Biology:Vitamin C
![]() | |
![]() | |
Clinical data | |
---|---|
Pronunciation | /əˈskɔːrbɪk/, /əˈskɔːrbeɪt, -bɪt/ |
Trade names | Ascor, Cecon, Cevalin, others |
Other names | l-ascorbic acid, ascorbic acid, ascorbate |
AHFS/Drugs.com | Monograph |
MedlinePlus | a682583 |
License data | |
Routes of administration | By mouth, intramuscular (IM), intravenous (IV), subcutaneous |
ATC code | |
Legal status | |
Legal status | |
Pharmacokinetic data | |
Bioavailability | Rapid, diminishes as dose increases[4] |
Protein binding | Negligible |
Elimination half-life | Varies according to plasma concentration |
Excretion | Kidney |
Identifiers | |
| |
CAS Number | |
PubChem CID | |
IUPHAR/BPS | |
DrugBank | |
ChemSpider | |
UNII |
|
KEGG | |
ChEBI |
|
ChEMBL |
|
NIAID ChemDB | |
PDB ligand | |
Chemical and physical data | |
Formula | C6H8O6 |
Molar mass | 176.124 g·mol−1 |
3D model (JSmol) | |
Density | 1.694 g/cm3 |
Melting point | 190 to 192 °C (374 to 378 °F) |
Boiling point | 552.7 °C (1,026.9 °F) [5] |
| |
| |
(verify) |
Vitamin C (also known as ascorbic acid and ascorbate) is a water-soluble vitamin found in citrus and other fruits, berries and vegetables, also sold as a dietary supplement[6] and as a topical "serum" ingredient to treat melasma (dark pigment spots) and wrinkles on the face.[7] It is used to prevent and treat scurvy.[6] Vitamin C is an essential nutrient involved in the repair of tissue, the formation of collagen, and the enzymatic production of certain neurotransmitters.[6][8] It is required for the functioning of several enzymes and is important for immune system function.[8][9] It also functions as an antioxidant.[4] Most animals are able to synthesize their own vitamin C. However, apes (including humans) and monkeys (but not all primates), most bats, most fish, some rodents, and certain other animals must acquire it from dietary sources because a gene for a synthesis enzyme has mutations.
Vitamin C may be taken by mouth or by intramuscular, subcutaneous or intravenous injection.[6] Other than for treating scurvy, which is a vitamin C deficiency caused disease, there are a number of diseases and health conditions for which claims have been made for increased risk if moderately deficient, and for benefits from vitamin C supplementation in excess of recommended dietary consumption for people who are not considered vitamin C deficient. The long history of claimed benefits includes the common cold, cancer, heart disease and COVID-19.
Vitamin C is generally well tolerated.[6] Normal doses are safe during pregnancy.[10] Large doses may cause gastrointestinal discomfort, headache, trouble sleeping, and flushing of the skin, [6][11] and the United States Institute of Medicine recommends against consuming large amounts.[8]
Vitamin C was discovered in 1912, isolated in 1928, and in 1933, the first vitamin to be chemically produced.[12] It is on the World Health Organization's List of Essential Medicines.[13] Vitamin C is available as an inexpensive generic and over-the-counter medication.[6][14] In 2021, it was the 255th most commonly prescribed medication in the United States, with more than 1 million prescriptions.[15][16] Partly for its discovery, Albert Szent-Györgyi and Walter Norman Haworth were awarded the 1937 Nobel Prizes in Physiology and Medicine and Chemistry, respectively.[17][18]
Definition
Vitamin C is an essential nutrient for certain animals including humans. The term vitamin C encompasses several vitamers that have vitamin C activity in animals. Ascorbate salts such as sodium ascorbate and calcium ascorbate are used in some dietary supplements. These release ascorbate upon digestion. Ascorbate and ascorbic acid are both naturally present in the body, since the forms interconvert according to pH.
Vitamin C functions as a cofactor in many enzymatic reactions in animals (including humans) that mediate a variety of essential biological functions, including wound healing and collagen synthesis. In humans, vitamin C deficiency leads to impaired collagen synthesis, contributing to the more severe symptoms of scurvy.[8] Another biochemical role of vitamin C is to act as an antioxidant (a reducing agent) by donating electrons to various enzymatic and non-enzymatic reactions.[8] Doing so converts vitamin C to an oxidized state – either as semidehydroascorbic acid or dehydroascorbic acid. These compounds can be restored to a reduced state by glutathione and NADPH-dependent enzymatic mechanisms.[8][19][20]
In plants, vitamin C is a substrate for ascorbate peroxidase. This enzyme utilizes ascorbate to neutralize excess hydrogen peroxide (H2O2) by converting it to water (H2O) and oxygen.[9][19][21]
Deficiency
Plasma vitamin C is the most widely applied test for vitamin C status.[19] Adequate levels are defined as near 50 μmol/L. Hypovitaminosis of vitamin C is defined as less thanb 23 μmol/L, and deficiency as less than 11.4 μmol/L.[22] For people 20 years of age or above, data from the US 2017-18 National Health and Nutrition Examination Survey showed mean serum concentrations of 53.4 μmol/L. The percent of people reported as deficient was 5.9%.[23] Globally, vitamin C deficiency is common in low and middle-income countries, and not uncommon in high income countries. In the latter, prevalence is higher in males than in females, and in the elderly compared to younger populations.[24]
Plasma levels are considered saturated at about 65 μmol/L, achieved by intakes of 100 to 200 mg/day, which are well above the recommended intakes. Even higher oral intake does not further raise plasma nor tissue concentrations because absorption efficiency decreases and any excess that is absorbed is excreted in urine.[19]
Scurvy is a disease resulting from a deficiency of vitamin C. Without this vitamin, collagen made by the body is too unstable to perform its function and several other enzymes in the body do not operate correctly.[9] Scurvy is characterized by spots on and bleeding under the skin, spongy gums, 'corkscrew' hair growth, and poor wound healing. The skin lesions are most abundant on the thighs and legs, and a person with the ailment looks pale, feels depressed, and is partially immobilized. In advanced scurvy there are open, suppurating wounds, loss of teeth, bone abnormalities and, eventually, death.[25]
Notable human dietary studies of experimentally induced scurvy were conducted on conscientious objectors during World War II in Britain and on Iowa state prisoners in the late 1960s to the 1980s. Men in the prison study developed the first signs of scurvy about four weeks after starting the vitamin C-free diet, whereas in the earlier British study, six to eight months were required, possibly due to the pre-loading of this group with a 70 mg/day supplement for six weeks before the scorbutic diet was fed. Men in both studies had blood levels of ascorbic acid too low to be accurately measured by the time they developed signs of scurvy. These studies both reported that all obvious symptoms of scurvy could be completely reversed by supplementation of only 10 mg a day.[26][27]
Diagnostic testing
Vitamin C content in plasma is used to determine vitamin status. For research purposes, concentrations can be assessed in leukocytes and tissues, which are normally maintained at an order of magnitude higher than in plasma via an energy-dependent transport system, depleted slower than plasma cocentrations during dietary deficiency and restored faster during dietary repletion,[8] but these analysis are difficult to measure, and hence not part of standard diagnostic testing.[19][28]
Medical uses
Vitamin C has a definitive role in treating scurvy, which is a disease caused by vitamin C deficiency. Beyond that, a role for vitamin C as prevention or treatment for various diseases is disputed, with reviews often reporting conflicting results. A 2012 Cochrane review reported no effect of vitamin C supplementation on overall mortality.[29] It is on the World Health Organization's List of Essential Medicines[13] and on the World Health Organization's Model Forumulary.[11]
Scurvy
The disease scurvy is caused by vitamin C deficiency and can be treated with vitamin C-containing foods or dietary supplements or injection.[6][8] It takes at least a month of little to no vitamin C before symptoms occur.[26] Early symptoms are malaise and lethargy, progressing to shortness of breath, bone pain, bleeding gums, susceptibility to bruising, poor wound healing, and finally fever, convulsions and eventual death.[6] Until quite late in the disease the damage is reversible, as healthy collagen replaces the defective collagen with vitamin C repletion. Scurvy was known to Hippocrates in the classical era.[30] The disease was shown to be prevented by citrus fruits in an early controlled trial by a Royal Navy surgeon, James Lind, in 1747, on board HMS Salisbury.[31] From 1796 onward, lemon juice was issued to all Royal Navy crewmen.[32][31]
Sepsis
People in sepsis or septic shock may have micronutrient deficiencies, including low levels of vitamin C.[33] Reviews mention that an intake of 3.0 g/day, which requires intravenous administration, may needed to maintain normal plasma concentrations in people with sepsis or severe burn injury.[34][35] Sepsis mortality is reduced with administration of intravenous vitamin C, but not septic shock.[36][37]
Common cold
Research on vitamin C in the common cold has been divided into effects on prevention, duration, and severity. A Cochrane review which looked at at least 200 mg/day concluded that vitamin C taken on a regular basis was not effective in prevention of the common cold. Restricting analysis to trials that used at least 1000 mg/day also saw no prevention benefit. However, taking vitamin C on a regular basis did reduce the average duration of the illness by 8% in adults and 14% in children, and also reduced the severity of colds.[38] In a more recent meta-analysis, vitamin C taken on a regular basis reduced the duration of severe symptoms but had no effect on the duration of mild symptoms.[39]
A subset of trials looked at therapeutic use, meaning that the vitamin was not started unless the people started to feel the beginnings of a cold. In these, vitamin C did not affect the duration or severity of the illness.[38]
Vitamin C distributes readily in high concentrations into immune cells, has antimicrobial and natural killer cell activities, promotes lymphocyte proliferation, and is consumed quickly during infections, effects suggesting a prominent role in immune system regulation.[40] The European Food Safety Authority found a cause and effect relationship exists between the dietary intake of vitamin C and functioning of a normal immune system in adults and in children under three years of age.[41][42]
COVID-19
During March through July 2020, vitamin C was the subject of more US FDA warning letters than any other ingredient for prevention and/or treatment of COVID-19.[43]
In April 2021, the US National Institutes of Health (NIH) COVID-19 Treatment Guidelines stated that "there are insufficient data to recommend either for or against the use of vitamin C for the prevention or treatment of COVID-19."[44] In an update posted December 2022, the NIH position was unchanged:
- There is insufficient evidence for the COVID-19 Treatment Guidelines Panel (the Panel) to recommend either for or against the use of vitamin C for the treatment of COVID-19 in nonhospitalized patients.
