Astronomy:Asteroid
An asteroid is a minor planet—an object that is neither a true planet nor a comet—that orbits within the inner Solar System. They are rocky, metallic, or icy bodies with no atmosphere. The size and shape of asteroids vary significantly, ranging from small rubble piles under a kilometer across to Ceres, a dwarf planet almost 1000 km in diameter.
Of the roughly one million known asteroids,[1] the greatest number are located between the orbits of Mars and Jupiter, approximately 2 to 4 AU from the Sun, in a region known as the main asteroid belt. Asteroids are generally classified to be of three types: C-type, M-type, and S-type. These were named after and are generally identified with carbonaceous, metallic, and silicaceous compositions, respectively. The size of asteroids varies greatly; the largest, Ceres, is almost 1,000 km (600 mi) across and qualifies as a dwarf planet. The total mass of all the asteroids combined is only 3% that of Earth's Moon. The majority of main belt asteroids follow slightly elliptical, stable orbits, revolving in the same direction as the Earth and taking from three to six years to complete a full circuit of the Sun.[2]
Asteroids have been historically observed from Earth; the Galileo spacecraft provided the first close observation of an asteroid. Several dedicated missions to asteroids were subsequently launched by NASA and JAXA, with plans for other missions in progress. NASA's NEAR Shoemaker studied Eros, and Dawn observed Vesta and Ceres. JAXA's missions Hayabusa and Hayabusa2 studied and returned samples of Itokawa and Ryugu, respectively. OSIRIS-REx studied Bennu, collecting a sample in 2020 which was delivered back to Earth in 2023. NASA's Lucy, launched in 2021, will study ten different asteroids, two from the main belt and eight Jupiter trojans. Psyche, launched in October 2023, will study a metallic asteroid of the same name.
Near-Earth asteroids can threaten all life on the planet; an asteroid impact event resulted in the Cretaceous–Paleogene extinction. Different asteroid deflection strategies have been proposed; the Double Asteroid Redirection Test spacecraft, or DART, was launched in 2021 and intentionally impacted Dimorphos in September 2022, successfully altering its orbit by crashing into it.
History of observations
Despite their large numbers, asteroids are a relatively recent discovery, with the first known asteroid—Ceres—only being identified in 1801.[3] Only one asteroid, 4 Vesta, which has a relatively reflective surface, is normally visible to the naked eye. When favorably positioned, 4 Vesta can be seen in dark skies. Rarely, small asteroids passing close to Earth may be visible to the naked eye for a short amount of time.[4] (As of April 2022), the Minor Planet Center had data on 1,199,224 minor planets in the inner and outer Solar System, of which about 614,690 had enough information to be given numbered designations.[5]
Discovery of Ceres
In 1772, German astronomer Johann Elert Bode, citing Johann Daniel Titius, published a numerical procession known as the Titius–Bode law (now discredited). Except for an unexplained gap between Mars and Jupiter, Bode's formula seemed to predict the orbits of the known planets.[6][7] He wrote the following explanation for the existence of a "missing planet":
This latter point seems in particular to follow from the astonishing relation which the known six planets observe in their distances from the Sun. Let the distance from the Sun to Saturn be taken as 100, then Mercury is separated by 4 such parts from the Sun. Venus is 4 + 3 = 7. The Earth 4 + 6 = 10. Mars 4 + 12 = 16. Now comes a gap in this so orderly progression. After Mars there follows a space of 4 + 24 = 28 parts, in which no planet has yet been seen. Can one believe that the Founder of the universe had left this space empty? Certainly not. From here we come to the distance of Jupiter by 4 + 48 = 52 parts, and finally to that of Saturn by 4 + 96 = 100 parts.[8]
Bode's formula predicted another planet would be found with an orbital radius near 2.8 astronomical units (AU), or 420 million km, from the Sun.[7] The Titius–Bode law got a boost with William Herschel's discovery of Uranus near the predicted distance for a planet beyond Saturn.[6] In 1800, a group headed by Franz Xaver von Zach, editor of the German astronomical journal Monatliche Correspondenz (Monthly Correspondence), sent requests to 24 experienced astronomers (whom he dubbed the "celestial police"),[7] asking that they combine their efforts and begin a methodical search for the expected planet.[7] Although they did not discover Ceres, they later found the asteroids 2 Pallas, 3 Juno and 4 Vesta.[7]
One of the astronomers selected for the search was Giuseppe Piazzi, a Catholic priest at the Academy of Palermo, Sicily. Before receiving his invitation to join the group, Piazzi discovered Ceres on 1 January 1801.[9] He was searching for "the 87th [star] of the Catalogue of the Zodiacal stars of Mr la Caille",[6] but found that "it was preceded by another".[6] Instead of a star, Piazzi had found a moving star-like object, which he first thought was a comet:[10]
The light was a little faint, and of the colour of Jupiter, but similar to many others which generally are reckoned of the eighth magnitude. Therefore I had no doubt of its being any other than a fixed star. [...] The evening of the third, my suspicion was converted into certainty, being assured it was not a fixed star. Nevertheless before I made it known, I waited till the evening of the fourth, when I had the satisfaction to see it had moved at the same rate as on the preceding days.[6]
Piazzi observed Ceres a total of 24 times, the final time on 11 February 1801, when illness interrupted his work. He announced his discovery on 24 January 1801 in letters to only two fellow astronomers, his compatriot Barnaba Oriani of Milan and Bode in Berlin.[3] He reported it as a comet but "since its movement is so slow and rather uniform, it has occurred to me several times that it might be something better than a comet".[6] In April, Piazzi sent his complete observations to Oriani, Bode, and French astronomer Jérôme Lalande. The information was published in the September 1801 issue of the Monatliche Correspondenz.[10]
By this time, the apparent position of Ceres had changed (mostly due to Earth's motion around the Sun), and was too close to the Sun's glare for other astronomers to confirm Piazzi's observations. Toward the end of the year, Ceres should have been visible again, but after such a long time it was difficult to predict its exact position. To recover Ceres, mathematician Carl Friedrich Gauss, then 24 years old, developed an efficient method of orbit determination.[10] In a few weeks, he predicted the path of Ceres and sent his results to von Zach. On 31 December 1801, von Zach and fellow celestial policeman Heinrich W. M. Olbers found Ceres near the predicted position and thus recovered it.[10] At 2.8 AU from the Sun, Ceres appeared to fit the Titius–Bode law almost perfectly; however, Neptune, once discovered in 1846, was 8 AU closer than predicted, leading most astronomers to conclude that the law was a coincidence.[11] Piazzi named the newly discovered object Ceres Ferdinandea, "in honor of the patron goddess of Sicily and of King Ferdinand of Bourbon".[8]
Further search
Three other asteroids (2 Pallas, 3 Juno, and 4 Vesta) were discovered by von Zach's group over the next few years, with Vesta found in 1807.[7] No new asteroids were discovered until 1845. Amateur astronomer Karl Ludwig Hencke started his searches of new asteroids in 1830, and fifteen years later, while looking for Vesta, he found the asteroid later named 5 Astraea. It was the first new asteroid discovery in 38 years. Carl Friedrich Gauss was given the honor of naming the asteroid. After this, other astronomers joined; 15 asteroids were found by the end of 1851. In 1868, when James Craig Watson discovered the 100th asteroid, the French Academy of Sciences engraved the faces of Karl Theodor Robert Luther, John Russell Hind, and Hermann Goldschmidt, the three most successful asteroid-hunters at that time, on a commemorative medallion marking the event.[12]
In 1891, Max Wolf pioneered the use of astrophotography to detect asteroids, which appeared as short streaks on long-exposure photographic plates.[12] This dramatically increased the rate of detection compared with earlier visual methods: Wolf alone discovered 248 asteroids, beginning with 323 Brucia,[13] whereas only slightly more than 300 had been discovered up to that point. It was known that there were many more, but most astronomers did not bother with them, some calling them "vermin of the skies",[14] a phrase variously attributed to Eduard Suess[15] and Edmund Weiss.[16] Even a century later, only a few thousand asteroids were identified, numbered and named.
19th and 20th centuries
In the past, asteroids were discovered by a four-step process. First, a region of the sky was photographed by a wide-field telescope or astrograph. Pairs of photographs were taken, typically one hour apart. Multiple pairs could be taken over a series of days. Second, the two films or plates of the same region were viewed under a stereoscope. A body in orbit around the Sun would move slightly between the pair of films. Under the stereoscope, the image of the body would seem to float slightly above the background of stars. Third, once a moving body was identified, its location would be measured precisely using a digitizing microscope. The location would be measured relative to known star locations.[17]
These first three steps do not constitute asteroid discovery: the observer has only found an apparition, which gets a provisional designation, made up of the year of discovery, a letter representing the half-month of discovery, and finally a letter and a number indicating the discovery's sequential number (example: 1998 FJ74). The last step is sending the locations and time of observations to the Minor Planet Center, where computer programs determine whether an apparition ties together earlier apparitions into a single orbit. If so, the object receives a catalogue number and the observer of the first apparition with a calculated orbit is declared the discoverer, and granted the honor of naming the object subject to the approval of the International Astronomical Union.[18]
Naming
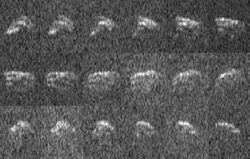
By 1851, the Royal Astronomical Society decided that asteroids were being discovered at such a rapid rate that a different system was needed to categorize or name asteroids. In 1852, when de Gasparis discovered the twentieth asteroid, Benjamin Valz gave it a name and a number designating its rank among asteroid discoveries, 20 Massalia. Sometimes asteroids were discovered and not seen again. So, starting in 1892, new asteroids were listed by the year and a capital letter indicating the order in which the asteroid's orbit was calculated and registered within that specific year. For example, the first two asteroids discovered in 1892 were labeled 1892A and 1892B. However, there were not enough letters in the alphabet for all of the asteroids discovered in 1893, so 1893Z was followed by 1893AA. A number of variations of these methods were tried, including designations that included year plus a Greek letter in 1914. A simple chronological numbering system was established in 1925.[12][19]
Currently all newly discovered asteroids receive a provisional designation (such as 2002 AT4) consisting of the year of discovery and an alphanumeric code indicating the half-month of discovery and the sequence within that half-month. Once an asteroid's orbit has been confirmed, it is given a number, and later may also be given a name (e.g. 433 Eros). The formal naming convention uses parentheses around the number—e.g. (433) Eros—but dropping the parentheses is quite common. Informally, it is also common to drop the number altogether, or to drop it after the first mention when a name is repeated in running text.[20] In addition, names can be proposed by the asteroid's discoverer, within guidelines established by the International Astronomical Union.[21]
Symbols
The first asteroids to be discovered were assigned iconic symbols like the ones traditionally used to designate the planets. By 1855 there were two dozen asteroid symbols, which often occurred in multiple variants.[22]
In 1851, after the fifteenth asteroid, Eunomia, had been discovered, Johann Franz Encke made a major change in the upcoming 1854 edition of the Berliner Astronomisches Jahrbuch (BAJ, Berlin Astronomical Yearbook). He introduced a disk (circle), a traditional symbol for a star, as the generic symbol for an asteroid. The circle was then numbered in order of discovery to indicate a specific asteroid. The numbered-circle convention was quickly adopted by astronomers, and the next asteroid to be discovered (16 Psyche, in 1852) was the first to be designated in that way at the time of its discovery. However, Psyche was given an iconic symbol as well, as were a few other asteroids discovered over the next few years. 20 Massalia was the first asteroid that was not assigned an iconic symbol, and no iconic symbols were created after the 1855 discovery of 37 Fides.[lower-alpha 1][23]
Terminology
The first discovered asteroid, Ceres, was originally considered a new planet.[lower-alpha 2] It was followed by the discovery of other similar bodies, which with the equipment of the time appeared to be points of light like stars, showing little or no planetary disc, though readily distinguishable from stars due to their apparent motions. This prompted the astronomer Sir William Herschel to propose the term asteroid,[lower-alpha 3] coined in Greek as ἀστεροειδής, or asteroeidēs, meaning 'star-like, star-shaped', and derived from the Ancient Greek ἀστήρ astēr 'star, planet'. In the early second half of the 19th century, the terms asteroid and planet (not always qualified as "minor") were still used interchangeably.[lower-alpha 4]
Traditionally, small bodies orbiting the Sun were classified as comets, asteroids, or meteoroids, with anything smaller than one meter across being called a meteoroid. The term asteroid never had a formal definition,[28] with the broader term small Solar System bodies being preferred by the International Astronomical Union (IAU).[29] As no IAU definition exists, asteroid can be defined as "an irregularly shaped rocky body orbiting the Sun that does not qualify as a planet or a dwarf planet under the IAU definitions of those terms".[30]
When found, asteroids were seen as a class of objects distinct from comets, and there was no unified term for the two until small Solar System body was coined in 2006. The main difference between an asteroid and a comet is that a comet shows a coma due to sublimation of near-surface ices by solar radiation. A few objects have ended up being dual-listed because they were first classified as minor planets but later showed evidence of cometary activity. Conversely, some (perhaps all) comets are eventually depleted of their surface volatile ices and become asteroid-like. A further distinction is that comets typically have more eccentric orbits than most asteroids; "asteroids" with notably eccentric orbits are probably dormant or extinct comets.[31]
For almost two centuries, from the discovery of Ceres in 1801 until the discovery of the first centaur, 2060 Chiron in 1977, all known asteroids spent most of their time at or within the orbit of Jupiter, though a few such as 944 Hidalgo ventured far beyond Jupiter for part of their orbit. When astronomers started finding more small bodies that permanently resided further out than Jupiter, now called centaurs, they numbered them among the traditional asteroids. There was debate over whether these objects should be considered asteroids or given a new classification. Then, when the first trans-Neptunian object (other than Pluto), 15760 Albion, was discovered in 1992, and especially when large numbers of similar objects started turning up, new terms were invented to sidestep the issue: Kuiper-belt object, trans-Neptunian object, scattered-disc object, and so on. They inhabit the cold outer reaches of the Solar System where ices remain solid and comet-like bodies are not expected to exhibit much cometary activity; if centaurs or trans-Neptunian objects were to venture close to the Sun, their volatile ices would sublimate, and traditional approaches would classify them as comets and not asteroids.
