene megafauna extinctions on nutrient availability in Amazonia". Nature Geoscience 6 (9): 761–764. doi:10.1038/ngeo1895. Bibcode: 2013NatGe...6..761D. https://zenodo.org/record/3425870.[yes|permanent dead link|dead link}}]
- ↑ 235.0 235.1 Doughty, C. E.; Wolf, A.; Field, C. B. (2010). "Biophysical feedbacks between the Pleistocene megafauna extinction and climate: The first human‐induced global warming?". Geophysical Research Letters 37 (15): n/a. doi:10.1029/2010GL043985. Bibcode: 2010GeoRL..3715703D.
- ↑ Vignieri, S. (25 July 2014). "Vanishing fauna (Special issue)". Science 345 (6195): 392–412. doi:10.1126/science.345.6195.392. PMID 25061199. Bibcode: 2014Sci...345..392V.
- ↑ Sandom, Christopher; Faurby, Søren; Sandel, Brody; Svenning, Jens-Christian (4 June 2014). "Global late Quaternary megafauna extinctions linked to humans, not climate change". Proceedings of the Royal Society B 281 (1787): 20133254. doi:10.1098/rspb.2013.3254. PMID 24898370.
- ↑ 238.0 238.1 238.2 Wilkinson, D. M.; Nisbet, E. G.; Ruxton, G. D. (2012). "Could methane produced by sauropod dinosaurs have helped drive Mesozoic climate warmth?". Current Biology 22 (9): R292–R293. doi:10.1016/j.cub.2012.03.042. PMID 22575462.
- ↑ "Dinosaur gases 'warmed the Earth'". 7 May 2012. http://www.bbc.co.uk/nature/17953792.
- ↑ Kelliher, F. M.; Clark, H. (15 March 2010). "Methane emissions from bison—An historic herd estimate for the North American Great Plains". Agricultural and Forest Meteorology 150 (3): 473–577. doi:10.1016/j.agrformet.2009.11.019. Bibcode: 2010AgFM..150..473K.
- ↑ Smith, F. A.; Elliot, S. M.; Lyons, S. K. (23 May 2010). "Methane emissions from extinct megafauna". Nature Geoscience 3 (6): 374–375. doi:10.1038/ngeo877. Bibcode: 2010NatGe...3..374S.
- ↑ Tóth, Anikó B.; Lyons, S. Kathleen; Barr, W. Andrew; Behrensmeyer, Anna K.; Blois, Jessica L.; Bobe, René; Davis, Matt; Du, Andrew et al. (20 September 2019). "Reorganization of surviving mammal communities after the end-Pleistocene megafaunal extinction" (in en). Science 365 (6459): 1305–1308. doi:10.1126/science.aaw1605. ISSN 0036-8075. https://www.science.org/doi/10.1126/science.aaw1605. Retrieved 2 January 2024.
- ↑ Smith, Felisa A.; Elliott Smith, Emma A.; Villaseñor, Amelia; Tomé, Catalina P.; Lyons, S. Kathleen; Newsome, Seth D. (27 September 2022). "Late Pleistocene megafauna extinction leads to missing pieces of ecological space in a North American mammal community" (in en). Proceedings of the National Academy of Sciences of the United States of America 119 (39). doi:10.1073/pnas.2115015119. ISSN 0027-8424. PMID 36122233. PMC 9522422. https://pnas.org/doi/full/10.1073/pnas.2115015119. Retrieved 2 January 2024.
- ↑ Galetti, Mauro; Moleón, Marcos; Jordano, Pedro; Pires, Mathias M.; Guimarães, Paulo R.; Pape, Thomas; Nichols, Elizabeth; Hansen, Dennis et al. (9 October 2017). "Ecological and evolutionary legacy of megafauna extinctions" (in en). Biological Reviews 93 (2): 845–862. doi:10.1111/brv.12374. ISSN 1464-7931. https://onlinelibrary.wiley.com/doi/10.1111/brv.12374. Retrieved 2 January 2024.
- ↑ Tan, Hui Zhen; Jansen, Justin JFJ; Allport, Gary A; Garg, Kritika M; Chattopadhyay, Balaji; Irestedt, Martin; Pang, Sean EH; Chilton, Glen et al. (7 August 2023). "Megafaunal extinctions, not climate change, may explain Holocene genetic diversity declines in Numenius shorebirds" (in en). eLife 12. doi:10.7554/eLife.85422. ISSN 2050-084X. PMID 37549057. PMC 10406428. https://elifesciences.org/articles/85422. Retrieved 2 January 2024.
- ↑ Wehi, Priscilla M.; Cox, Murray P.; Roa, Tom; Whaanga, Hēmi (4 June 2018). "Human Perceptions of Megafaunal Extinction Events Revealed by Linguistic Analysis of Indigenous Oral Traditions" (in en). Human Ecology 46 (4): 461–470. doi:10.1007/s10745-018-0004-0. ISSN 0300-7839. PMID 30237652. PMC 6133014. http://link.springer.com/10.1007/s10745-018-0004-0. Retrieved 2 January 2024.
External links
Hyperdisease hypothesis
- MacFee, Ross D. E.; Marx, Preston A. (1998). "Lightning Strikes Twice: Blitzkrieg, Hyperdisease, and Global Explanations of the Late Quaternary Catastrophic Extinctions". American Museum of Natural History. http://www.amnh.biz/science/biodiversity/extinction/Day1/bytes/MacPheePres.html.
- J.H. Brown. "Was a hyperdisease responsible?". http://biology.unm.edu/JHBrown/Published/WasAHyperdiseaseResponsible.x.pdf.
Second-order predation
- Elin Whitney-Smith. "Quaternary.Net". http://quaternary.net/.
Other links
- "Ice Age Bay Area". http://www.kqed.org/quest/television/ice-age-bay-area2.
- "The Extinct Late Pleistocene Mammals of North America". https://www.pbs.org/wgbh/nova/stoneage/mega-list.html.
- Peter Tyson. "End of the Big Beasts". https://www.pbs.org/wgbh/nova/beta/evolution/end-big-beasts.html.
- S. Kathleen Lyons; Felisa A. Smith; James H. Brown (2004). "Of mice, mastodons and men: human-mediated extinctions on four continents". Evolutionary Ecology Research 6: 339–358. http://biology.unm.edu/fasmith/Web_Page_PDFs/Lyons_et_al_2004_EER.pdf.
- "Return to the Ice Age: The La Brea Exploration Guide". http://www.tarpits.org/education/guide/index.html.
![]() | Original source: https://en.wikipedia.org/wiki/Late Pleistocene extinctions.
Read more |