- There is insufficient evidence for the Panel to recommend either for or against the use of vitamin C for the treatment of COVID-19 in hospitalized patients.[45]
Three meta-analyses of people hospitalized with severe COVID-19 - with a high overlap in the clinical trials being included - reported a significant reduction in the risk of all-cause, in-hospital mortality with the administration of vitamin C relative to no vitamin C. There were no significant differences in ventilation incidence, hospitalization duration or length of intensive care unit stay between the two groups. The majority of the trials used intravenous administration of the vitamin.[46][47][48] Acute kidney injury was lower in people treated with vitamin C treatment. There were no differences in the frequency of other adverse events due to the vitamin.[48] All three journal articles concluded that further large-scale studies are needed to affirm its mortality benefits before issuing updated guidelines and recommendations.[46][47][48]
Cancer
There is no evidence that vitamin C supplementation reduces the risk of lung cancer in healthy people or those at high risk due to smoking or asbestos exposure.[49] A second meta-analysis found no effect on the risk of prostate cancer.[50] Two meta-analyses evaluated the effect of vitamin C supplementation on the risk of colorectal cancer. One found a weak association between vitamin C consumption and reduced risk, and the other found no effect from supplementation.[51][52] A 2011 meta-analysis failed to find support for the prevention of breast cancer with vitamin C supplementation,[53] but a second study concluded that vitamin C may be associated with increased survival in those already diagnosed.[54]
A 2015 meta-analysis showed no antitumor effect nor improvement in quality-of-life measures from high-dose vitamin C, either oral or intravenous.[55] However, there is research investigating whether intravenous vitamin C administration as a co-treatment will suppress cancer stem cells, which are responsible for tumor recurrence, metastasis and chemoresistance.[56][57]
Cardiovascular disease
A review concluded there was no evidence that vitamin C supplementation decreases the risk cardiovascular disease.[58] An earlier review reported an association between higher circulating vitamin C levels or dietary vitamin C and a lower risk of stroke,[59] and another review found a positive effect of vitamin C on endothelial dysfunction when taken at doses greater than 500 mg per day. The endothelium is a layer of cells that line the interior surface of blood vessels.[60]
Blood pressure
Serum vitamin C was reported to be 15.13 μmol/L lower in people with hypertension compared to normotensives. The vitamin was inversely associated with both systolic blood pressure (SBP) and diastolic blood pressure (DBP).[61] Two meta-analyses of intervention clinical trials with oral supplementation of the vitamin reported a very modest but statistically significant decrease in SBP in people with hypertension.[62][63] The more recent meta-analysis of the two stated that the median supplementation of vitamin C was 757.5 mg/d[63] The proposed explanation is that vitamin C increases intracellular concentrations of tetrahydrobiopterin, an endothelial nitric oxide synthase cofactor that promotes the production of nitric oxide, which is a potent vasodilator. Vitamin C supplementation might also reverse the nitric oxide synthase inhibitor NG-monomethyl-L-arginine 1, and there is also evidence cited that vitamin C directly enhances the biological activity of nitric oxide.[62]
Type 2 diabetes
A 2021 review concluded that vitamin C supplementation cannot be recommended for management of type 2 diabetes.[64] In contrast, a 2023 review found that supplementation with high doses of vitamin C (one gram or more per day) over 12 weeks or longer can decrease blood glucose, insulin, and hemoglobin A1c in people with type 2 diabetes.[65]
Iron deficiency
One of the causes of iron-deficiency anemia is reduced absorption of iron. Iron absorption can be enhanced through ingestion of vitamin C alongside iron-containing food or supplements. Vitamin C helps to keep iron in the reduced ferrous state, which is more soluble and more easily absorbed.[66]
Topical application to prevent signs of skin aging
Human skin contains vitamin C, which supports collagen synthesis, decreases collagen degradation, and assists in antioxidant protection against UV-induced photo-aging, including photocarcinogenesis. This knowledge is often used as a rationale for the marketing of vitamin C as a topical "serum" ingredient to prevent or treat facial skin aging, melasma (dark pigmented spots) and wrinkles. The purported mechanism is that it functions as an antioxidant, neutralizing free radicals from sunlight exposure, air pollutants or normal metabolic processes.[7] The efficacy of topical treatment, as opposed to oral intake is poorly understood.[67][68] Two reviews published in 2023 characterize the clinical trial literature as insufficient to support health claims, one reason being put forward was that "All the studies used vitamin C in combination with other ingredients or therapeutic mechanisms, thereby complicating any specific conclusions regarding the efficacy of vitamin C.[69] A systematic review noted that only a few clinical trials met design criteria. It reported smoother and less wrinkled skin and significant lightening of skin, but concluded that more studies are needed.[70]
Other diseases
Several reviews reported lower vitamin C concentrations in people with cognitive impairment and Alzheimer's disease compared to people with normal cognition. Some of these reported multiple vitamin shortfalls and none were reviews of intervention trials.[71][72][73][74] A 2010 review found no role for vitamin C supplementation in the treatment of rheumatoid arthritis.[75] Vitamin C supplementation does not prevent or slow the progression of age-related cataract.[76] A systematic review reported that low intake and low serum concentration was associated with greater progression of periodontal disease.[77]
Adverse effects
Vitamin C is a water-soluble vitamin,[19] with dietary excesses poorly absorbed,[4] and excesses in the blood rapidly excreted in the urine, so it exhibits low acute toxicity.[9] More than two to three grams, consumed orally, may cause nausea, abdominal cramps and diarrhea. These effects are attributed to the osmotic effect of unabsorbed vitamin C passing through the intestine.[8] In theory, high vitamin C intake may cause excessive absorption of iron. A summary of reviews of supplementation in healthy subjects did not report this problem, but left as untested the possibility that individuals with hereditary hemochromatosis might be adversely affected.[8]
There is a longstanding belief among the mainstream medical community that vitamin C increases risk of kidney stones.[78] "Reports of kidney stone formation associated with excess ascorbic acid intake are limited to individuals with renal disease".[8] A review states that "data from epidemiological studies do not support an association between excess ascorbic acid intake and kidney stone formation in apparently healthy individuals",[79] although one large, multi-year trial did report a nearly two-fold increase in kidney stones in men who regularly consumed a vitamin C supplement.[80]
There is extensive research on the purported benefits of intravenous vitamin C for treatment of sepsis,[34] severe COVID-19[46][47] and cancer.[55] Reviews list trials with doses as high as 24 grams per day.[46] Concerns about possible adverse effects are that intravenous high-dose vitamin C leads to a supraphysiological level of vitamin C followed by oxidative degradation to dehydroascorbic acid and hence to oxalate, increasing the risk of oxalate kidney stones and oxalate nephropathy. The risk may be higher in people with renal impairment, as kidneys efficiently excrete excess vitamin C. Second, treatment with high dose vitamin C should be avoided in patients with glucose-6-phosphate dehydrogenase deficiency as it can lead to acute hemolysis. Third, treatment might interfere with the accuracy of glucometer measurement of blood glucose levels, as both vitamin C and glucose have similar molecular structure, which could lead to false high blood glucose readings. Despite all these concerns, meta-analyses of patients in intensive care for sepsis, septic shock, COVID-19 and other acute conditions reported no increase in new-onset kidney stones, acute kidney injury or requirement for renal replacement therapy for patients receiving short-term, high-dose, intravenous vitamin C treatment. This suggests that intravenous vitamin C is safe under these short-term applications.[81][82][83]
Diet
Recommended levels
Recommendations for vitamin C intake by adults have been set by various national agencies:
- 40 mg/day: India National Institute of Nutrition, Hyderabad[84]
- 45 mg/day or 300 mg/week: the World Health Organization[85]
- 80 mg/day: the European Commission Council on nutrition labeling[86]
- 90 mg/day (males) and 75 mg/day (females): Health Canada 2007[87]
- 90 mg/day (males) and 75 mg/day (females): United States National Academy of Sciences.[8]
- 100 mg/day: Japan National Institute of Health and Nutrition.[88]
- 110 mg/day (males) and 95 mg/day (females): European Food Safety Authority[89]
US vitamin C recommendations (mg per day)[8] | |
---|---|
RDA (children ages 1–3 years) | 15 |
RDA (children ages 4–8 years) | 25 |
RDA (children ages 9–13 years) | 45 |
RDA (girls ages 14–18 years) | 65 |
RDA (boys ages 14–18 years) | 75 |
RDA (adult female) | 75 |
RDA (adult male) | 90 |
RDA (pregnancy) | 85 |
RDA (lactation) | 120 |
UL (adult female) | 2,000 |
UL (adult male) | 2,000 |
In 2000, the chapter on Vitamin C in the North American Dietary Reference Intake was updated to give the Recommended Dietary Allowance (RDA) as 90 milligrams per day for adult men, 75 mg/day for adult women, and setting a Tolerable upper intake level (UL) for adults of 2,000 mg/day.[8] The table (right) shows RDAs for the United States and Canada for children, and for pregnant and lactating women,[8] as well as the ULs for adults.
For the European Union, the EFSA set higher recommendations for adults, and also for children: 20 mg/day for ages 1–3, 30 mg/day for ages 4–6, 45 mg/day for ages 7–10, 70 mg/day for ages 11–14, 100 mg/day for males ages 15–17, 90 mg/day for females ages 15–17. For pregnancy 100 mg/day; for lactation 155 mg/day.[89]
India, on the other hand, has set recommendations much lower: 40 mg/day for ages 1 through adult, 60 mg/day for pregnancy, and 80 mg/day for lactation.[84] Clearly, there is not consensus among countries.
Cigarette smokers and people exposed to secondhand smoke have lower serum vitamin C levels than nonsmokers.[22] The thinking is that inhalation of smoke causes oxidative damage, depleting this antioxidant vitamin.[8] The US Institute of Medicine estimated that smokers need 35 mg more vitamin C per day than nonsmokers, but did not formally establish a higher RDA for smokers.[8] One meta-analysis showed an inverse relationship between vitamin C intake and lung cancer, although it concluded that more research is needed to confirm this observation.[90]
The US National Center for Health Statistics conducts biannual National Health and Nutrition Examination Survey (NHANES) to assess the health and nutritional status of adults and children in the United States. Some results are reported as What We Eat In America. The 2013–2014 survey reported that for adults ages 20 years and older, men consumed on average 83.3 mg/d and women 75.1 mg/d. This means that half the women and more than half the men are not consuming the RDA for vitamin C.[91] The same survey stated that about 30% of adults reported they consumed a vitamin C dietary supplement or a multi-vitamin/mineral supplement that included vitamin C, and that for these people total consumption was between 300 and 400 mg/d.[92]
Tolerable upper intake level
In 2000, the Institute of Medicine of the US National Academy of Sciences set a Tolerable upper intake level (UL) for adults of 2,000 mg/day. The amount was chosen because human trials had reported diarrhea and other gastrointestinal disturbances at intakes of greater than 3,000 mg/day. This was the Lowest-Observed-Adverse-Effect Level (LOAEL), meaning that other adverse effects were observed at even higher intakes. ULs are progressively lower for younger and younger children.[8] In 2006, the European Food Safety Authority (EFSA) also pointed out the disturbances at that dose level, but reached the conclusion that there was not sufficient evidence to set a UL for vitamin C,[93] as did the Japan National Institute of Health and Nutrition in 2010.[88]
Food labeling
For US food and dietary supplement labeling purposes, the amount in a serving is expressed as a percent of Daily Value (%DV). For vitamin C labeling purposes, 100% of the Daily Value was 60 mg, but as of May 27, 2016, it was revised to 90 mg to bring it into agreement with the RDA.[94][95] A table of the old and new adult daily values is provided at Reference Daily Intake.
European Union regulations require that labels declare energy, protein, fat, saturated fat, carbohydrates, sugars, and salt. Voluntary nutrients may be shown if present in significant amounts. Instead of Daily Values, amounts are shown as percent of Reference Intakes (RIs). For vitamin C, 100% RI was set at 80 mg in 2011.[96]
Sources
Although also present in other plant-derived foods, the richest natural sources of vitamin C are fruits and vegetables.[9] The vitamin is the most widely taken dietary supplement.[9]
Plant sources
"While plant foods are generally a good source of vitamin C, the amount in foods of plant origin depends on the variety of the plant, soil condition, climate where it grew, length of time since it was picked, storage conditions, and method of preparation."[97] As one example, organically farmed citrus may have a higher vitamin C content than conventionally farmed citrus.[97] The following table is approximate and shows the relative abundance in different raw plant sources.[98][99] The amount is given in milligrams per 100 grams of the edible portion of the fruit or vegetable:
Raw plant source[100] | Amount (mg / 100g) |
---|---|
Kakadu plum | 1000–5300[101] |
Camu camu | 2800[99][102] |
Acerola | 1677[103] |
Indian gooseberry | 445[104][105] |
Rose hip | 426 |
Common sea-buckthorn | 400[106] |
Guava | 228 |
Blackcurrant | 200 |
Yellow bell pepper/capsicum | 183 |
Red bell pepper/capsicum | 128 |
Kale | 120 |
Broccoli | 90 |
Kiwifruit | 90 |
Raw plant source[100] | Amount (mg / 100g) |
---|---|
Green bell pepper/capsicum | 80 |
Brussels sprouts | 80 |
Loganberry, redcurrant | 80 |
Cloudberry, elderberry | 60 |
Strawberry | 60 |
Papaya | 60 |
Orange, lemon | 53 |
Cauliflower | 48 |
Pineapple | 48 |
Cantaloupe | 40 |
Passion fruit, raspberry | 30 |
Grapefruit, lime | 30 |
Cabbage, spinach | 30 |
Raw plant source[100] | Amount (mg / 100g) |
---|---|
Mango | 28 |
Blackberry, cassava | 21 |
Potato | 20 |
Honeydew melon | 20 |
Tomato | 14 |
Cranberry | 13 |
Blueberry, grape | 10 |
Apricot, plum, watermelon | 10 |
Avocado | 8.8 |
Onion | 7.4 |
Cherry, peach | 7 |
Apple | 6 |
Carrot, asparagus | 6 |
Animal sources
Compared to plant sources, animal-sourced foods do not provide so great an amount of vitamin C, and what there is, is largely destroyed by the heat used when it is cooked. For example, raw chicken liver contains 17.9 mg/100 g, but fried, the content is reduced to 2.7 mg/100 g. Vitamin C is present in human breast milk at 5.0 mg/100 g. Cow's milk contains 1.0 mg/100 g, but the heat of pasteurization destroys it.[107]
Food preparation
Vitamin C chemically decomposes under certain conditions, many of which may occur during the cooking of food. Vitamin C concentrations in various food substances decrease with time in proportion to the temperature at which they are stored.[108] Cooking can reduce the vitamin C content of vegetables by around 60%, possibly due to increased enzymatic destruction.[109] Longer cooking times may add to this effect.[110] Another cause of vitamin C loss from food is leaching, which transfers vitamin C to the cooking water, which is decanted and not consumed.[111]
Supplements
Vitamin C dietary supplements are available as tablets, capsules, drink mix packets, in multi-vitamin/mineral formulations, in antioxidant formulations, and as crystalline powder.[6] Vitamin C is also added to some fruit juices and juice drinks. Tablet and capsule content ranges from 25 mg to 1500 mg per serving. The most commonly used supplement compounds are ascorbic acid, sodium ascorbate and calcium ascorbate.[6] Vitamin C molecules can also be bound to the fatty acid palmitate, creating ascorbyl palmitate, or else incorporated into liposomes.[112]
Food fortification
Countries fortify foods with nutrients to address known deficiencies.[113] While many countries mandate or have voluntary programs to fortify wheat flour, maize (corn) flour or rice with vitamins,[114] none include vitamin C in those programs.[114] As described in Vitamin C Fortification of Food Aid Commodities (1997), the United States provides rations to international food relief programs, later under the asupices of the Food for Peace Act and the Bureau for Humanitarian Assistance.[115] Vitamin C is added to corn-soy blend and wheat-soy blend products at 40 mg/100 grams. (along with minerals and other vitamins). Supplemental rations of these highly fortified, blended foods are provided to refugees and displaced persons in camps and to beneficiaries of development feeding programs that are targeted largely toward mothers and children.[111] The report adds: "The stability of vitamin C (L-ascorbic acid) is of concern because this is one of the most labile vitamins in foods. Its main loss during processing and storage is from oxidation, which is accelerated by light, oxygen, heat, increased pH, high moisture content (water activity), and the presence of copper or ferrous salts. To reduce oxidation, the vitamin C used in commodity fortification is coated with ethyl cellulose (2.5 percent). Oxidative losses also occur during food processing and preparation, and additional vitamin C may be lost if it dissolves into cooking liquid and is then discarded."[111]