The innermost of these are the Kuiper-belt objects, called "objects" partly to avoid the need to classify them as asteroids or comets.[32] They are thought to be predominantly comet-like in composition, though some may be more akin to asteroids.[33] Furthermore, most do not have the highly eccentric orbits associated with comets, and the ones so far discovered are larger than traditional comet nuclei. (The much more distant Oort cloud is hypothesized to be the main reservoir of dormant comets.) Other recent observations, such as the analysis of the cometary dust collected by the Stardust probe, are increasingly blurring the distinction between comets and asteroids,[34] suggesting "a continuum between asteroids and comets" rather than a sharp dividing line.[35]
The minor planets beyond Jupiter's orbit are sometimes also called "asteroids", especially in popular presentations.[lower-alpha 5] However, it is becoming increasingly common for the term asteroid to be restricted to minor planets of the inner Solar System.[32] Therefore, this article will restrict itself for the most part to the classical asteroids: objects of the asteroid belt, Jupiter trojans, and near-Earth objects.
When the IAU introduced the class small Solar System bodies in 2006 to include most objects previously classified as minor planets and comets, they created the class of dwarf planets for the largest minor planets—those that have enough mass to have become ellipsoidal under their own gravity. According to the IAU, "the term 'minor planet' may still be used, but generally, the term 'Small Solar System Body' will be preferred."[37] Currently only the largest object in the asteroid belt, Ceres, at about 975 km (606 mi) across, has been placed in the dwarf planet category.[38][39]
Formation
Many asteroids are the shattered remnants of planetesimals, bodies within the young Sun's solar nebula that never grew large enough to become planets.[40] It is thought that planetesimals in the asteroid belt evolved much like the rest of objects in the solar nebula until Jupiter neared its current mass, at which point excitation from orbital resonances with Jupiter ejected over 99% of planetesimals in the belt. Simulations and a discontinuity in spin rate and spectral properties suggest that asteroids larger than approximately 120 km (75 mi) in diameter accreted during that early era, whereas smaller bodies are fragments from collisions between asteroids during or after the Jovian disruption.[41] Ceres and Vesta grew large enough to melt and differentiate, with heavy metallic elements sinking to the core, leaving rocky minerals in the crust.[42]
In the Nice model, many Kuiper-belt objects are captured in the outer asteroid belt, at distances greater than 2.6 AU. Most were later ejected by Jupiter, but those that remained may be the D-type asteroids, and possibly include Ceres.[43]
Distribution within the Solar System
Various dynamical groups of asteroids have been discovered orbiting in the inner Solar System. Their orbits are perturbed by the gravity of other bodies in the Solar System and by the Yarkovsky effect. Significant populations include:
Asteroid belt
The majority of known asteroids orbit within the asteroid belt between the orbits of Mars and Jupiter, generally in relatively low-eccentricity (i.e. not very elongated) orbits. This belt is estimated to contain between 1.1 and 1.9 million asteroids larger than 1 km (0.6 mi) in diameter,[44] and millions of smaller ones. These asteroids may be remnants of the protoplanetary disk, and in this region the accretion of planetesimals into planets during the formative period of the Solar System was prevented by large gravitational perturbations by Jupiter.
Contrary to popular imagery, the asteroid belt is mostly empty. The asteroids are spread over such a large volume that reaching an asteroid without aiming carefully would be improbable. Nonetheless, hundreds of thousands of asteroids are currently known, and the total number ranges in the millions or more, depending on the lower size cutoff. Over 200 asteroids are known to be larger than 100 km,[45] and a survey in the infrared wavelengths has shown that the asteroid belt has between 700,000 and 1.7 million asteroids with a diameter of 1 km or more.[46] The absolute magnitudes of most of the known asteroids are between 11 and 19, with the median at about 16.[47]
The total mass of the asteroid belt is estimated to be 2.39×1021 kg, which is just 3% of the mass of the Moon; the mass of the Kuiper Belt and Scattered Disk is over 100 times as large.[48] The four largest objects, Ceres, Vesta, Pallas, and Hygiea, account for maybe 62% of the belt's total mass, with 39% accounted for by Ceres alone.
Trojans
Trojans are populations that share an orbit with a larger planet or moon, but do not collide with it because they orbit in one of the two Lagrangian points of stability, L4 and L5, which lie 60° ahead of and behind the larger body.
In the Solar System, most known trojans share the orbit of Jupiter. They are divided into the Greek camp at L4 (ahead of Jupiter) and the Trojan camp at L5 (trailing Jupiter). More than a million Jupiter trojans larger than one kilometer are thought to exist,[49] of which more than 7,000 are currently catalogued. In other planetary orbits only nine Mars trojans, 28 Neptune trojans, two Uranus trojans, and two Earth trojans, have been found to date. A temporary Venus trojan is also known. Numerical orbital dynamics stability simulations indicate that Saturn and Uranus probably do not have any primordial trojans.[50]
Near-Earth asteroids
Near-Earth asteroids, or NEAs, are asteroids that have orbits that pass close to that of Earth. Asteroids that actually cross Earth's orbital path are known as Earth-crossers. (As of April 2022), a total of 28,772 near-Earth asteroids were known; 878 have a diameter of one kilometer or larger.[51]
A small number of NEAs are extinct comets that have lost their volatile surface materials, although having a faint or intermittent comet-like tail does not necessarily result in a classification as a near-Earth comet, making the boundaries somewhat fuzzy. The rest of the near-Earth asteroids are driven out of the asteroid belt by gravitational interactions with Jupiter.[52][53]
Many asteroids have natural satellites (minor-planet moons). (As of October 2021), there were 85 NEAs known to have at least one moon, including three known to have two moons.[54] The asteroid 3122 Florence, one of the largest potentially hazardous asteroids with a diameter of 4.5 km (2.8 mi), has two moons measuring 100–300 m (330–980 ft) across, which were discovered by radar imaging during the asteroid's 2017 approach to Earth.[55]
Near-Earth asteroids are divided into groups based on their semi-major axis (a), perihelion distance (q), and aphelion distance (Q):[56][52]
- The Atiras or Apoheles have orbits strictly inside Earth's orbit: an Atira asteroid's aphelion distance (Q) is smaller than Earth's perihelion distance (0.983 AU). That is, Q < 0.983 AU, which implies that the asteroid's semi-major axis is also less than 0.983 AU.[57]
- The Atens have a semi-major axis of less than 1 AU and cross Earth's orbit. Mathematically, a < 1.0 AU and Q > 0.983 AU. (0.983 AU is Earth's perihelion distance.)
- The Apollos have a semi-major axis of more than 1 AU and cross Earth's orbit. Mathematically, a > 1.0 AU and q < 1.017 AU. (1.017 AU is Earth's aphelion distance.)
- The Amors have orbits strictly outside Earth's orbit: an Amor asteroid's perihelion distance (q) is greater than Earth's aphelion distance (1.017 AU). Amor asteroids are also near-earth objects so q < 1.3 AU. In summary, 1.017 AU < q < 1.3 AU. (This implies that the asteroid's semi-major axis (a) is also larger than 1.017 AU.) Some Amor asteroid orbits cross the orbit of Mars.
Martian moons
Lua error in Module:Multiple_image at line 163: attempt to perform arithmetic on local 'totalwidth' (a nil value).
It is unclear whether Martian moons Phobos and Deimos are captured asteroids or were formed due to impact event on Mars.[58] Phobos and Deimos both have much in common with carbonaceous C-type asteroids, with spectra, albedo, and density very similar to those of C- or D-type asteroids.[59] Based on their similarity, one hypothesis is that both moons may be captured main-belt asteroids.[60][61] Both moons have very circular orbits which lie almost exactly in Mars's equatorial plane, and hence a capture origin requires a mechanism for circularizing the initially highly eccentric orbit, and adjusting its inclination into the equatorial plane, most probably by a combination of atmospheric drag and tidal forces,[62] although it is not clear whether sufficient time was available for this to occur for Deimos.[58] Capture also requires dissipation of energy. The current Martian atmosphere is too thin to capture a Phobos-sized object by atmospheric braking.[58] Geoffrey A. Landis has pointed out that the capture could have occurred if the original body was a binary asteroid that separated under tidal forces.[61][63]
Phobos could be a second-generation Solar System object that coalesced in orbit after Mars formed, rather than forming concurrently out of the same birth cloud as Mars.[64]
Another hypothesis is that Mars was once surrounded by many Phobos- and Deimos-sized bodies, perhaps ejected into orbit around it by a collision with a large planetesimal.[65] The high porosity of the interior of Phobos (based on the density of 1.88 g/cm3, voids are estimated to comprise 25 to 35 percent of Phobos's volume) is inconsistent with an asteroidal origin.[66] Observations of Phobos in the thermal infrared suggest a composition containing mainly phyllosilicates, which are well known from the surface of Mars. The spectra are distinct from those of all classes of chondrite meteorites, again pointing away from an asteroidal origin.[67] Both sets of findings support an origin of Phobos from material ejected by an impact on Mars that reaccreted in Martian orbit,[68] similar to the prevailing theory for the origin of Earth's moon.
Characteristics
Size distribution
Asteroids vary greatly in size, from almost 1000 km for the largest down to rocks just 1 meter across, below which an object is classified as a meteoroid.[lower-alpha 6] The three largest are very much like miniature planets: they are roughly spherical, have at least partly differentiated interiors,[69] and are thought to be surviving protoplanets. The vast majority, however, are much smaller and are irregularly shaped; they are thought to be either battered planetesimals or fragments of larger bodies.
The dwarf planet Ceres is by far the largest asteroid, with a diameter of 940 km (580 mi). The next largest are 4 Vesta and 2 Pallas, both with diameters of just over 500 km (300 mi). Vesta is the brightest of the four main-belt asteroids that can, on occasion, be visible to the naked eye.[70] On some rare occasions, a near-Earth asteroid may briefly become visible without technical aid; see 99942 Apophis.
The mass of all the objects of the asteroid belt, lying between the orbits of Mars and Jupiter, is estimated to be (2394±6)×1018 kg, ≈ 3.25% of the mass of the Moon. Of this, Ceres comprises 938×1018 kg, about 40% of the total. Adding in the next three most massive objects, Vesta (11%), Pallas (8.5%), and Hygiea (3–4%), brings this figure up to a bit over 60%, whereas the next seven most-massive asteroids bring the total up to 70%.[48] The number of asteroids increases rapidly as their individual masses decrease.
The number of asteroids decreases markedly with increasing size. Although the size distribution generally follows a power law, there are 'bumps' at about 5 km and 100 km, where more asteroids than expected from such a curve are found. Most asteroids larger than approximately 120 km in diameter are primordial (surviving from the accretion epoch), whereas most smaller asteroids are products of fragmentation of primordial asteroids. The primordial population of the main belt was probably 200 times what it is today.[71][72]
Largest asteroids
Three largest objects in the asteroid belt, Ceres, Vesta, and Pallas, are intact protoplanets that share many characteristics common to planets, and are atypical compared to the majority of irregularly shaped asteroids. The fourth-largest asteroid, Hygiea, appears nearly spherical although it may have an undifferentiated interior,[73] like the majority of asteroids. The four largest asteroids constitute half the mass of the asteroid belt.