As food preservation additives
Ascorbic acid and some of its salts and esters are common additives added to various foods, such as canned fruits, mostly to slow oxidation and enzymatic browning.[116] It may be used as a flour treatment agent used in breadmaking.[117] As food additives, they are assigned E numbers, with safety assessment and approval the responsibility of the European Food Safety Authority.[118] The relevant E numbers are:
- E300 ascorbic acid (approved for use as a food additive in the UK,[119] US[120] Canada,[121] Australia and New Zealand[122])
- E301 sodium ascorbate (approved for use as a food additive in the UK,[119] US,[123] Canada,[121] Australia and New Zealand[122])
- E302 calcium ascorbate (approved for use as a food additive in the UK,[119] US[120] Canada,[121] Australia and New Zealand[122])
- E303 potassium ascorbate (approved in Australia and New Zealand,[122] but not in the UK, US or Canada)
- E304 fatty acid esters of ascorbic acid such as ascorbyl palmitate (approved for use as a food additive in the UK,[119] US,[120] Canada,[121] Australia and New Zealand[122])
The stereoisomers of Vitamin C have a similar effect in food despite their lack of efficacy in humans. They include erythorbic acid and its sodium salt (E315, E316).[119]
Pharmacology
Pharmacodynamics is the study of how the drug – in this instance vitamin C – affects the organism, whereas pharmacokinetics is the study of how an organism affects the drug. Pharmacodynamics includes enzymes for which vitamin C is a cofactor, with function potentially compromised in a deficiency state, and any enzyme cofactor or other physiological function affected by administration of vitamin C, orally or injected, in excess of normal requirements.
Pharmacodynamics
Vitamin C performs numerous physiological functions. It serves as an enzyme substrate or cofactor and an electron donor antioxidant. The enzymatic functions include the synthesis of collagen, carnitine, and neurotransmitters; the synthesis and catabolism of tyrosine; and the metabolism of microsomes.[20] In nonenzymatic functions it acts as a reducing agent, donating electrons to oxidized molecules and preventing oxidation in order to keep iron and copper atoms in their reduced states.[19]
Vitamin C functions as a cofactor for the following enzymes:[19]
- Three groups of enzymes (prolyl-3-hydroxylases, prolyl-4-hydroxylases, and lysyl hydroxylases) that are required for the hydroxylation of proline and lysine in the synthesis of collagen. These reactions add hydroxyl groups to the amino acids proline or lysine in the collagen molecule via prolyl hydroxylase and lysyl hydroxylase, both requiring vitamin C as a cofactor. The role of vitamin C as a cofactor is to oxidize prolyl hydroxylase and lysyl hydroxylase from Fe2+ to Fe3+ and to reduce it from Fe3+ to Fe2+. Hydroxylation allows the collagen molecule to assume its triple helix structure, and thus vitamin C is essential to the development and maintenance of scar tissue, blood vessels, and cartilage.
- Two enzymes (ε-N-trimethyl-L-lysine hydroxylase and γ-butyrobetaine hydroxylase) are necessary for synthesis of carnitine. Carnitine is essential for the transport of fatty acids into mitochondria for ATP generation.
- Hypoxia-inducible factor-proline dioxygenase enzymes (isoforms: EGLN1, EGLN2, and EGLN3) allows cells to respond physiologically to low concentrations of oxygen.
- Dopamine beta-hydroxylase participates in the biosynthesis of norepinephrine from dopamine.
- Peptidylglycine alpha-amidating monooxygenase amidates peptide hormones by removing the glyoxylate residue from their c-terminal glycine residues. This increases peptide hormone stability and activity.
Pharmacokinetics
Ascorbic acid is absorbed in the body by both active transport and simple diffusion. From the US National Institutes of Health: [In humans] "Approximately 70%–90% of vitamin C is absorbed at moderate intakes of 30–180 mg/day. However, at doses above 1,000 mg/day, absorption falls to less than 50%."[4] Sodium-Dependent Active Transport – Sodium-Ascorbate Co-Transporters (SVCTs) and Hexose transporters (GLUTs) – are the two transporter proteins required for active absorption. SVCT1 and SVCT2 import the reduced form of ascorbate across plasma membranes.[124] GLUT1 and GLUT3 are glucose transporters, and transfer only the dehydroascorbic acid (DHA) form of vitamin C.[125] Although dehydroascorbic acid is absorbed in higher rate than ascorbate, the amount of dehydroascorbic acid found in plasma and tissues under normal conditions is low, as cells rapidly reduce dehydroascorbic acid to ascorbate.[126]
SVCTs appear to be the predominant system for vitamin C transport in the body,[124] the notable exception being red blood cells, which lose SVCT proteins during maturation.[127] In both vitamin C synthesizers (example: rat) and non-synthesizers (example: human) cells with few exceptions maintain ascorbic acid concentrations much higher than the approximately 50 micromoles/liter (µmol/L) found in plasma. For example, the ascorbic acid content of pituitary and adrenal glands can exceed 2,000 µmol/L, and muscle is at 200–300 µmol/L.[128] The known coenzymatic functions of ascorbic acid do not require such high concentrations, so there may be other, as yet unknown functions. A consequence of all this high concentration organ content is that plasma vitamin C is not a good indicator of whole-body status, and people may vary in the amount of time needed to show symptoms of deficiency when consuming a diet very low in vitamin C.[128]
Excretion can be as ascorbic acid, via urine. In humans, during times of low dietary intake, vitamin C is reabsorbed by the kidneys rather than excreted. This salvage process delays onset of deficiency. Only when plasma concentrations are 1.4 mg/dL or higher does re-absorption decline and the excess amounts pass freely into the urine.[129] Ascorbic acid also converts (reversibly) to dehydroascorbate (DHA) and from that compound non-reversibly to 2,3-diketogulonate and then oxalate. These three compounds are also excreted via urine. Humans are better than guinea pigs at converting DHA back to ascorbate, and thus take much longer to become vitamin C deficient.[130]
Chemistry
The name "vitamin C" always refers to the l-enantiomer of ascorbic acid and its oxidized forms, such as dehydroascorbate (DHA). Therefore, unless written otherwise, "ascorbate" and "ascorbic acid" refer in the nutritional literature to l-ascorbate and l-ascorbic acid respectively. Ascorbic acid is a weak sugar acid structurally related to glucose. In biological systems, ascorbic acid can be found only at low pH, but in solutions above pH 5 is predominantly found in the ionized form, ascorbate. All of these molecules have vitamin C activity and thus are used synonymously with vitamin C, unless otherwise specified.[19]
Numerous analytical methods have been developed for ascorbic acid detection. For example, vitamin C content of a food sample such as fruit juice can be calculated by measuring the volume of the sample required to decolorize a solution of dichlorophenolindophenol (DCPIP) and then calibrating the results by comparison with a known concentration of vitamin C.[131][132]
Synthesis
Most animals and plants are able to synthesize vitamin C through a sequence of enzyme-driven steps, which convert monosaccharides to vitamin C. Yeasts do not make l-ascorbic acid but rather its stereoisomer, erythorbic acid.[133] In plants, synthesis is accomplished through the conversion of mannose or galactose to ascorbic acid.[134][135] In animals, the starting material is glucose. In some species that synthesize ascorbate in the liver (including mammals and perching birds), the glucose is extracted from glycogen; ascorbate synthesis is a glycogenolysis-dependent process.[136] In humans and in animals that cannot synthesize vitamin C, the enzyme l-gulonolactone oxidase (GULO), which catalyzes the last step in the biosynthesis, is highly mutated and non-functional.[137][138][139][140]
Animal synthesis
There is some information on serum vitamin C concentrations maintained in animal species that are able to synthesize vitamin C. One study of several breeds of dogs reported an average of 35.9 μmol/L.[141] A report on goats, sheep and cattle reported ranges of 100–110, 265–270 and 160–350 μmol/L, respectively.[142]
The biosynthesis of ascorbic acid in vertebrates starts with the formation of UDP-glucuronic acid. UDP-glucuronic acid is formed when UDP-glucose undergoes two oxidations catalyzed by the enzyme UDP-glucose 6-dehydrogenase. UDP-glucose 6-dehydrogenase uses the co-factor NAD+ as the electron acceptor. The transferase UDP-glucuronate pyrophosphorylase removes a UMP and glucuronokinase, with the cofactor ADP, removes the final phosphate leading to d-glucuronic acid. The aldehyde group of this compound is reduced to a primary alcohol using the enzyme glucuronate reductase and the cofactor NADPH, yielding l-gulonic acid. This is followed by lactone formation—utilizing the hydrolase gluconolactonase—between the carbonyl on C1 and hydroxyl group on C4. l-Gulonolactone then reacts with oxygen, catalyzed by the enzyme L-gulonolactone oxidase (which is nonfunctional in humans and other Haplorrhini primates; see Unitary pseudogenes) and the cofactor FAD+. This reaction produces 2-oxogulonolactone (2-keto-gulonolactone), which spontaneously undergoes enolization to form ascorbic acid.[135][143][130] Reptiles and older orders of birds make ascorbic acid in their kidneys. Recent orders of birds and most mammals make ascorbic acid in their liver.[135]
Non-synthesizers
Some mammals have lost the ability to synthesize vitamin C, including simians and tarsiers, which together make up one of two major primate suborders, Haplorhini. This group includes humans. The other more primitive primates (Strepsirrhini) have the ability to make vitamin C. Synthesis does not occur in some species in the rodent family Caviidae, which includes guinea pigs and capybaras, but does occur in other rodents, including rats and mice.[144]
Synthesis does not occur in most bat species,[145] but there are at least two species, frugivorous bat Rousettus leschenaultii and insectivorous bat Hipposideros armiger, that retain (or regained) their ability of vitamin C production.[146][147] A number of species of passerine birds also do not synthesize, but not all of them, and those that do not are not clearly related; it has been proposed that the ability was lost separately a number of times in birds.[148] In particular, the ability to synthesize vitamin C is presumed to have been lost and then later re-acquired in at least two cases.[149] The ability to synthesize vitamin C has also been lost in about 96% of extant fish[150] (the teleosts).[149]
On a milligram consumed per kilogram of body weight basis, simian non-synthesizer species consume the vitamin in amounts 10 to 20 times higher than what is recommended by governments for humans.[151] This discrepancy constituted some of the basis of the controversy on human recommended dietary allowances being set too low.[152] However, simian consumption does not indicate simian requirements. Merck's veterinary manual states that daily intake of vitamin C at 3–6 mg/kg prevents scurvy in non-human primates.[153] By way of comparison, across several countries, the recommended dietary intake for adult humans is in the range of 1–2 mg/kg.
Evolution of animal synthesis
Ascorbic acid is a common enzymatic cofactor in mammals used in the synthesis of collagen, as well as a powerful reducing agent capable of rapidly scavenging a number of reactive oxygen species (ROS). Given that ascorbate has these important functions, it is surprising that the ability to synthesize this molecule has not always been conserved. In fact, anthropoid primates, Cavia porcellus (guinea pigs), teleost fishes, most bats, and some passerine birds have all independently lost the ability to internally synthesize vitamin C in either the kidney or the liver.[154][149] In all of the cases where genomic analysis was done on an ascorbic acid auxotroph, the origin of the change was found to be a result of loss-of-function mutations in the gene that encodes L-gulono-γ-lactone oxidase, the enzyme that catalyzes the last step of the ascorbic acid pathway outlined above.[155] One explanation for the repeated loss of the ability to synthesize vitamin C is that it was the result of genetic drift; assuming that the diet was rich in vitamin C, natural selection would not act to preserve it.[156][157]
In the case of the simians, it is thought that the loss of the ability to make vitamin C may have occurred much farther back in evolutionary history than the emergence of humans or even apes, since it evidently occurred soon after the appearance of the first primates, yet sometime after the split of early primates into the two major suborders Haplorrhini (which cannot make vitamin C) and its sister suborder of non-tarsier prosimians, the Strepsirrhini ("wet-nosed" primates), which retained the ability to make vitamin C.[158] According to molecular clock dating, these two suborder primate branches parted ways about 63 to 60 million years ago.[159] Approximately three to five million years later (58 million years ago), only a short time afterward from an evolutionary perspective, the infraorder Tarsiiformes, whose only remaining family is that of the tarsier (Tarsiidae), branched off from the other haplorrhines.[160][161] Since tarsiers also cannot make vitamin C, this implies the mutation had already occurred, and thus must have occurred between these two marker points (63 to 58 million years ago).[158]
It has also been noted that the loss of the ability to synthesize ascorbate strikingly parallels the inability to break down uric acid, also a characteristic of primates. Uric acid and ascorbate are both strong reducing agents. This has led to the suggestion that, in higher primates, uric acid has taken over some of the functions of ascorbate.[162]
Plant synthesis
There are many different biosynthesis pathways for ascorbic acid in plants. Most of these pathways are derived from products found in glycolysis and other pathways. For example, one pathway ges through the plant cell wall polymers.[137] The plant ascorbic acid biosynthesis pathway most principal seems to be l-galactose. l-Galactose reacts with the enzyme l-galactose dehydrogenase, whereby the lactone ring opens and forms again but with lactone between the carbonyl on C1 and hydroxyl group on C4, resulting in l-galactonolactone.[143] l-Galactonolactone then reacts with the mitochondrial flavoenzyme l-galactonolactone dehydrogenase.[163] to produce ascorbic acid.[143] l-Ascorbic acid has a negative feedback on l-galactose dehydrogenase in spinach.[164] Ascorbic acid efflux by embryo of dicots plants is a well-established mechanism of iron reduction, and a step obligatory for iron uptake.[lower-alpha 1]
All plants synthesize ascorbic acid. Ascorbic acid functions as a cofactor for enzymes involved in photosynthesis, synthesis of plant hormones, as an antioxidant and also regenerator of other antioxidants.[166] Plants use multiple pathways to synthesize vitamin C. The major pathway starts with glucose, fructose or mannose (all simple sugars) and proceeds to L-galactose, L-galactonolactone and ascorbic acid.[166][167] There is feedback regulation in place, in that the presence of ascorbic acid inhibits enzymes in the synthesis pathway.[168] This process follows a diurnal rhythm, so that enzyme expression peaks in the morning to support biosynthesis later on when mid-day sunlight intensity demands high ascorbic acid concentrations.[167] Minor pathways may be specific to certain parts of plants; these can be either identical to the vertebrate pathway (including the GLO enzyme), or start with inositol and get to ascorbic acid via L-galactonic acid to L-galactonolactone.[166]
Industrial synthesis
Vitamin C can be produced from glucose by two main routes. The no longer utilized Reichstein process, developed in the 1930s, used a single fermentation followed by a purely chemical route. The modern two-step fermentation process, originally developed in China in the 1960s, uses additional fermentation to replace part of the later chemical stages. The Reichstein process and the modern two-step fermentation processes both use glucose as the starting material, convert that to sorbitol, and then to sorbose using fermentation.[169] The two-step fermentation process then converts sorbose to 2-keto-l-gulonic acid (KGA) through another fermentation step, avoiding an extra intermediate. Both processes yield approximately 60% vitamin C from the glucose starting point.[170] Researchers are exploring means for one-step fermentation.[171][172]
China produces about 70% of the global vitamin C market. The rest is split among European Union, India and North America. The global market is expected to exceed 141 thousand metric tons in 2024.[173] Cost per metric ton (1000 kg) in US dollars was $2,220 in Shanghia, $2,850 in Hamburg and $3,490 in the US.[174]