Ceres is the only asteroid that appears to have a plastic shape under its own gravity and hence the only one that is a dwarf planet.[74] It has a much higher absolute magnitude than the other asteroids, of around 3.32,[75] and may possess a surface layer of ice.[76] Like the planets, Ceres is differentiated: it has a crust, a mantle and a core.[76] No meteorites from Ceres have been found on Earth.[77]
Vesta, too, has a differentiated interior, though it formed inside the Solar System's frost line, and so is devoid of water;[78][79] its composition is mainly of basaltic rock with minerals such as olivine.[80] Aside from the large crater at its southern pole, Rheasilvia, Vesta also has an ellipsoidal shape. Vesta is the parent body of the Vestian family and other V-type asteroids, and is the source of the HED meteorites, which constitute 5% of all meteorites on Earth.
Pallas is unusual in that, like Uranus, it rotates on its side, with its axis of rotation tilted at high angles to its orbital plane.[81] Its composition is similar to that of Ceres: high in carbon and silicon, and perhaps partially differentiated.[82] Pallas is the parent body of the Palladian family of asteroids.
Hygiea is the largest carbonaceous asteroid[83] and, unlike the other largest asteroids, lies relatively close to the plane of the ecliptic. It is the largest member and presumed parent body of the Hygiean family of asteroids. Because there is no sufficiently large crater on the surface to be the source of that family, as there is on Vesta, it is thought that Hygiea may have been completely disrupted in the collision that formed the Hygiean family and recoalesced after losing a bit less than 2% of its mass. Observations taken with the Very Large Telescope's SPHERE imager in 2017 and 2018, revealed that Hygiea has a nearly spherical shape, which is consistent both with it being in hydrostatic equilibrium, or formerly being in hydrostatic equilibrium, or with being disrupted and recoalescing.[84][85]
Internal differentiation of large asteroids is possibly related to their lack of natural satellites, as satellites of main belt asteroids are mostly believed to form from collisional disruption, creating a rubble pile structure.[77]
Name | Orbital radius (AU) |
Orbital period (years) |
Inclination to ecliptic |
Orbital eccentricity |
Diameter (km) |
Diameter (% of Moon) |
Mass (×1018 kg) |
Mass (% of Ceres) |
Density (g/cm3) |
Rotation period (hr) |
---|---|---|---|---|---|---|---|---|---|---|
Ceres | 2.77 | 4.60 | 10.6° | 0.079 | 964×964×892 (mean 939.4) |
27% | 938 | 100% | 2.16±0.01 | 9.07 |
Vesta | 2.36 | 3.63 | 7.1° | 0.089 | 573×557×446 (mean 525.4) |
15% | 259 | 28% | 3.46 ± 0.04 | 5.34 |
Pallas | 2.77 | 4.62 | 34.8° | 0.231 | 550×516×476 (mean 511±4) |
15% | 204±3 | 21% | 2.92±0.08 | 7.81 |
Hygiea | 3.14 | 5.56 | 3.8° | 0.117 | 450×430×424 (mean 433±8) |
12% | 87±7 | 9% | 2.06±0.20 | 13.8 |
Rotation
Measurements of the rotation rates of large asteroids in the asteroid belt show that there is an upper limit. Very few asteroids with a diameter larger than 100 meters have a rotation period less than 2.2 hours.[86] For asteroids rotating faster than approximately this rate, the inertial force at the surface is greater than the gravitational force, so any loose surface material would be flung out. However, a solid object should be able to rotate much more rapidly. This suggests that most asteroids with a diameter over 100 meters are rubble piles formed through the accumulation of debris after collisions between asteroids.[87]
Color
Asteroids become darker and redder with age due to space weathering.[88] However evidence suggests most of the color change occurs rapidly, in the first hundred thousand years, limiting the usefulness of spectral measurement for determining the age of asteroids.[89]
Surface features
Except for the "big four" (Ceres, Pallas, Vesta, and Hygiea), asteroids are likely to be broadly similar in appearance, if irregular in shape. 50 km (31 mi) 253 Mathilde is a rubble pile saturated with craters with diameters the size of the asteroid's radius. Earth-based observations of 300 km (190 mi) 511 Davida, one of the largest asteroids after the big four, reveal a similarly angular profile, suggesting it is also saturated with radius-size craters.[90] Medium-sized asteroids such as Mathilde and 243 Ida, that have been observed up close, also reveal a deep regolith covering the surface. Of the big four, Pallas and Hygiea are practically unknown. Vesta has compression fractures encircling a radius-size crater at its south pole but is otherwise a spheroid.
Dawn spacecraft revealed that Ceres has a heavily cratered surface, but with fewer large craters than expected.[91] Models based on the formation of the current asteroid belt had suggested Ceres should possess 10 to 15 craters larger than 400 km (250 mi) in diameter.[91] The largest confirmed crater on Ceres, Kerwan Basin, is 284 km (176 mi) across.[92] The most likely reason for this is viscous relaxation of the crust slowly flattening out larger impacts.[91]
Composition
Asteroids are classified by their characteristic emission spectra, with the majority falling into three main groups: C-type, M-type, and S-type. These were named after and are generally identified with carbonaceous (carbon-rich), metallic, and silicaceous (stony) compositions, respectively. The physical composition of asteroids is varied and in most cases poorly understood. Ceres appears to be composed of a rocky core covered by an icy mantle, where Vesta is thought to have a nickel-iron core, olivine mantle, and basaltic crust.[93] Thought to be the largest undifferentiated asteroid, 10 Hygiea seems to have a uniformly primitive composition of carbonaceous chondrite, but it may actually be a differentiated asteroid that was globally disrupted by an impact and then reassembled. Other asteroids appear to be the remnant cores or mantles of proto-planets, high in rock and metal. Most small asteroids are believed to be piles of rubble held together loosely by gravity, although the largest are probably solid. Some asteroids have moons or are co-orbiting binaries: rubble piles, moons, binaries, and scattered asteroid families are thought to be the results of collisions that disrupted a parent asteroid, or possibly a planet.[94]
In the main asteroid belt, there appear to be two primary populations of asteroid: a dark, volatile-rich population, consisting of the C-type and P-type asteroids, with albedos less than 0.10 and densities under 2.2 g/cm3, and a dense, volatile-poor population, consisting of the S-type and M-type asteroids, with albedos over 0.15 and densities greater than 2.7. Within these populations, larger asteroids are denser, presumably due to compression. There appears to be minimal macro-porosity (interstitial vacuum) in the score of asteroids with masses greater than 10×1018 kg.[95]
Composition is calculated from three primary sources: albedo, surface spectrum, and density. The last can only be determined accurately by observing the orbits of moons the asteroid might have. So far, every asteroid with moons has turned out to be a rubble pile, a loose conglomeration of rock and metal that may be half empty space by volume. The investigated asteroids are as large as 280 km in diameter, and include 121 Hermione (268×186×183 km), and 87 Sylvia (384×262×232 km). Few asteroids are larger than 87 Sylvia, none of them have moons. The fact that such large asteroids as Sylvia may be rubble piles, presumably due to disruptive impacts, has important consequences for the formation of the Solar System: computer simulations of collisions involving solid bodies show them destroying each other as often as merging, but colliding rubble piles are more likely to merge. This means that the cores of the planets could have formed relatively quickly.[96]
Water
Scientists hypothesize that some of the first water brought to Earth was delivered by asteroid impacts after the collision that produced the Moon.[97] In 2009, the presence of water ice was confirmed on the surface of 24 Themis using NASA's Infrared Telescope Facility. The surface of the asteroid appears completely covered in ice. As this ice layer is sublimating, it may be getting replenished by a reservoir of ice under the surface. Organic compounds were also detected on the surface.[98][99][97][100] The presence of ice on 24 Themis makes the initial theory plausible.[97]
In October 2013, water was detected on an extrasolar body for the first time, on an asteroid orbiting the white dwarf GD 61.[101] On 22 January 2014, European Space Agency (ESA) scientists reported the detection, for the first definitive time, of water vapor on Ceres, the largest object in the asteroid belt.[102] The detection was made by using the far-infrared abilities of the Herschel Space Observatory.[103] The finding is unexpected because comets, not asteroids, are typically considered to "sprout jets and plumes". According to one of the scientists, "The lines are becoming more and more blurred between comets and asteroids."[103]
Findings have shown that solar winds can react with the oxygen in the upper layer of the asteroids and create water. It has been estimated that "every cubic metre of irradiated rock could contain up to 20 litres"; study was conducted using an atom probe tomography, numbers are given for the Itokawa S-type asteroid.[104][105]
Acfer 049, a meteorite discovered in Algeria in 1990, was shown in 2019 to have an ultraporous lithology (UPL): porous texture that could be formed by removal of ice that filled these pores, this suggests that UPL "represent fossils of primordial ice".[106]
Organic compounds
Asteroids contain traces of amino acids and other organic compounds, and some speculate that asteroid impacts may have seeded the early Earth with the chemicals necessary to initiate life, or may have even brought life itself to Earth (an event called "panspermia").[107][108] In August 2011, a report, based on NASA studies with meteorites found on Earth, was published suggesting DNA and RNA components (adenine, guanine and related organic molecules) may have been formed on asteroids and comets in outer space.[109][110][111]
In November 2019, scientists reported detecting, for the first time, sugar molecules, including ribose, in meteorites, suggesting that chemical processes on asteroids can produce some fundamentally essential bio-ingredients important to life, and supporting the notion of an RNA world prior to a DNA-based origin of life on Earth, and possibly, as well, the notion of panspermia.[112][113][114]
Classification
Asteroids are commonly categorized according to two criteria: the characteristics of their orbits, and features of their reflectance spectrum.
Orbital classification
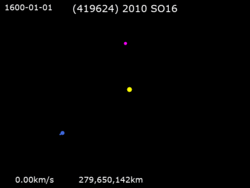
Many asteroids have been placed in groups and families based on their orbital characteristics. Apart from the broadest divisions, it is customary to name a group of asteroids after the first member of that group to be discovered. Groups are relatively loose dynamical associations, whereas families are tighter and result from the catastrophic break-up of a large parent asteroid sometime in the past.[115] Families are more common and easier to identify within the main asteroid belt, but several small families have been reported among the Jupiter trojans.[116] Main belt families were first recognized by Kiyotsugu Hirayama in 1918 and are often called Hirayama families in his honor.
About 30–35% of the bodies in the asteroid belt belong to dynamical families, each thought to have a common origin in a past collision between asteroids. A family has also been associated with the plutoid dwarf planet Haumea.
Some asteroids have unusual horseshoe orbits that are co-orbital with Earth or another planet. Examples are 3753 Cruithne and 2002 AA29. The first instance of this type of orbital arrangement was discovered between Saturn's moons Epimetheus and Janus. Sometimes these horseshoe objects temporarily become quasi-satellites for a few decades or a few hundred years, before returning to their earlier status. Both Earth and Venus are known to have quasi-satellites.
Such objects, if associated with Earth or Venus or even hypothetically Mercury, are a special class of Aten asteroids. However, such objects could be associated with the outer planets as well.
Spectral classification
In 1975, an asteroid taxonomic system based on color, albedo, and spectral shape was developed by Chapman, Morrison, and Zellner.[117] These properties are thought to correspond to the composition of the asteroid's surface material. The original classification system had three categories: C-types for dark carbonaceous objects (75% of known asteroids), S-types for stony (silicaceous) objects (17% of known asteroids) and U for those that did not fit into either C or S. This classification has since been expanded to include many other asteroid types. The number of types continues to grow as more asteroids are studied.
The two most widely used taxonomies now used are the Tholen classification and SMASS classification. The former was proposed in 1984 by David J. Tholen, and was based on data collected from an eight-color asteroid survey performed in the 1980s. This resulted in 14 asteroid categories.[118] In 2002, the Small Main-Belt Asteroid Spectroscopic Survey resulted in a modified version of the Tholen taxonomy with 24 different types. Both systems have three broad categories of C, S, and X asteroids, where X consists of mostly metallic asteroids, such as the M-type. There are also several smaller classes.[119]
The proportion of known asteroids falling into the various spectral types does not necessarily reflect the proportion of all asteroids that are of that type; some types are easier to detect than others, biasing the totals.
Problems
Originally, spectral designations were based on inferences of an asteroid's composition.[120] However, the correspondence between spectral class and composition is not always very good, and a variety of classifications are in use. This has led to significant confusion. Although asteroids of different spectral classifications are likely to be composed of different materials, there are no assurances that asteroids within the same taxonomic class are composed of the same (or similar) materials.