History
Scurvy at sea
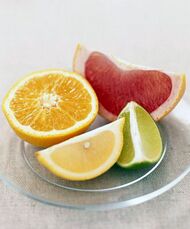
In the 1497 expedition of Vasco da Gama, the curative effects of citrus fruit were known.[175][176] Later, the Portuguese planted fruit trees and vegetables in Saint Helena, a stopping point for homebound voyages from Asia, which sustained passing ships.[177] Authorities occasionally recommended plant food to prevent scurvy during long sea voyages. John Woodall, the first surgeon to the British East India Company, recommended the preventive and curative use of lemon juice in his 1617 book, The Surgeon's Mate.[178] In 1734, the Dutch writer Johann Bachstrom gave the firm opinion, "scurvy is solely owing to a total abstinence from fresh vegetable food, and greens."[179][180] Scurvy had long been a principal killer of sailors during the long sea voyages.[181] According to Jonathan Lamb, "In 1499, Vasco da Gama lost 116 of his crew of 170; In 1520, Magellan lost 208 out of 230;...all mainly to scurvy."[182]

The first attempt to give scientific basis for the cause of this disease was by a ship's surgeon in the Royal Navy, James Lind. While at sea in May 1747, Lind provided some crew members with two oranges and one lemon per day, in addition to normal rations, while others continued on cider, vinegar, sulfuric acid or seawater, along with their normal rations, in one of the world's first controlled experiments.[31] The results showed that citrus fruits prevented the disease. Lind published his work in 1753 in his Treatise on the Scurvy.[32][183]
Fresh fruit was expensive to keep on board, whereas boiling it down to juice allowed easy storage but destroyed the vitamin (especially if boiled in copper kettles).[110] It was 1796 before the British navy adopted lemon juice as standard issue at sea. In 1845, ships in the West Indies were provided with lime juice instead, and in 1860 lime juice was used throughout the Royal Navy, giving rise to the American use of the nickname "limey" for the British.[31] Captain James Cook had previously demonstrated the advantages of carrying "Sour krout" on board, by taking his crew on a 1772-75 Pacific Ocean voyage without losing any of his men to scurvy.[184] For his report on his methods the British Royal Society awarded him the Copley Medal in 1776.[185]
The name antiscorbutic was used in the eighteenth and nineteenth centuries for foods known to prevent scurvy. These foods included lemons, limes, oranges, sauerkraut, cabbage, malt, and portable soup.[186] In 1928, the Canadian Arctic anthropologist Vilhjalmur Stefansson showed that the Inuit avoid scurvy on a diet of largely raw meat. Later studies on traditional food diets of the Yukon First Nations, Dene, Inuit, and Métis of Northern Canada showed that their daily intake of vitamin C averaged between 52 and 62 mg/day,[187] comparable with the Estimated Average Requirement.[8]
Discovery

Vitamin C was discovered in 1912, isolated in 1928 and synthesized in 1933, making it the first vitamin to be synthesized.[12] Shortly thereafter Tadeus Reichstein succeeded in synthesizing the vitamin in bulk by what is now called the Reichstein process.[189] This made possible the inexpensive mass-production of vitamin C. In 1934, Hoffmann–La Roche bought the Reichstein process patent, trademarked synthetic vitamin C under the brand name Redoxon, and began to market it as a dietary supplement.[190][191]
In 1907, a laboratory animal model which would help to identify the antiscorbutic factor was discovered by the Norwegian physicians Axel Holst and Theodor Frølich, who when studying shipboard beriberi, fed guinea pigs their test diet of grains and flour and were surprised when scurvy resulted instead of beriberi. Unknown at that time, this species did not make its own vitamin C (being a caviomorph), whereas mice and rats do.[192] In 1912, the Poland biochemist Casimir Funk developed the concept of vitamins. One of these was thought to be the anti-scorbutic factor. In 1928, this was referred to as "water-soluble C", although its chemical structure had not been determined.[193]

From 1928 to 1932, Albert Szent-Györgyi and Joseph L. Svirbely's Hungarian team, and Charles Glen King's American team, identified the anti-scorbutic factor. Szent-Györgyi isolated hexuronic acid from animal adrenal glands, and suspected it to be the antiscorbutic factor.[194] In late 1931, Szent-Györgyi gave Svirbely the last of his adrenal-derived hexuronic acid with the suggestion that it might be the anti-scorbutic factor. By the spring of 1932, King's laboratory had proven this, but published the result without giving Szent-Györgyi credit for it. This led to a bitter dispute over priority.[194] In 1933, Walter Norman Haworth chemically identified the vitamin as l-hexuronic acid, proving this by synthesis in 1933.[195][196][197][198] Haworth and Szent-Györgyi proposed that L-hexuronic acid be named a-scorbic acid, and chemically l-ascorbic acid, in honor of its activity against scurvy.[198][12] The term's etymology is from Latin, "a-" meaning away, or off from, while -scorbic is from Medieval Latin scorbuticus (pertaining to scurvy), cognate with Old Norse skyrbjugr, French scorbut, Dutch scheurbuik and Low German scharbock.[199] Partly for this discovery, Szent-Györgyi was awarded the 1937 Nobel Prize in Medicine,[17] and Haworth shared that year's Nobel Prize in Chemistry.[18]
In 1957, J. J. Burns showed that some mammals are susceptible to scurvy as their liver does not produce the enzyme l-gulonolactone oxidase, the last of the chain of four enzymes that synthesize vitamin C.[200][201] American biochemist Irwin Stone was the first to exploit vitamin C for its food preservative properties. He later developed the idea that humans possess a mutated form of the l-gulonolactone oxidase coding gene.[202] Stone introduced Linus Pauling to the theory that humans needed to consume vitamin C in quantities far higher than what was considered a recommended daily intake in order to optimize health.[203]
In 2008, researchers discovered that in humans and other primates the red blood cells have evolved a mechanism to more efficiently utilize the vitamin C present in the body by recycling oxidized l-dehydroascorbic acid (DHA) back into ascorbic acid for reuse by the body. The mechanism was not found to be present in mammals that synthesize their own vitamin C.[204]
History of large doses
Vitamin C megadosage is a term describing the consumption or injection of vitamin C in doses comparable to or higher than the amounts produced by the livers of mammals which are able to synthesize vitamin C. An argument for this, although not the actual term, was described in 1970 in an article by Linus Pauling. Briefly, his position was that for optimal health, humans should be consuming at least 2,300 mg/day to compensate for the inability to synthesize vitamin C. The recommendation also fell into the consumption range for gorillas – a non-synthesizing near-relative to humans.[152] A second argument for high intake is that serum ascorbic acid concentrations increase as intake increases until it plateaus at about 190 to 200 micromoles per liter (µmol/L) once consumption exceeds 1,250 milligrams.[205] As noted, government recommendations are a range of 40 to 110 mg/day and normal plasma is approximately 50 µmol/L, so 'normal' is about 25% of what can be achieved when oral consumption is in the proposed megadose range.
Pauling popularized the concept of high dose vitamin C as prevention and treatment of the common cold in 1970. A few years later he proposed that vitamin C would prevent cardiovascular disease, and that 10 grams/day, initially administered intravenously and thereafter orally, would cure late-stage cancer.[206] Mega-dosing with ascorbic acid has other champions, among them chemist Irwin Stone[203] and the controversial Matthias Rath and Patrick Holford, who both have been accused of making unsubstantiated treatment claims for treating cancer and HIV infection.[207][208] The idea that large amounts of intravenous ascorbic acid can be used to treat late-stage cancer or ameliorate the toxicity of chemotherapy is – some forty years after Pauling's seminal paper – still considered unproven and still in need of high quality research.[209][210][55] However, a lack of conclusive evidence has not stopped individual physicians from prescribing intravenous ascorbic acid to people with cancer.[55]
Society and culture
In February 2011, the Swiss Post issued a postage stamp bearing a depiction of a model of a molecule of vitamin C to mark the International Year of Chemistry.[211]
Pharmacopoeias
Notes
References
- ↑ "Ascorbic Acid Injection 500mg/5ml - Summary of Product Characteristics (SmPC)". July 15, 2015. https://www.medicines.org.uk/emc/product/1520/smpc.
- ↑ "Ascorbic Acid 100mg Tablets - Summary of Product Characteristics (SmPC)". October 29, 2018. https://www.medicines.org.uk/emc/product/9615/smpc.
- ↑ "Ascor- ascorbic acid injection". October 2, 2020. https://dailymed.nlm.nih.gov/dailymed/drugInfo.cfm?setid=388aad52-fc01-4784-9791-1dbc80c69306.
- ↑ 4.0 4.1 4.2 4.3 "Fact Sheet for Health Professionals – Vitamin C". Office of Dietary Supplements, US National Institutes of Health. February 11, 2016. https://ods.od.nih.gov/factsheets/VitaminC-HealthProfessional/.
- ↑ "Vitamin C". Royal Society of Chemistry. http://www.chemspider.com/Chemical-Structure.10189562.html.
- ↑ 6.00 6.01 6.02 6.03 6.04 6.05 6.06 6.07 6.08 6.09 6.10 "Ascorbic Acid". The American Society of Health-System Pharmacists. https://www.drugs.com/monograph/ascorbic-acid.html.
- ↑ 7.0 7.1 "Why is topical vitamin C important for skin health?". November 10, 2021. https://www.health.harvard.edu/blog/why-is-topical-vitamin-c-important-for-skin-health-202111102635.
- ↑ 8.00 8.01 8.02 8.03 8.04 8.05 8.06 8.07 8.08 8.09 8.10 8.11 8.12 8.13 8.14 8.15 8.16 8.17 8.18 Institute of Medicine (US) Panel on Dietary Antioxidants Related Compounds (2000). "Vitamin C". Dietary Reference Intakes for Vitamin C, Vitamin e, Selenium, and Carotenoids. Washington, DC: The National Academies Press. pp. 95–185. doi:10.17226/9810. ISBN 978-0-309-06935-9. https://www.nap.edu/read/9810/chapter/7. Retrieved September 1, 2017.
- ↑ 9.0 9.1 9.2 9.3 9.4 9.5 "Vitamin C". Linus Pauling Institute (Micronutrient Information Center, Linus Pauling Institute, Oregon State University, Corvallis, OR). July 1, 2018. http://lpi.oregonstate.edu/mic/vitamins/vitamin-C.
- ↑ "Ascorbic acid Use During Pregnancy". https://www.drugs.com/pregnancy/ascorbic-acid.html.
- ↑ 11.0 11.1 WHO Model Formulary 2008. World Health Organization. 2009. ISBN 9789241547659.
- ↑ 12.0 12.1 12.2 The Role of Food, Agriculture, Forestry and Fisheries in Human Nutrition - Volume IV. EOLSS Publications. 2011. p. 121. ISBN 9781848261952. https://books.google.com/books?id=VJWoCwAAQBAJ&pg=PA121. Retrieved September 17, 2017.
- ↑ 13.0 13.1 The selection and use of essential medicines 2023: web annex A: World Health Organization model list of essential medicines: 23rd list (2023). Geneva: World Health Organization. 2023. WHO/MHP/HPS/EML/2023.02.
- ↑ British national formulary : BNF 76 (76 ed.). Pharmaceutical Press. 2018. p. 1049. ISBN 9780857113382.
- ↑ "The Top 300 of 2021". https://clincalc.com/DrugStats/Top300Drugs.aspx.
- ↑ "Ascorbic Acid - Drug Usage Statistics". https://clincalc.com/DrugStats/Drugs/AscorbicAcid.
- ↑ 17.0 17.1 "The Nobel Prize in Physiology or Medicine 1937". Nobel Media AB. https://www.nobelprize.org/nobel_prizes/medicine/laureates/1937.
- ↑ 18.0 18.1 18.2 "Nobel Prize 1937 to Albert von Szent-Györgyi: identification of vitamin C as the anti-scorbutic factor". Acta Paediatrica 98 (5): 915–9. May 2009. doi:10.1111/j.1651-2227.2009.01239.x. PMID 19239412.
- ↑ 19.0 19.1 19.2 19.3 19.4 19.5 19.6 19.7 19.8 "Vitamin C". Present Knowledge in Nutrition, Eleventh Edition. London, United Kingdom: Academic Press (Elsevier). 2020. pp. 155–70. ISBN 978-0-323-66162-1.
- ↑ 20.0 20.1 Advanced nutrition and human metabolism. Belmont, CA: Thomson Wadsworth. 2005. pp. 260–275. ISBN 978-0-534-55986-1.
- ↑ Ascorbate-Glutathione Pathway and Stress Tolerance in Plants. Springer. September 13, 2010. p. 324. ISBN 978-9-048-19403-2. https://books.google.com/books?id=HUgvF_9EwHcC&pg=PA324. Retrieved August 3, 2017.
- ↑ 22.0 22.1 "Serum vitamin C and the prevalence of vitamin C deficiency in the United States: 2003-2004 National Health and Nutrition Examination Survey (NHANES)". The American Journal of Clinical Nutrition 90 (5): 1252–63. November 2009. doi:10.3945/ajcn.2008.27016. PMID 19675106.
- ↑ "Current Estimates of Serum Vitamin C and Vitamin C Deficiency in the United States". Curr Dev Nutr 7 (5): 1067. June 2021. doi:10.1093/cdn/nzab053_060.
- ↑ "Global Vitamin C Status and Prevalence of Deficiency: A Cause for Concern?". Nutrients 12 (7): 2008. July 2020. doi:10.3390/nu12072008. PMID 32640674.
- ↑ "Scurvy: past, present and future.". The European Journal of Internal Medicine 22 (2): 147–52. April 2011. doi:10.1016/j.ejim.2010.10.006. PMID 21402244.
- ↑ 26.0 26.1 "Experimental scurvy in man". The American Journal of Clinical Nutrition 22 (5): 535–48. May 1969. doi:10.1093/ajcn/22.5.535. PMID 4977512.
- ↑ "Medical experiments carried out in Sheffield on conscientious objectors to military service during the 1939-45 war". International Journal of Epidemiology 35 (3): 556–8. June 2006. doi:10.1093/ije/dyl020. PMID 16510534.
- ↑ "Measurement of intracellular vitamin C levels in human lymphocytes by reverse phase high performance liquid chromatography (HPLC)". Clinical Biochemistry 38 (5): 450–56. May 2005. doi:10.1016/j.clinbiochem.2005.01.018. PMID 15820776.
- ↑ "Antioxidant supplements for prevention of mortality in healthy participants and patients with various diseases". The Cochrane Database of Systematic Reviews 2012 (3): CD007176. March 2012. doi:10.1002/14651858.CD007176.pub2. PMID 22419320.
- ↑ Hippocrates described symptoms of scurvy in book 2 of his Prorrheticorum and in his Liber de internis affectionibus. Cited by James Lind, A Treatise on the Scurvy (3rd ed.). London, England: G. Pearch and W. Woodfall. 1772. p. 285. https://archive.org/details/treatiseonscurvy1772lind/page/285. Symptoms of scurvy were also described by: (i) "49". Naturalis historiae. 3.; and (ii) Strabo, in Geographicorum, book 16. (Cited by "The International Encyclopedia of Surgery". The International Encyclopedia of Surgery. 1. New York, New York: William Wood and Co.. 1881. p. 278. https://books.google.com/books?id=mDV11NpZyNgC&pg=PA278.)
- ↑ 31.0 31.1 31.2 31.3 31.4 "Sailors' scurvy before and after James Lind--a reassessment". Nutrition Reviews 67 (6): 315–32. June 2009. doi:10.1111/j.1753-4887.2009.00205.x. PMID 19519673.
- ↑ 32.0 32.1 A Treatise of the Scurvy. London: A. Millar. 1753. In the 1757 edition of his work, Lind discusses his experiment starting on "A Treatise of the Scurvy". p. 149. https://archive.org/stream/treatiseonscurvy00lind#page/149/mode/1up.
- ↑ "A review of micronutrients in sepsis: the role of thiamine, L-carnitine, vitamin C, selenium and vitamin D". Nutrition Research Reviews 31 (2): 281–90. December 2018. doi:10.1017/S0954422418000124. PMID 29984680.