Active asteroids
Active asteroids are objects that have asteroid-like orbits but show comet-like visual characteristics. That is, they show comae, tails, or other visual evidence of mass-loss (like a comet), but their orbit remains within Jupiter's orbit (like an asteroid).[121][122] These bodies were originally designated main-belt comets (MBCs) in 2006 by astronomers David Jewitt and Henry Hsieh, but this name implies they are necessarily icy in composition like a comet and that they only exist within the main-belt, whereas the growing population of active asteroids shows that this is not always the case.[121][123][124]
The first active asteroid discovered is 7968 Elst–Pizarro. It was discovered (as an asteroid) in 1979 but then was found to have a tail by Eric Elst and Guido Pizarro in 1996 and given the cometary designation 133P/Elst-Pizarro.[121][125] Another notable object is 311P/PanSTARRS: observations made by the Hubble Space Telescope revealed that it had six comet-like tails.[126] The tails are suspected to be streams of material ejected by the asteroid as a result of a rubble pile asteroid spinning fast enough to remove material from it.[127]
By smashing into the asteroid Dimorphos, NASA's Double Asteroid Redirection Test spacecraft made it an active asteroid. Scientists had proposed that some active asteroids are the result of impact events, but no one had ever observed the activation of an asteroid. The DART mission activated Dimorphos under precisely known and carefully observed impact conditions, enabling the detailed study of the formation of an active asteroid for the first time.[128][129] Observations show that Dimorphos lost approximately 1 million kilograms after the collision.[130] Impact produced a dust plume that temporarily brightened the Didymos system and developed a 10,000-kilometer (6,200 mi)-long dust tail that persisted for several months.[131][132][133]
Observation and exploration
Until the age of space travel, objects in the asteroid belt could only be observed with large telescopes, their shapes and terrain remaining a mystery. The best modern ground-based telescopes and the Earth-orbiting Hubble Space Telescope can only resolve a small amount of detail on the surfaces of the largest asteroids. Limited information about the shapes and compositions of asteroids can be inferred from their light curves (variation in brightness during rotation) and their spectral properties. Sizes can be estimated by timing the lengths of star occultations (when an asteroid passes directly in front of a star). Radar imaging can yield good information about asteroid shapes and orbital and rotational parameters, especially for near-Earth asteroids. Spacecraft flybys can provide much more data than any ground or space-based observations; sample-return missions gives insights about regolith composition.
Ground-based observations
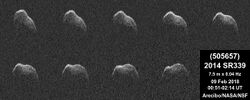
As asteroids are rather small and faint objects, the data that can be obtained from ground-based observations (GBO) are limited. By means of ground-based optical telescopes the visual magnitude can be obtained; when converted into the absolute magnitude it gives a rough estimate of the asteroid's size. Light-curve measurements can also be made by GBO; when collected over a long period of time it allows an estimate of the rotational period, the pole orientation (sometimes), and a rough estimate of the asteroid's shape. Spectral data (both visible-light and near-infrared spectroscopy) gives information about the object's composition, used to classify the observed asteroids. Such observations are limited as they provide information about only the thin layer on the surface (up to several micrometers).[134] As planetologist Patrick Michel writes:
Mid- to thermal-infrared observations, along with polarimetry measurements, are probably the only data that give some indication of actual physical properties. Measuring the heat flux of an asteroid at a single wavelength gives an estimate of the dimensions of the object; these measurements have lower uncertainty than measurements of the reflected sunlight in the visible-light spectral region. If the two measurements can be combined, both the effective diameter and the geometric albedo—the latter being a measure of the brightness at zero phase angle, that is, when illumination comes from directly behind the observer—can be derived. In addition, thermal measurements at two or more wavelengths, plus the brightness in the visible-light region, give information on the thermal properties. The thermal inertia, which is a measure of how fast a material heats up or cools off, of most observed asteroids is lower than the bare-rock reference value but greater than that of the lunar regolith; this observation indicates the presence of an insulating layer of granular material on their surface. Moreover, there seems to be a trend, perhaps related to the gravitational environment, that smaller objects (with lower gravity) have a small regolith layer consisting of coarse grains, while larger objects have a thicker regolith layer consisting of fine grains. However, the detailed properties of this regolith layer are poorly known from remote observations. Moreover, the relation between thermal inertia and surface roughness is not straightforward, so one needs to interpret the thermal inertia with caution.[134][excessive quote]
Near-Earth asteroids that come into close vicinity of the planet can be studied in more details with radar; it provides information about the surface of the asteroid (for example can show the presence of craters and boulders). Such observations were conducted by the Arecibo Observatory in Puerto Rico (305 meter dish) and Goldstone Observatory in California (70 meter dish). Radar observations can also be used for accurate determination of the orbital and rotational dynamics of observed objects.[134]
Space-based observations
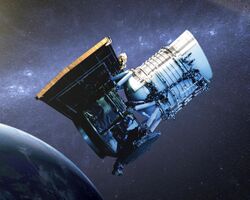

Both space and ground-based observatories conducted asteroid search programs; the space-based searches are expected to detect more objects because there is no atmosphere to interfere and because they can observe larger portions of the sky. NEOWISE observed more than 100,000 asteroids of the main belt, Spitzer Space Telescope observed more than 700 near-Earth asteroids. These observations determined rough sizes of the majority of observed objects, but provided limited detail about surface properties (such as regolith depth and composition, angle of repose, cohesion, and porosity).[134]
Asteroids were also studied by the Hubble Space Telescope, such as tracking the colliding asteroids in the main belt,[135][136] break-up of an asteroid,[137] observing an active asteroid with six comet-like tails,[138] and observing asteroids that were chosen as targets of dedicated missions.[139][140]
Space probe missions
According to Patrick Michel
The internal structure of asteroids is inferred only from indirect evidence: bulk densities measured by spacecraft, the orbits of natural satellites in the case of asteroid binaries, and the drift of an asteroid's orbit due to the Yarkovsky thermal effect. A spacecraft near an asteroid is perturbed enough by the asteroid's gravity to allow an estimate of the asteroid's mass. The volume is then estimated using a model of the asteroid's shape. Mass and volume allow the derivation of the bulk density, whose uncertainty is usually dominated by the errors made on the volume estimate. The internal porosity of asteroids can be inferred by comparing their bulk density with that of their assumed meteorite analogues, dark asteroids seem to be more porous (>40%) than bright ones. The nature of this porosity is unclear.[134]
Dedicated missions
The first asteroid to be photographed in close-up was 951 Gaspra in 1991, followed in 1993 by 243 Ida and its moon Dactyl, all of which were imaged by the Galileo probe en route to Jupiter. Other asteroids briefly visited by spacecraft en route to other destinations include 9969 Braille (by Deep Space 1 in 1999), 5535 Annefrank (by Stardust in 2002), 2867 Šteins and 21 Lutetia (by the Rosetta probe in 2008), and 4179 Toutatis (China's lunar orbiter Chang'e 2, which flew within 3.2 km (2 mi) in 2012).
The first dedicated asteroid probe was NASA's NEAR Shoemaker, which photographed 253 Mathilde in 1997, before entering into orbit around 433 Eros, finally landing on its surface in 2001. It was the first spacecraft to successfully orbit and land on an asteroid.[141] From September to November 2005, the Japanese Hayabusa probe studied 25143 Itokawa in detail and returned samples of its surface to Earth on 13 June 2010, the first asteroid sample-return mission. In 2007, NASA launched the Dawn spacecraft, which orbited 4 Vesta for a year, and observed the dwarf planet Ceres for three years.
Hayabusa2, a probe launched by JAXA 2014, orbited its target asteroid 162173 Ryugu for more than a year and took samples that were delivered to Earth in 2020. The spacecraft is now on an extended mission and expected to arrive at a new target in 2031.
NASA launched the OSIRIS-REx in 2016, a sample return mission to asteroid 101955 Bennu. In 2021, the probe departed the asteroid with a sample from its surface. Sample was delivered to Earth in September 2023. The spacecraft continues its extended mission, designated OSIRIS-APEX, to explore near-Earth asteroid Apophis in 2029.
In 2021, NASA launched Double Asteroid Redirection Test (DART), a mission to test technology for defending Earth against potential hazardous objects. DART deliberately crashed into the minor-planet moon Dimorphos of the double asteroid Didymos in September 2022 to assess the potential of a spacecraft impact to deflect an asteroid from a collision course with Earth.[142] In October, NASA declared DART a success, confirming it had shortened Dimorphos' orbital period around Didymos by about 32 minutes.[143]
- Asteroid-dedicated space probes
Planned missions
- NASA's Lucy, launched in 2021, would visit eight asteroids, one from the main belt and seven Jupiter trojans; it is the first mission to trojans. The main mission would start in 2027.[144][145]
- NASA's Psyche, launched in October 2023, will study the large metallic asteroid of the same name, and will arrive there in 2029.
- ESA's Hera, planned for launch in 2024, will study the results of the DART impact. It will measure the size and morphology of the crater, and momentum transmitted by the impact, to determine the efficiency of the deflection produced by DART.
- JAXA's DESTINY+ is a mission for a flyby of the Geminids meteor shower parent body 3200 Phaethon, as well as various minor bodies. Its launch is planned for 2024.[146]
- CNSA's Tianwen-2 is planned to launch in 2025.[147] It will use solar electric propulsion to explore the co-orbital near-Earth asteroid 469219 Kamoʻoalewa and the active asteroid 311P/PanSTARRS. The spacecraft will collect samples of the regolith of Kamo'oalewa.[148]
Asteroid mining
The concept of asteroid mining was proposed in 1970s. Matt Anderson defines successful asteroid mining as "the development of a mining program that is both financially self-sustaining and profitable to its investors".[149] It has been suggested that asteroids might be used as a source of materials that may be rare or exhausted on Earth,[150] or materials for constructing space habitats. Materials that are heavy and expensive to launch from Earth may someday be mined from asteroids and used for space manufacturing and construction.[151][152]
As resource depletion on Earth becomes more real, the idea of extracting valuable elements from asteroids and returning these to Earth for profit, or using space-based resources to build solar-power satellites and space habitats,[153][154] becomes more attractive. Hypothetically, water processed from ice could refuel orbiting propellant depots.[155][156]
From the astrobiological perspective, asteroid prospecting could provide scientific data for the search for extraterrestrial intelligence (SETI). Some astrophysicists have suggested that if advanced extraterrestrial civilizations employed asteroid mining long ago, the hallmarks of these activities might be detectable.[157][158][159]
Threats to Earth
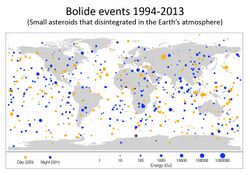
There is increasing interest in identifying asteroids whose orbits cross Earth's, and that could, given enough time, collide with Earth. The three most important groups of near-Earth asteroids are the Apollos, Amors, and Atens.
The near-Earth asteroid 433 Eros had been discovered as long ago as 1898, and the 1930s brought a flurry of similar objects. In order of discovery, these were: 1221 Amor, 1862 Apollo, 2101 Adonis, and finally 69230 Hermes, which approached within 0.005 AU of Earth in 1937. Astronomers began to realize the possibilities of Earth impact.
Two events in later decades increased the alarm: the increasing acceptance of the Alvarez hypothesis that an impact event resulted in the Cretaceous–Paleogene extinction, and the 1994 observation of Comet Shoemaker-Levy 9 crashing into Jupiter. The U.S. military also declassified the information that its military satellites, built to detect nuclear explosions, had detected hundreds of upper-atmosphere impacts by objects ranging from one to ten meters across.
All of these considerations helped spur the launch of highly efficient surveys, consisting of charge-coupled device (CCD) cameras and computers directly connected to telescopes. (As of 2011), it was estimated that 89% to 96% of near-Earth asteroids one kilometer or larger in diameter had been discovered.[51] (As of October 2018), the LINEAR system alone had discovered 147,132 asteroids.[160] Among the surveys, 19,266 near-Earth asteroids have been discovered[161] including almost 900 more than 1 km (0.6 mi) in diameter.[162]
In June 2018, the National Science and Technology Council warned that the United States is unprepared for an asteroid impact event, and has developed and released the "National Near-Earth Object Preparedness Strategy Action Plan" to better prepare.[163][164][165] According to expert testimony in the United States Congress in 2013, NASA would require at least five years of preparation before a mission to intercept an asteroid could be launched.[166]
Asteroid deflection strategies
Various collision avoidance techniques have different trade-offs with respect to metrics such as overall performance, cost, failure risks, operations, and technology readiness.[167] There are various methods for changing the course of an asteroid/comet.[168] These can be differentiated by various types of attributes such as the type of mitigation (deflection or fragmentation), energy source (kinetic, electromagnetic, gravitational, solar/thermal, or nuclear), and approach strategy (interception,[169][170] rendezvous, or remote station).
Strategies fall into two basic sets: fragmentation and delay.[168][171] Fragmentation concentrates on rendering the impactor harmless by fragmenting it and scattering the fragments so that they miss the Earth or are small enough to burn up in the atmosphere. Delay exploits the fact that both the Earth and the impactor are in orbit. An impact occurs when both reach the same point in space at the same time, or more correctly when some point on Earth's surface intersects the impactor's orbit when the impactor arrives. Since the Earth is approximately 12,750 km in diameter and moves at approx. 30 km per second in its orbit, it travels a distance of one planetary diameter in about 425 seconds, or slightly over seven minutes. Delaying, or advancing the impactor's arrival by times of this magnitude can, depending on the exact geometry of the impact, cause it to miss the Earth.[172]
"Project Icarus" was one of the first projects designed in 1967 as a contingency plan in case of collision with 1566 Icarus. The plan relied on the new Saturn V rocket, which did not make its first flight until after the report had been completed. Six Saturn V rockets would be used, each launched at variable intervals from months to hours away from impact. Each rocket was to be fitted with a single 100-megaton nuclear warhead as well as a modified Apollo Service Module and uncrewed Apollo Command Module for guidance to the target. The warheads would be detonated 30 meters from the surface, deflecting or partially destroying the asteroid. Depending on the subsequent impacts on the course or the destruction of the asteroid, later missions would be modified or cancelled as needed. The "last-ditch" launch of the sixth rocket would be 18 hours prior to impact.[173]
Fiction
Asteroids and the asteroid belt are a staple of science fiction stories. Asteroids play several potential roles in science fiction: as places human beings might colonize, resources for extracting minerals, hazards encountered by spacecraft traveling between two other points, and as a threat to life on Earth or other inhabited planets, dwarf planets, and natural satellites by potential impact.
See also
- Exoasteroid
- List of asteroid close approaches to Earth
- List of exceptional asteroids
- Lost minor planet
- Meanings of minor-planet names
Notes
- ↑ Except for Pluto and, in the astrological community, for a few outer bodies such as 2060 Chiron.
- ↑ Ceres is the largest asteroid and now classified as a dwarf planet. All other asteroids are now classified as small Solar System bodies along with comets, centaurs, and the smaller trans-Neptunian objects.
- ↑ In an oral presentation,[24] Clifford Cunningham presented his finding that the word was coined by Charles Burney, Jr., the son of a friend of Herschel.[25][26]
- ↑ For example, the Annual of Scientific Discovery: "Professor J. Watson has been awarded by the Paris Academy of Sciences, the astronomical prize, Lalande foundation, for the discovery of eight new asteroids in one year. The planet Lydia (No. 110), discovered by M. Borelly at the Marseilles Observatory [...] M. Borelly had previously discovered two planets bearing the numbers 91 and 99 in the system of asteroids revolving between Mars and Jupiter".[27]
The Universal English Dictionary (John Craig, 1869) lists the asteroids (and gives their pronunciations) up to 64 Angelina, along with the definition "one of the recently-discovered planets." At this time it was common to anglicize the spellings of the names, e.g. "Aglaia" for 47 Aglaja and "Atalanta" for 36 Atalante. - ↑ For instance, a joint NASA–JPL public-outreach website states: We include Trojans (bodies captured in Jupiter's 4th and 5th Lagrange points), Centaurs (bodies in orbit between Jupiter and Neptune), and trans-Neptunian objects (orbiting beyond Neptune) in our definition of "asteroid" as used on this site, even though they may more correctly be called "minor planets" instead of asteroids.[36]
- ↑ The definition in the 1995 paper (Beech and Steel) has been updated by a 2010 paper (Rubin and Grossman) and the discovery of 1 meter asteroids.
References
- ↑
One or more of the preceding sentences incorporates text from a work now in the public domain: "Asteroids". NASA Solar System Exploration. https://solarsystem.nasa.gov/asteroids-comets-and-meteors/asteroids/overview/.
- ↑
One or more of the preceding sentences incorporates text from a work now in the public domain: "Asteroids (from the NEAR press kit)". https://nssdc.gsfc.nasa.gov/planetary/text/asteroids.txt.
- ↑ 3.0 3.1 Cunningham, Clifford J. (2001). The first asteroid: Ceres, 1801–2001. Star Lab Press. ISBN 978-0-9708162-1-4. https://books.google.com/books?id=CXdMPwAACAAJ. Retrieved 23 October 2015.
- ↑ Britt, Robert Roy (4 February 2005). "Closest flyby of large asteroid to be naked-eye visible". http://www.space.com/spacewatch/050204_2004_mn4.html.
- ↑ "Latest Published Data". International Astronomical Union. http://minorplanetcenter.net/mpc/summary.
- ↑ 6.0 6.1 6.2 6.3 6.4 6.5 Hoskin, Michael (26 June 1992). "Bode's Law and the Discovery of Ceres". Observatorio Astronomico di Palermo "Giuseppe S. Vaiana". http://www.astropa.unipa.it/HISTORY/hoskin.html.
- ↑ 7.0 7.1 7.2 7.3 7.4 7.5 Hogg, Helen Sawyer (1948). "The Titius-Bode Law and the Discovery of Ceres". Journal of the Royal Astronomical Society of Canada 242: 241–246. Bibcode: 1948JRASC..42..241S. http://articles.adsabs.harvard.edu//full/1948JRASC..42..241S/0000244.000.html. Retrieved 18 July 2021.
- ↑ 8.0 8.1 Foderà Serio, G.; Manara, A.; Sicoli, P. (2002). "Giuseppe Piazzi and the Discovery of Ceres". Asteroids III. Tucson: University of Arizona Press. pp. 17–24. ISBN 978-0-8165-4651-0. Bibcode: 2002aste.book...17F. https://www.lpi.usra.edu/books/AsteroidsIII/pdf/3027.pdf.
- ↑ Landau, Elizabeth (26 January 2016). "Ceres: Keeping Well-Guarded Secrets for 215 Years". NASA. http://www.jpl.nasa.gov/news/news.php?feature=4824.
- ↑ 10.0 10.1 10.2 10.3 Forbes, Eric G. (1971). "Gauss and the Discovery of Ceres". Journal for the History of Astronomy 2 (3): 195–199. doi:10.1177/002182867100200305. Bibcode: 1971JHA.....2..195F. http://adsabs.harvard.edu/full/1971JHA.....2..195F. Retrieved 18 July 2021.
- ↑ Michael Martin Nieto (1972). The Titius-Bode Law of Planetary Distances: Its History and Theory. Pergamon Press. ISBN 978-1-4831-5936-2. https://books.google.com/books?id=NneoBQAAQBAJ&q=bode+law+neptune+coincidence+1846&pg=PP1. Retrieved 23 September 2021.
- ↑ 12.0 12.1 12.2
One or more of the preceding sentences incorporates text from a work now in the public domain: "Dawn Community". JPL NASA. 21 May 2009. http://dawn.jpl.nasa.gov/DawnCommunity/flashbacks/fb_09.asp.
- ↑ "Dawn Classrooms – Biographies". JPL NASA. 18 June 2009. http://dawn.jpl.nasa.gov/DawnClassrooms/1_hist_dawn/bio.asp#wolf.
- ↑ Friedman, Lou. "Vermin of the Sky". http://www.planetary.org/blogs/guest-blogs/lou-friedman/20130219-vermin-of-the-sky.html.
- ↑ Hale, George E. (1916). "Some Reflections on the Progress of Astrophysics". Popular Astronomy. Address at the semi-centennial of the Dearborn Observatory 24: 550–558 [555]. Bibcode: 1916PA.....24..550H.
- ↑ Seares, Frederick H. (1930). "Address of the Retiring President of the Society in Awarding the Bruce Medal to Professor Max Wolf". Publications of the Astronomical Society of the Pacific 42 (245): 5–22 [10]. doi:10.1086/123986. Bibcode: 1930PASP...42....5S.
- ↑ Chapman, Mary G. (17 May 1992). "Carolyn Shoemaker, planetary astronomer and most successful 'comet hunter' to date". USGS. https://astrogeology.usgs.gov/About/People/CarolynShoemaker.
- ↑ "ESA Science & Technology – Asteroid numbers and names". https://sci.esa.int/web/home/-/30244-asteroid-numbers-and-names.
- ↑ "New- And Old-Style Minor Planet Designations". Harvard. 22 August 2009. http://www.cfa.harvard.edu/iau/info/OldDesDoc.html.
- ↑ "The Naming of Asteroids". The Open University. http://www.open.edu/openlearn/science-maths-technology/science/physics-and-astronomy/astronomy/the-naming-asteroids.
- ↑ "Asteroid naming guidelines". The Planetary Society. http://www.planetary.org/get-involved/contests/osirisrex/guidelines.html.
- ↑ "On the symbolic notation of the asteroids". Astronomical Journal 2: 80. 1852. doi:10.1086/100212. Bibcode: 1852AJ......2...80G.
- ↑ "When did the asteroids become minor planets?". Naval Meteorology and Oceanography Command. http://www.usno.navy.mil/USNO/astronomical-applications/astronomical-information-center/minor-planets.
- ↑ "HADII Abstracts". HAD Meeting with DPS. Denver, CO. October 2013. http://had.aas.org/meetings/2013bAbstracts.html#HADII. Retrieved 14 October 2013.
- ↑ Nolin, Robert (8 October 2013). "Local expert reveals who really coined the word 'asteroid'". Sun-Sentinel. http://www.sun-sentinel.com/news/broward/fl-asteroid-word-origin-20131008,0,501498,full.story.
- ↑ Wall, Mike (10 January 2011). "Who really invented the word 'Asteroid' for space rocks?". http://www.space.com/10593-post-william-herschel-coin-term-asteroid.html.
- ↑ Annual of Scientific Discovery. 1871. p. 316. https://books.google.com/books?id=NAMAAAAAMAAJ&pg=PA316.
- ↑ Bottke, William F.; Cellino, Alberto; Paolicchi, Paolo et al., eds (2002). Asteroids III. Tucson: University of Arizona Press. p. 670. ISBN 978-0-8165-4651-0. https://books.google.com/books?id=JwHTyO6IHh8C&pg=PA670. Retrieved 30 March 2022. "Since no formal definitions of comets and asteroids exist..."
- ↑ "Resolution B5 Definition of a Planet in the Solar System". The Minor Planet Center. https://www.iau.org/static/resolutions/Resolution_GA26-5-6.pdf. "All other objects (These currently include most of the Solar System asteroids, most Trans-Neptunian Objects (TNOs), comets, and other small bodies.), except satellites, orbiting the Sun shall be referred to collectively as "Small Solar System Bodies"."
- ↑ Harris, Alan W. (2011). "Asteroid". Encyclopedia of Astrobiology. pp. 102–112. doi:10.1007/978-3-642-11274-4_116. ISBN 978-3-642-11271-3.
- ↑ Weissman, Paul R.; Bottke, William F. Jr.; Levinson, Harold F. (2002). "Evolution of Comets into Asteroids". Southwest Research Institute. http://www.boulder.swri.edu/~hal/PDF/asteroids3.pdf.
- ↑ 32.0 32.1 "Are Kuiper Belt objects asteroids?". Cornell University. http://curious.astro.cornell.edu/question.php?number=601.
- ↑ Short, Nicholas M. Sr.. "Asteroids and Comets". NASA. http://rst.gsfc.nasa.gov/Sect19/Sect19_22.html.
- ↑ Comet dust seems more 'asteroidy'. Scientific American (audio podcast). 25 January 2008.
- ↑ "Comet samples are surprisingly asteroid-like". New Scientist. 24 January 2008. https://www.newscientist.com/channel/solar-system/comets-asteroids/dn13224-comet-samples-are-surprisingly-asteroidlike.html.
- ↑ "Asteroids". Jet Propulsion Laboratory. https://ssd.jpl.nasa.gov/?asteroids.
- ↑ "Pluto". International Astrophysical Union. http://www.iau.org/public/themes/pluto/.
- ↑ "Pluto and the Developing Landscape of Our Solar System". https://www.iau.org/public/themes/pluto/.
- ↑ "Exploration: Ceres". 26 June 2019. https://solarsystem.nasa.gov/planets/dwarf-planets/ceres/exploration.
- ↑ "What are asteroids and comets?". http://neo.jpl.nasa.gov/faq/#ast.
- ↑ Bottke, William F. Jr.; Durda, Daniel D.; Nesvorny, David; Jedicke, Robert; Morbidelli, Alessandro; Vokrouhlicky, David; Levison, Hal (2005). "The fossilized size distribution of the main asteroid belt". Icarus 175 (1): 111. doi:10.1016/j.icarus.2004.10.026. Bibcode: 2005Icar..175..111B. http://astro.mff.cuni.cz/davok/papers/fossil05.pdf.
- ↑ Kerrod, Robin (2000). Asteroids, Comets, and Meteors. Lerner Publications Co.. ISBN 978-0-585-31763-2. https://archive.org/details/asteroidscometsm00robi.
- ↑ McKinnon, William; McKinnon, B. (2008). "On The Possibility of Large KBOs Being Injected into The Outer Asteroid Belt". Bulletin of the American Astronomical Society 40: 464. Bibcode: 2008DPS....40.3803M.
- ↑ Tedesco, Edward; Metcalfe, Leo (4 April 2002). "New study reveals twice as many asteroids as previously believed" (Press release). European Space Agency. Archived from the original on 6 March 2023. Retrieved 21 February 2008.
- ↑ Yeomans, Donald K. (April 26, 2007). "JPL Small-Body Database Search Engine". NASA JPL. Search for asteroids in the main belt regions with a diameter >100. http://ssd.jpl.nasa.gov/sbdb_query.cgi.
- ↑ Tedesco, E. F.; Desert, F.-X. (2002). "The Infrared Space Observatory Deep Asteroid Search". The Astronomical Journal 123 (4): 2070–2082. doi:10.1086/339482. Bibcode: 2002AJ....123.2070T.
- ↑ Williams, Gareth (September 25, 2010). "Distribution of the Minor Planets". Minor Planet Center. http://www.minorplanetcenter.org/iau/lists/MPDistribution.html.
- ↑ 48.0 48.1 Pitjeva, E. V. (2018). "Masses of the Main Asteroid Belt and the Kuiper Belt from the Motions of Planets and Spacecraft". Solar System Research 44 (8–9): 554–566. doi:10.1134/S1063773718090050. Bibcode: 2018AstL...44..554P.
- ↑ Yoshida, F.; Nakamura, T. (Dec 2005). "Size Distribution of Faint Jovian L4 Trojan Asteroids". The Astronomical Journal 130 (6): 2900–2911. doi:10.1086/497571. Bibcode: 2005AJ....130.2900Y.
- ↑ Sheppard, Scott S.; Trujillo, Chadwick A. (June 2006). "A Thick Cloud of Neptune Trojans and their Colors". Science 313 (5786): 511–514. doi:10.1126/science.1127173. PMID 16778021. Bibcode: 2006Sci...313..511S. http://www.dtm.ciw.edu/users/sheppard/pub/Sheppard06NepTroj.pdf.
- ↑ 51.0 51.1 "Discovery Statistics". https://cneos.jpl.nasa.gov/stats/totals.html.
- ↑ 52.0 52.1 Morbidelli, Alessandro; Bottke, William F. Jr.; Froeschlé, Christiane; Michel, Patrick (January 2002). W. F. Bottke Jr.. ed. "Origin and Evolution of Near-Earth Objects". Asteroids III: 409–422. doi:10.2307/j.ctv1v7zdn4.33. Bibcode: 2002aste.book..409M. http://www.boulder.swri.edu/~bottke/Reprints/Morbidelli-etal_2002_AstIII_NEOs.pdf. Retrieved 2017-11-09.
- ↑ D.F. Lupishko; M. di Martino; T.A. Lupishko (September 2000). "What the physical properties of near-Earth asteroids tell us about sources of their origin?". Kinematika I Fizika Nebesnykh Tel Supplimen 3 (3): 213–216. Bibcode: 2000KFNTS...3..213L.
- ↑ "Asteroids with Satellites". Johnston's Archive. http://www.johnstonsarchive.net/astro/asteroidmoons.html.
- ↑ Lance Benner; Shantanu Naidu; Marina Brozovic; Paul Chodas (September 1, 2017). "Radar Reveals Two Moons Orbiting Asteroid Florence". News (NASA/JPL CNEOS). https://cneos.jpl.nasa.gov/news/news199.html.
- ↑ "NEO Basics. NEO Groups". NASA/JPL CNEOS. http://cneos.jpl.nasa.gov/neo/groups.html.
- ↑ de la Fuente Marcos, Carlos; de la Fuente Marcos, Raúl (1 August 2019). "Understanding the evolution of Atira-class asteroid 2019 AQ3, a major step towards the future discovery of the Vatira population". Monthly Notices of the Royal Astronomical Society 487 (2): 2742–2752. doi:10.1093/mnras/stz1437. Bibcode: 2019MNRAS.487.2742D.
- ↑ 58.0 58.1 58.2 Burns, Joseph A. (1992). "Contradictory Clues as to the Origin of the Martian Moons" in Mars, H. H. Kieffer et al., eds., Tucson: University of Arizona Press, Tucson [page needed]
- ↑ "Views of Phobos and Deimos". NASA. 27 November 2007. https://www.nasa.gov/mission_pages/MRO/multimedia/20071127-caption.html.
- ↑ "Close Inspection for Phobos". http://sci.esa.int/science-e/www/object/index.cfm?fobjectid=31031. "One idea is that Phobos and Deimos, Mars's other moon, are captured asteroids."
- ↑ 61.0 61.1 Landis, Geoffrey A.; "Origin of Martian Moons from Binary Asteroid Dissociation", American Association for the Advancement of Science Annual Meeting; Boston, MA, 2001, abstract
- ↑ Canup, Robin (2018-04-18). "Origin of Phobos and Deimos by the impact of a Vesta-to-Ceres sized body with Mars". Science Advances 4 (4): eaar6887. doi:10.1126/sciadv.aar6887. PMID 29675470. Bibcode: 2018SciA....4.6887C.
- ↑ Pätzold, Martin; Witasse, Olivier (4 March 2010). "Phobos Flyby Success". ESA. https://www.esa.int/Science_Exploration/Space_Science/Mars_Express/Phobos_flyby_success.
- ↑ Craddock, Robert A.; (1994); "The Origin of Phobos and Deimos", Abstracts of the 25th Annual Lunar and Planetary Science Conference, held in Houston, TX, 14–18 March 1994, p. 293
- ↑ Andert, Thomas P.; Rosenblatt, Pascal; Pätzold, Martin; Häusler, Bernd et al. (7 May 2010). "Precise mass determination and the nature of Phobos". Geophysical Research Letters 37 (9): L09202. doi:10.1029/2009GL041829. Bibcode: 2010GeoRL..37.9202A.
- ↑ Giuranna, Marco; Roush, Ted L.; Duxbury, Thomas; Hogan, Robert C.; Geminale, Anna; Formisano, Vittorio (2010). "Compositional Interpretation of PFS/MEx and TES/MGS Thermal Infrared Spectra of Phobos". http://meetingorganizer.copernicus.org/EPSC2010/EPSC2010-211.pdf. Retrieved 1 October 2010.
- ↑ "Mars Moon Phobos Likely Forged by Catastrophic Blast". Space.com. 27 September 2010. https://www.space.com/9201-mars-moon-phobos-forged-catastrophic-blast.html.
- ↑ Schmidt, B.; Russell, C.T.; Bauer, J.M.; Li, J.; McFadden, L.A.; Mutchler, M. et al. (2007). "Hubble Space Telescope Observations of 2 Pallas". Bulletin of the American Astronomical Society 39: 485. Bibcode: 2007DPS....39.3519S.
- ↑ Martinez, Patrick, ed (1994). The Observer's Guide to Astronomy. Practical Astronomy Handbooks. 1. Cambridge University Press. p. 297. ISBN 978-0-521-37945-8. https://books.google.com/books?id=k5iUVz7iFTQC&pg=PA297.
- ↑ Bottkejr, W; Durda, D; Nesvorny, D; Jedicke, R; Morbidelli, A; Vokrouhlicky, D; Levison, H (May 2005). "The fossilized size distribution of the main asteroid belt" (in en). Icarus 175 (1): 111–140. doi:10.1016/j.icarus.2004.10.026. Bibcode: 2005Icar..175..111B. https://linkinghub.elsevier.com/retrieve/pii/S0019103504003811.
- ↑ O'Brien, David P.; Sykes, Mark V. (December 2011). "The Origin and Evolution of the Asteroid Belt – Implications for Vesta and Ceres" (in en). Space Science Reviews 163 (1–4): 41–61. doi:10.1007/s11214-011-9808-6. ISSN 0038-6308. Bibcode: 2011SSRv..163...41O. http://link.springer.com/10.1007/s11214-011-9808-6.
- ↑ "Asteroids | Imaging the Universe". http://astro.physics.uiowa.edu/ITU/labs/general-astronomy/asteroids/.
- ↑ "The final IAU resolution on the definition of "planet" ready for voting" (Press release). International Astronomical Union. 24 August 2006. Retrieved 2 March 2007.
- ↑ Parker, J.W.; Stern, S.A.; Thomas, P.C.; Festou, M.C.; Merline, W.J.; Young, E.F.; Binzel, R.P.; Lebofsky, L.A. (2002). "Analysis of the First Disk-resolved Images of Ceres from Ultraviolet Observations with the Hubble Space Telescope". The Astronomical Journal 123 (1): 549–557. doi:10.1086/338093. Bibcode: 2002AJ....123..549P.
- ↑ 76.0 76.1 "Asteroid 1 Ceres". The Planetary Society. http://www.planetary.org/explore/topics/asteroids_and_comets/ceres.html.
- ↑ 77.0 77.1 "Dawn mission's search for satellites of Ceres: Intact protoplanets don't have satellites". Icarus 316: 191–204. December 2018. doi:10.1016/j.icarus.2018.02.017. Bibcode: 2018Icar..316..191M. "Examination of the physical properties of the 41 largest and most massive main belt asteroids suggests that large asteroids without satellites are intact and their interiors have internal strength. This is consistent with results from the Dawn mission at both Vesta and Ceres. Ceres' volatile-rich composition also is a likely contributor to both the absence of satellites at Ceres and of Ceres meteorites at Earth. These results suggest that collisional disruption creating rubble pile structure is a necessary condition for formation of satellites around main belt asteroids.".
- ↑ "Asteroid or mini-planet? Hubble maps the ancient surface of Vesta". Hubble Space Telescope (Press release). Space Telescope Science Institute. 19 April 1995. STScI-1995-20. Retrieved 16 December 2017.
"Key stages in the evolution of the asteroid Vesta". Hubble Space Telescope (Press release). Space Telescope Science Institute. 19 April 1995. Archived from the original on 7 September 2008. Retrieved 20 October 2007. - ↑ Russel, C.; Raymond, C.; Fraschetti, T.; Rayman, M.; Polanskey, C.; Schimmels, K.; Joy, S. (2005). "Dawn mission and operations". Proceedings of the International Astronomical Union 1 (S229): 97–119. doi:10.1017/S1743921305006691. Bibcode: 2006IAUS..229...97R.
- ↑ Burbine, T.H. (July 1994). "Where are the olivine asteroids in the main belt?". Meteoritics 29 (4): 453. Bibcode: 1994Metic..29..453B.
- ↑ Torppa, J.; Kaasalainen, M.; Michałowski, T.; Kwiatkowski, T.; Kryszczyńska, A.; Denchev, P.; Kowalski, R. (1996). "Shapes and rotational properties of thirty asteroids from photometric data". Icarus 164 (2): 346–383. doi:10.1016/S0019-1035(03)00146-5. Bibcode: 2003Icar..164..346T.
- ↑ Larson, H.P.; Feierberg, M.A.; Lebofsky, L.A. (1983). "The composition of asteroid 2 Pallas and its relation to primitive meteorites". Icarus 56 (3): 398. doi:10.1016/0019-1035(83)90161-6. Bibcode: 1983Icar...56..398L.
- ↑ Barucci, M.A. (2002). "10 Hygiea: ISO Infrared Observations". Icarus 156 (1): 202–210. doi:10.1006/icar.2001.6775. Bibcode: 2002Icar..156..202B. http://www.lesia.obspm.fr/~crovisier/biblio/preprint/bar02_icarus.pdf. Retrieved 21 October 2007.
- ↑ Vernazza, P. et al. (2019-10-28). "A basin-free spherical shape as an outcome of a giant impact on asteroid Hygiea, Supplementary Information". Nature Astronomy 4: 136. doi:10.1038/s41550-019-0915-8. Bibcode: 2020NatAs...4..136V. https://static-content.springer.com/esm/art%3A10.1038%2Fs41550-019-0915-8/MediaObjects/41550_2019_915_MOESM1_ESM.pdf. Retrieved 2019-10-30.
- ↑ Strickland, A. (2019-10-28). "It's an asteroid! No, it's the new smallest dwarf planet in our solar system". https://www.cnn.com/2019/10/28/world/hygiea-smallest-dwarf-planet-intl-scli-trnd-scn/index.html.
- ↑ "About Lightcurves". 4 December 2018. http://alcdef.org/.
- ↑ Rossi, Alessandro (20 May 2004). "The mysteries of the asteroid rotation day". The Spaceguard Foundation. http://spaceguard.iasf-roma.inaf.it/tumblingstone/issues/current/eng/ast-day.htm.
- ↑ "University of Hawaii astronomer and colleagues find evidence that asteroids change color as they age". Institute for Astronomy (Press release). University of Hawaii. 19 May 2005. Retrieved 27 February 2013.
- ↑ Courtland, Rachel (30 April 2009). "Sun damage conceals asteroids' true ages". New Scientist. https://www.newscientist.com/article/dn17062-sun-damage-conceals-asteroids-true-ages.html. Retrieved 27 February 2013.
- ↑ Conrad, A.R.; Dumas, C.; Merline, W.J.; Drummonf, J.D.; Campbell, R.D.; Goodrich, R.W. et al. (2007). "Direct measurement of the size, shape, and pole of 511 Davida with Keck AO in a single night". Icarus 191 (2): 616–627. doi:10.1016/j.icarus.2007.05.004. Bibcode: 2007Icar..191..616C. http://www2.keck.hawaii.edu/inst/people/conrad/research/pub/d511.pdf.
- ↑ 91.0 91.1 91.2 Marchi, S.; Ermakov, A. I.; Raymond, C. A.; Fu, R. R.; O'Brien, D. P.; Bland, M. T.; Ammannito, E.; De Sanctis, M. C. et al. (26 July 2016). "The missing large impact craters on Ceres". Nature Communications 7: 12257. doi:10.1038/ncomms12257. PMID 27459197. Bibcode: 2016NatCo...712257M.
- ↑ David A. Williams, T. Kneiss (December 2018). "The geology of the Kerwan quadrangle of dwarf planet Ceres: Investigating Ceres' oldest, largest impact basin". Icarus 316: 99–113. doi:10.1016/j.icarus.2017.08.015. Bibcode: 2018Icar..316...99W. https://www.sciencedirect.com/science/article/abs/pii/S0019103516305632. Retrieved 16 August 2021.
- ↑ "Asteroid or mini-planet? Hubble maps the ancient surface of Vesta". HubbleSite (Press release). News Center / Release Images. Space Telescope Science Institute. 19 April 1995. Retrieved 27 January 2015.
- ↑ Soter, Steven (16 August 2006). "What is a Planet?". The Astronomical Journal 132 (6): 2513–2519. doi:10.1086/508861. Bibcode: 2006AJ....132.2513S. https://arxiv.org/ftp/astro-ph/papers/0608/0608359.pdf. Retrieved 25 December 2017.
- ↑ P. Vernazza et al. (2021) VLT/SPHERE imaging survey of the largest main-belt asteroids: Final results and synthesis. Astronomy & Astrophysics 54, A56
- ↑ Descamps, P.; Marchis, F.; Berthier, J.; Emery, J.P.; Duchêne, G.; de Pater, I. et al. (February 2011). "Triplicity and physical characteristics of asteroid (216) Kleopatra". Icarus 211 (2): 1022–1033. doi:10.1016/j.icarus.2010.11.016. Bibcode: 2011Icar..211.1022D.
- ↑ 97.0 97.1 97.2 Campins, H.; Hargrove, K (2010). "Water ice and organics on the surface of the asteroid 24 Themis". Nature 464 (7293): 1320–1321. doi:10.1038/nature09029. PMID 20428164. Bibcode: 2010Natur.464.1320C.
- ↑ Cowen, Ron (8 October 2009). "Ice confirmed on an asteroid". Science News. http://www.sciencenews.org/view/generic/id/48174/title/Ice_confirmed_on_an_asteroid. Retrieved 9 October 2009.
- ↑ Atkinson, Nancy (8 October 2009). "More water out there, ice found on an asteroid". http://spacefellowship.com/2009/10/08/more-water-out-there-ice-found-on-an-asteroid/.
- ↑ Rivkin, Andrew S.; Emery, Joshua P. (2010). "Detection of ice and organics on an asteroidal surface". Nature 464 (7293): 1322–1323. doi:10.1038/nature09028. PMID 20428165. Bibcode: 2010Natur.464.1322R.
- ↑ "Watery Asteroid Discovered in Dying Star Points to Habitable Exoplanets – W. M. Keck Observatory". https://keckobservatory.org/watery_asteroid_discovered_in_dying_star_points_to_habitable_exoplanets/.
- ↑ Küppers, Michael; O'Rourke, Laurence (2014). "Localized sources of water vapour on the dwarf planet (1) Ceres". Nature 505 (7484): 525–527. doi:10.1038/nature12918. PMID 24451541. Bibcode: 2014Natur.505..525K.
- ↑ 103.0 103.1 Harrington, J.D. (22 January 2014). "Herschel Telescope Detects Water on Dwarf Planet" (Press release). NASA. Release 14-021. Retrieved 22 January 2014.
- ↑ Daly, Luke; Lee, Martin R.; Hallis, Lydia J.; Ishii, Hope A.; Bradley, John P.; Bland, Phillip A.; Saxey, David W.; Fougerouse, Denis et al. (December 2021). "Solar wind contributions to Earth's oceans" (in en). Nature Astronomy 5 (12): 1275–1285. doi:10.1038/s41550-021-01487-w. ISSN 2397-3366. Bibcode: 2021NatAs...5.1275D. https://www.nature.com/articles/s41550-021-01487-w. Retrieved 30 March 2022.
- ↑ "Earth's water may have been formed by solar winds" (in en). https://www.nhm.ac.uk/discover/news/2021/december/earth-s-water-may-have-been-formed-by-solar-winds.html.
- ↑ Matsumoto, Megumi; Tsuchiyama, Akira; Nakato, Aiko; Matsuno, Junya; Miyake, Akira; Kataoka, Akimasa; Ito, Motoo; Tomioka, Naotaka et al. (November 2019). "Discovery of fossil asteroidal ice in primitive meteorite Acfer 094". Science Advances 5 (11): eaax5078. doi:10.1126/sciadv.aax5078. PMID 31799392. Bibcode: 2019SciA....5.5078M.
- ↑ "Life is sweet: Sugar-packing asteroids may have seeded life on Earth". 19 December 2001. http://www.space.com/scienceastronomy/planetearth/meteor_sugar_011219.html.
- ↑ Reuell, Peter (2019-07-08). "Harvard study suggests asteroids might play key role in spreading life" (in en-US). Harvard Gazette. https://news.harvard.edu/gazette/story/2019/07/harvard-study-suggests-asteroids-might-play-key-role-in-spreading-life/.
- ↑ Callahan, M.P.; Smith, K.E.; Cleaves, H.J.; Ruzica, J.; Stern, J.C.; Glavin, D.P.; House, C.H.; Dworkin, J.P. (11 August 2011). "Carbonaceous meteorites contain a wide range of extraterrestrial nucleobases". PNAS 108 (34): 13995–13998. doi:10.1073/pnas.1106493108. PMID 21836052. Bibcode: 2011PNAS..10813995C.
- ↑ Steigerwald, John (8 August 2011). "NASA researchers: DNA building blocks can be made in space" (Press release). NASA. Archived from the original on 23 June 2015. Retrieved 10 August 2011.
- ↑ "DNA building blocks can be made in space, NASA evidence suggests". 9 August 2011. https://www.sciencedaily.com/releases/2011/08/110808220659.htm.
- ↑ Steigerwald, Bill; Jones, Nancy; Furukawa, Yoshihiro (18 November 2019). "First detection of sugars in meteorites gives clues to origin of life" (Press release). NASA. Retrieved 18 November 2019.
- ↑ Furukawa, Yoshihiro (18 November 2019). "Extraterrestrial ribose and other sugars in primitive meteorites". Proceedings of the National Academy of Sciences of the United States of America 116 (49): 24440–24445. doi:10.1073/pnas.1907169116. PMID 31740594. Bibcode: 2019PNAS..11624440F.
- ↑ Steigerwald, Bill (2022-03-31). "Could the Blueprint for Life Have Been Generated in Asteroids?". http://www.nasa.gov/feature/goddard/2022/life-blueprint-in-asteroids.
- ↑ Zappalà, V.; Bendjoya, Ph.; Cellino, A.; Farinella, P.; Froeschlé, C. (1995). "Asteroid families: Search of a 12,487 asteroid sample using two different clustering techniques". Icarus 116 (2): 291–314. doi:10.1006/icar.1995.1127. Bibcode: 1995Icar..116..291Z.
- ↑ Jewitt, David C.; Sheppard, Scott; Porco, Carolyn (2004). "Jupiter's outer satellites and Trojans". Jupiter: The Planet, Satellites and Magnetosphere. Cambridge University Press. http://www.dtm.ciw.edu/users/sheppard/pub/Sheppard04JupChapter.pdf.
- ↑ "Surface properties of asteroids: A synthesis of polarimetry, radiometry, and spectrophotometry". Icarus 25 (1): 104–130. 1975. doi:10.1016/0019-1035(75)90191-8. Bibcode: 1975Icar...25..104C.
- ↑ "Asteroid taxonomic classifications". University of Arizona Press. 1989. pp. 1139–1150. Bibcode: 1989aste.conf.1139T.
- ↑ Bus, S.J. (2002). "Phase II of the Small Main-belt Asteroid Spectroscopy Survey: A feature-based taxonomy". Icarus 158 (1): 146. doi:10.1006/icar.2002.6856. Bibcode: 2002Icar..158..146B.
- ↑ McSween, Harry Y. Jr. (1999). Meteorites and their Parent Planets (2nd ed.). Oxford University Press. ISBN 978-0-521-58751-8.
- ↑ 121.0 121.1 121.2 David Jewitt. "The Active Asteroids". UCLA, Department of Earth and Space Sciences. http://www2.ess.ucla.edu/~jewitt/mbc.html.
- ↑ Jewitt, David; Hsieh, Henry; Agarwal, Jessica (2015). "The Active Asteroids". in Michel, P.; others. Asteroids IV. University of Arizona. pp. 221–241. doi:10.2458/azu_uapress_9780816532131-ch012. ISBN 978-0-8165-3213-1. Bibcode: 2015aste.book..221J. http://www2.ess.ucla.edu/~jewitt/papers/2015/JHA15.pdf. Retrieved 2020-01-30.
- ↑ Chang, Kenneth; Stirone, Shannon (19 March 2019). "The Asteroid Was Shooting Rocks Into Space. 'Were We Safe in Orbit?' – NASA's Osiris-Rex and Japan's Hayabusa2 spacecraft reached the space rocks they are surveying last year, and scientists from both teams announced early findings on Tuesday (03/19/2019)". The New York Times. https://www.nytimes.com/2019/03/19/science/bennu-ryugu-asteroids.html.
- ↑ "Hubble Observes Six Tails from an Unusual Asteroid". Space Telescope Science Institute (STScI), official YouTube channel for the Hubble Space Telescope. https://www.youtube.com/watch?v=CGgRNWUFfZ0.
- ↑ Hsieh, Henry (January 20, 2004). "133P/Elst-Pizarro". UH Institute for Astronomy. http://www.ifa.hawaii.edu/~hsieh/elstpiz.shtml.
- ↑ "NASA's Hubble Sees Asteroid Spouting Six Comet-Like Tails". Hubblesite. 7 November 2013. http://hubblesite.org/newscenter/archive/releases/2013/52/text/.
- ↑ Jewitt, D.; Agarwal, J.; Weaver, H.; Mutchler, M.; Larson, S. (2013). "The Extraordinary Multi-Tailed Main-Belt Comet P/2013 P5". The Astronomical Journal 778 (1): L21. doi:10.1088/2041-8205/778/1/L21. Bibcode: 2013ApJ...778L..21J.
- ↑ Furfaro, Emily (28 February 2023). "NASA's DART Data Validates Kinetic Impact as Planetary Defense Method". https://www.nasa.gov/feature/nasa-s-dart-data-validates-kinetic-impact-as-planetary-defense-method.
This article incorporates text from this source, which is in the public domain.
- ↑ Li, Jian-Yang; Hirabayashi, Masatoshi; Farnham, Tony L. et al. (1 March 2023). "Ejecta from the DART-produced active asteroid Dimorphos" (in en). Nature 616 (7957): 452–456. doi:10.1038/s41586-023-05811-4. ISSN 1476-4687. PMID 36858074. Bibcode: 2023Natur.616..452L.
- ↑ Witze, Alexandra (1 March 2023). "Asteroid lost 1 million kilograms after collision with DART spacecraft" (in en). Nature 615 (7951): 195. doi:10.1038/d41586-023-00601-4. PMID 36859675. Bibcode: 2023Natur.615..195W. https://www.nature.com/articles/d41586-023-00601-4. Retrieved 9 March 2023.
- ↑ Blue, Charles (3 October 2022). "SOAR Telescope Catches Dimorphos's Expanding Comet-like Tail After DART Impact". NOIRLab. https://noirlab.edu/public/news/noirlab2223/. Retrieved 4 February 2023.
- ↑ Merzdorf, Jessica (15 December 2022). "Early Results from NASA's DART Mission". NASA. https://www.nasa.gov/feature/early-results-from-nasa-s-dart-mission. Retrieved 4 February 2023.
- ↑ Li, Jian-YangExpression error: Unrecognized word "etal". (March 2022). "Ejecta from the DART-produced active asteroid Dimorphos". Nature 616 (7957): 452–456. doi:10.1038/s41586-023-05811-4. PMID 36858074. PMC 10115637. Bibcode: 2023Natur.616..452L. https://assets.researchsquare.com/files/rs-2292349/v1/34562254-db7b-4289-a7ff-238159687528.pdf?c=1669139626. Retrieved 11 March 2023.
- ↑ 134.0 134.1 134.2 134.3 134.4 Michel, Patrick (1 February 2014). "Formation and Physical Properties of Asteroids". Elements 10 (1): 19–24. doi:10.2113/gselements.10.1.19. Bibcode: 2014Eleme..10...19M. https://www.lpi.usra.edu/exploration/education/hsResearch/asteroid_101/Formation_Physical%20Properties_Asteroids.pdf. Retrieved 5 May 2022.
- ↑ "Suspected Asteroid Collision Leaves Odd X-Pattern of Trailing Debris" (in en). https://hubblesite.org/contents/news-releases/2010/news-2010-07.html.
- ↑ Garner, Rob (7 February 2017). "Discoveries | Highlights – Tracking Evolution in the Asteroid Belt". https://www.nasa.gov/content/discoveries-highlights-tracking-evolution-in-the-asteroid-belt.
- ↑ "Hubble Witnesses an Asteroid Mysteriously Disintegrating" (in en). https://hubblesite.org/contents/news-releases/2014/news-2014-15.html.
- ↑ "NASA's Hubble Sees Asteroid Spout Six Comet-like Tails" (in en). https://hubblesite.org/contents/news-releases/2013/news-2013-52.html.
- ↑ "Hubble Images of Asteroids Help Astronomers Prepare for Spacecraft Visit" (in en). https://hubblesite.org/contents/news-releases/2007/news-2007-27.html.
- ↑ "Hubble Reveals Huge Crater on the Surface of the Asteroid Vesta" (in en). https://hubblesite.org/contents/news-releases/1997/news-1997-27.html.
- ↑ "NEAR Shoemaker". NASA. https://solarsystem.nasa.gov/missions/near-shoemaker/in-depth/. Retrieved April 26, 2021.
- ↑ Potter, Sean (2021-11-23). "NASA, SpaceX Launch DART: First Test Mission to Defend Planet Earth". http://www.nasa.gov/press-release/nasa-spacex-launch-dart-first-test-mission-to-defend-planet-earth.
- ↑ Bardan, Roxana (2022-10-11). "NASA Confirms DART Mission Impact Changed Asteroid's Motion in Space". http://www.nasa.gov/press-release/nasa-confirms-dart-mission-impact-changed-asteroid-s-motion-in-space.
- ↑
One or more of the preceding sentences incorporates text from a work now in the public domain: Hille, Karl (2019-10-21). "NASA's Lucy Mission Clears Critical Milestone". NASA. http://www.nasa.gov/feature/goddard/2019/lucy-mission-clears-critical-milestone.
- ↑
One or more of the preceding sentences incorporates text from a work now in the public domain: "Lucy: The First Mission to the Trojan Asteroids". NASA. 21 April 2017. https://www.nasa.gov/mission_pages/lucy/overview/index.
- ↑ "DESTINY+ – Germany and Japan begin new asteroid mission". German Aerospace Center (DLR). 12 November 2020. https://www.dlr.de/content/en/articles/news/2020/04/20201112_destiny-germany-and-japan-begin-new-asteroid-mission.html.
- ↑ Andrew Jones published (2022-05-18). "China to launch Tianwen 2 asteroid-sampling mission in 2025" (in en). https://www.space.com/china-tianwen2-asteroid-sampling-mission-2025-launch.
- ↑ Gibney, Elizabeth (30 April 2019). "China plans mission to Earth's pet asteroid". Nature. doi:10.1038/d41586-019-01390-5. PMID 32346150. https://www.nature.com/articles/d41586-019-01390-5. Retrieved 4 June 2019.
- ↑ Anderson, Matt (1 May 2015). "Mining Near Earth Asteroids". Planetary Sciences Class. http://www.chara.gsu.edu/~thenry/PLANETS/paper.anderson.pdf. Retrieved 13 April 2022.
- ↑ Anderson, Scot W; Christensen, Korey; LaManna, Julia (3 April 2019). "The development of natural resources in outer space". Journal of Energy & Natural Resources Law 37 (2): 227–258. doi:10.1080/02646811.2018.1507343. Bibcode: 2019JENRL..37..227A. https://www.hoganlovells.com/~/media/hogan-lovells/pdf/2018/the_development_of_natural_resouces_in_outer_space_august_2018.pdf. Retrieved 13 April 2022.
- ↑ "How Asteroid Mining Will Work" (in en). 10 November 2000. https://science.howstuffworks.com/asteroid-mining.htm.
- ↑ Wall, Mike (22 January 2013). "Asteroid-Mining Project Aims for Deep-Space Colonies" (in en). https://www.space.com/19368-asteroid-mining-deep-space-industries.html.
- ↑ "Retrieval of Asteroidal Materials". NASA. 1979. http://settlement.arc.nasa.gov/spaceres/IV-2.html.
- ↑ Lee Valentine (2002). "A Space Roadmap: Mine the Sky, Defend the Earth, Settle the Universe". Space Studies Institute. http://ssi.org/reading/papers/space-studies-institute-roadmap/.
- ↑ Didier Massonnet; Benoît Meyssignac (2006). "A captured asteroid : Our David's stone for shielding earth and providing the cheapest extraterrestrial material". Acta Astronautica 59 (1–5): 77–83. doi:10.1016/j.actaastro.2006.02.030. Bibcode: 2006AcAau..59...77M.
- ↑ "Asteroid Retrieval Feasibility Study". Keck Institute for Space Studies, California Institute of Technology, Jet Propulsion Laboratory. 12 April 2012. http://kiss.caltech.edu/study/asteroid/asteroid_final_report.pdf.
- ↑ "Evidence of asteroid mining in our galaxy may lead to the discovery of extraterrestrial civilizations". Smithsonian Institution. 2011-04-05. http://smithsonianscience.org/2011/04/evidence-of-asteroid-mining-in-our-galaxy-may-lead-to-the-discovery-of-extraterrestrial-civilizations/.
- ↑ Gilster, Paul (2011-03-29). "Asteroid Mining: A Marker for SETI?". https://www.centauri-dreams.org/2011/03/29/asteroid-mining-a-marker-for-seti/.
- ↑ Marchis, Franck; Hestroffer, Daniel; Descamps, Pascal; Berthier, Jerome; Bouchez, Antonin H; Campbell, Randall D; Chin, Jason C. Y; van Dam, Marcos A et al. (2011). "Extrasolar Asteroid Mining as Forensic Evidence for Extraterrestrial Intelligence". International Journal of Astrobiology 10 (4): 307–313. doi:10.1017/S1473550411000127. Bibcode: 2011IJAsB..10..307F.
- ↑ "Minor Planet Discover Sites". International Astronomical Union. https://minorplanetcenter.net//iau/lists/MPDiscSites.html.
- ↑ "Unusual Minor Planets". International Astronomical Union. https://minorplanetcenter.net//iau/lists/Unusual.html.
- ↑ "Cumulative Totals". NASA. 20 December 2018. https://cneos.jpl.nasa.gov/stats/totals.html.
- ↑ Mandelbaum, Ryan F. (21 June 2018). "America isn't ready to handle a catastrophic asteroid impact, new report warns". https://gizmodo.com/america-isnt-ready-to-handle-a-catastrophic-asteroid-im-1827014709.
- ↑ "An empirical examination of WISE/NEOWISE asteroid analysis and results". Icarus 314: 64–97. 22 May 2018. doi:10.1016/j.icarus.2018.05.004. Bibcode: 2018Icar..314...64M.
- ↑ Chang, Kenneth (14 June 2018). "Asteroids and adversaries: Challenging what NASA knows about space rocks". The New York Times. https://www.nytimes.com/2018/06/14/science/asteroids-nasa-nathan-myhrvold.html.
- ↑ Threats from Space: A review of U.S. Government efforts to track and mitigate asteroids and meteors (Report). Hearing before the Committee on Science, Space, and Technology. Part I and Part II. House of Representatives. 19 March 2013. p. 147. http://www.gpo.gov/fdsys/pkg/CHRG-113hhrg80552/pdf/CHRG-113hhrg80552.pdf. Retrieved 26 November 2018.
- ↑ Canavan, G. H; Solem, J. C. (1992). "Interception of near-Earth objects". Mercury 21 (3): 107–109. ISSN 0047-6773. Bibcode: 1992Mercu..21..107C. https://www.researchgate.net/publication/253052410.
- ↑ 168.0 168.1 C. D. Hall and I. M. Ross, "Dynamics and Control Problems in the Deflection of Near-Earth Objects", Advances in the Astronautical Sciences, Astrodynamics 1997, Vol. 97, Part I, 1997, pp. 613–631.
- ↑ Solem, J. C. (1993). "Interception of comets and asteroids on collision course with Earth". Journal of Spacecraft and Rockets 30 (2): 222–228. doi:10.2514/3.11531. Bibcode: 1993JSpRo..30..222S. https://digital.library.unt.edu/ark:/67531/metadc1090076/.
- ↑ Solem, J. C.; Snell, C. (1994). "Terminal intercept for less than one orbital period warning ", a chapter in Hazards Due to Comets and Asteroids, Geherels, T., ed. (University of Arizona Press, Tucson), pp. 1013–1034.
- ↑ Solem, J. C. (2000). "Deflection and disruption of asteroids on collision course with Earth". Journal of the British Interplanetary Society 53: 180–196. Bibcode: 2000JBIS...53..180S. http://www.jbis.org.uk/paper.php?p=2000.53.180.
- ↑ Ross, I. M.; Park, S.-Y.; Porter, S. E. (2001). "Gravitational Effects of Earth in Optimizing Delta-V for Deflecting Earth-Crossing Asteroids". Journal of Spacecraft and Rockets 38 (5): 759–764. doi:10.2514/2.3743. https://calhoun.nps.edu/bitstream/handle/10945/30321/AIAA-3743-490.pdf. Retrieved 2019-08-30.
- ↑ David S. F. Portree. "MIT Saves the World: Project Icarus (1967)". Wired. https://www.wired.com/2012/03/mit-saves-the-world-project-icarus-1967/. Retrieved 21 October 2013.
Further reading
- Badescu, Viorel, ed (2013) (in en). Asteroids : prospective energy and material resources. Berlin: Springer. doi:10.1007/978-3-642-39244-3. ISBN 978-3-642-39244-3. Bibcode: 2013aste.book.....B. https://link.springer.com/book/10.1007/978-3-642-39244-3.
- Barnes-Svarney, Patricia L. (2003). Asteroid : Earth destroyer or New Frontier?. Cambridge, Mass.: Basic Books. ISBN 978-0-7382-0885-5.
- Binzel, Richard P.; Gehrels, Tom; Matthews, Mildred Shapley, eds (1989). Asteroids II. Tucson: University of Arizona Press. ISBN 978-0-8165-1123-5.
- Bottke, William F.; Cellino, Alberto; Paolicchi, Paolo et al., eds (2002). Asteroids III. Tucson: University of Arizona Press. ISBN 978-0-8165-4651-0. https://books.google.com/books?id=JwHTyO6IHh8C.
- Kowal, Charles T. (1996). Asteroids : their nature and utilization (2nd ed.). Chichester, England: J. Wiley. ISBN 978-0-471-96039-3.
- Metzger, Philip T.; Sykes, Mark V.; Stern, Alan; Runyon, Kirby (February 2019). "The Reclassification of Asteroids from Planets to Non-Planets". Icarus 319: 21–32. doi:10.1016/j.icarus.2018.08.026. Bibcode: 2019Icar..319...21M.
- Michel, Patrick; DeMeo, Francesca E.; Bottke, William F., eds (2015). Asteroids IV. Houston: Lunar and Planetary Institute. ISBN 978-0-8165-3218-6.
- Peebles, Curtis (2000). Asteroids: A History. Washington,DC: Smithsonian Institution Press. ISBN 978-1-56098-389-7.
External links
- "Alphabetical list of minor planet names". International Astronomical Union. http://www.minorplanetcenter.org/iau/lists/MPNames.html.
- "Asteroid articles in Planetary Science Research Discoveries". University of Hawaii. http://www.psrd.hawaii.edu/Archive/Archive-Asteroids.html.
- "JPL Asteroid Watch site". Jet Propulsion Laboratory. http://www.jpl.nasa.gov/asteroidwatch/.
- NASA Asteroid and Comet Watch site
![]() | Original source: https://en.wikipedia.org/wiki/Asteroid.
Read more |