- ↑ 34.0 34.1 "The outcome of IV vitamin C therapy in patients with sepsis or septic shock: a meta-analysis of randomized controlled trials". Crit Care 27 (1): 109. March 2023. doi:10.1186/s13054-023-04392-y. PMID 36915173.
- ↑ "Vitamin C supplementation in the critically ill patient". Curr Opin Clin Nutr Metab Care 18 (2): 193–201. March 2015. doi:10.1097/MCO.0000000000000148. PMID 25635594.
- ↑ "Association of Oral or Intravenous Vitamin C Supplementation with Mortality: A Systematic Review and Meta-Analysis". Nutrients 15 (8): 1848. April 2023. doi:10.3390/nu15081848. PMID 37111066.
- ↑ "Effect of intravenous vitamin C on adult septic patients: a systematic review and meta-analysis". Front Nutr 10: 1211194. 2023. doi:10.3389/fnut.2023.1211194. PMID 37599680.
- ↑ 38.0 38.1 "Vitamin C for preventing and treating the common cold". The Cochrane Database of Systematic Reviews 2013 (1): CD000980. January 2013. doi:10.1002/14651858.CD000980.pub4. PMID 23440782.
- ↑ "Vitamin C reduces the severity of common colds: a meta-analysis". BMC Public Health 23 (1): 2468. December 2023. doi:10.1186/s12889-023-17229-8. PMID 38082300.
- ↑ "Immune-enhancing role of vitamin C and zinc and effect on clinical conditions". Annals of Nutrition & Metabolism 50 (2): 85–94. 2006. doi:10.1159/000090495. PMID 16373990. http://doc.rero.ch/record/303675/files/S0029665108006927.pdf. Retrieved August 25, 2019.
- ↑ EFSA Panel on Dietetic Products, Nutrition and Allergies (2009). "Scientific Opinion on the substantiation of health claims related to vitamin C and protection of DNA, proteins and lipids from oxidative damage (ID 129, 138, 143, 148), antioxidant function of lutein (ID 146), maintenance of vision (ID 141, 142), collagen formation (ID 130, 131, 136, 137, 149), function of the nervous system (ID 133), function of the immune system (ID 134), function of the immune system during and after extreme physical exercise (ID 144), non-haem iron absorption (ID 132, 147), energy-yielding metabolism (ID 135), and relief in case of irritation in the upper respiratory tract (ID 1714, 1715) pursuant to Article 13(1) of Regulation (EC) No 1924/2006". EFSA Journal 7 (9): 1226. doi:10.2903/j.efsa.2009.1226.
- ↑ EFSA Panel on Dietetic Products, Nutrition and Allergies (2015). "Vitamin C and contribution to the normal function of the immune system: evaluation of a health claim pursuant to Article 14 of Regulation (EC) No 1924/2006". EFSA Journal 13 (11): 4298. doi:10.2903/j.efsa.2015.4298.
- ↑ "Unicorn Poo and Blessed Waters: COVID-19 Quackery and FDA Warning Letters". Ther Innov Regul Sci 55 (1): 239–244. October 2020. doi:10.1007/s43441-020-00224-1. PMID 33001378.
- ↑ "Vitamin C". April 21, 2021. https://www.covid19treatmentguidelines.nih.gov/therapies/supplements/vitamin-c/.
- ↑ "COVID-19 Treatment Guidelines". December 26, 2022. https://www.covid19treatmentguidelines.nih.gov/therapies/supplements/vitamin-c/.
- ↑ 46.0 46.1 46.2 46.3 "The effect of vitamin C on the risk of mortality in patients with COVID-19: a systematic review and meta-analysis of randomized controlled trials". Inflammopharmacology 31 (6): 3357–62. December 2023. doi:10.1007/s10787-023-01200-5. PMID 37071316.
- ↑ 47.0 47.1 47.2 "Association of Vitamin C Treatment with Clinical Outcomes for COVID-19 Patients: A Systematic Review and Meta-Analysis". Healthcare 10 (12): 2456. December 2022. doi:10.3390/healthcare10122456. PMID 36553979.
- ↑ 48.0 48.1 48.2 "Vitamin C Supplementation for the Treatment of COVID-19: A Systematic Review and Meta-Analysis". Nutrients 14 (19): 4217. October 2022. doi:10.3390/nu14194217. PMID 36235869.
- ↑ "Drugs for preventing lung cancer in healthy people". The Cochrane Database of Systematic Reviews 2020 (3): CD002141. March 2020. doi:10.1002/14651858.CD002141.pub3. PMID 32130738.
- ↑ "The effect of supplemental vitamins and minerals on the development of prostate cancer: a systematic review and meta-analysis". Family Practice 28 (3): 243–52. June 2011. doi:10.1093/fampra/cmq115. PMID 21273283.
- ↑ "Dietary intake of vitamins A, C, and E and the risk of colorectal adenoma: a meta-analysis of observational studies". European Journal of Cancer Prevention 22 (6): 529–39. November 2013. doi:10.1097/CEJ.0b013e328364f1eb. PMID 24064545.
- ↑ "Antioxidants in the chemoprevention of colorectal cancer and colorectal adenomas in the general population: a systematic review and meta-analysis". Colorectal Disease 13 (10): 1085–99. October 2011. doi:10.1111/j.1463-1318.2010.02289.x. PMID 20412095.
- ↑ "Retinol, vitamins A, C, and E and breast cancer risk: a meta-analysis and meta-regression". Cancer Causes & Control 22 (10): 1383–96. October 2011. doi:10.1007/s10552-011-9811-y. PMID 21761132.
- ↑ "Vitamin C and survival among women with breast cancer: a meta-analysis". European Journal of Cancer 50 (7): 1223–31. May 2014. doi:10.1016/j.ejca.2014.02.013. PMID 24613622.
- ↑ 55.0 55.1 55.2 55.3 "Is there a role for oral or intravenous ascorbate (vitamin C) in treating patients with cancer? A systematic review". The Oncologist 20 (2): 210–23. February 2015. doi:10.1634/theoncologist.2014-0381. PMID 25601965.
- ↑ "Role of Vitamin C in Targeting Cancer Stem Cells and Cellular Plasticity". Cancers (Basel) 15 (23): 5657. November 2023. doi:10.3390/cancers15235657. PMID 38067361.
- ↑ "Combination Therapy with Vitamin C Could Eradicate Cancer Stem Cells". Biomolecules 10 (1): 79. January 2020. doi:10.3390/biom10010079. PMID 31947879.
- ↑ "Vitamin C supplementation for the primary prevention of cardiovascular disease". The Cochrane Database of Systematic Reviews 2017 (3): CD011114. March 2017. doi:10.1002/14651858.CD011114.pub2. PMID 28301692.
- ↑ "Vitamin C intake, circulating vitamin C and risk of stroke: a meta-analysis of prospective studies". Journal of the American Heart Association 2 (6): e000329. November 2013. doi:10.1161/JAHA.113.000329. PMID 24284213.
- ↑ "Effect of vitamin C on endothelial function in health and disease: a systematic review and meta-analysis of randomized controlled trials". Atherosclerosis 235 (1): 9–20. July 2014. doi:10.1016/j.atherosclerosis.2014.04.004. PMID 24792921.
- ↑ "Association between Serum Vitamin C and the Blood Pressure: A Systematic Review and Meta-Analysis of Observational Studies". Cardiovasc Ther 2020: 4940673. April 2020. doi:10.1155/2020/4940673. PMID 32426036.
- ↑ 62.0 62.1 "Effects of vitamin C supplementation on essential hypertension: A systematic review and meta-analysis". Medicine (Baltimore) 99 (8): e19274. February 2020. doi:10.1097/MD.0000000000019274. PMID 32080138.
- ↑ 63.0 63.1 "Vitamin C supplementation showed greater effects on systolic blood pressure in hypertensive and diabetic patients: an updated systematic review and meta-analysis of randomized clinical trials". Int J Food Sci Nutr 74 (8): 814–25. December 2023. doi:10.1080/09637486.2023.2264549. PMID 37791386. https://figshare.com/articles/journal_contribution/24241426. Retrieved December 23, 2023.
- ↑ "Effects of Vitamin C Supplementation on Glycemic Control and Cardiovascular Risk Factors in People With Type 2 Diabetes: A GRADE-Assessed Systematic Review and Meta-analysis of Randomized Controlled Trials". Diabetes Care 44 (2): 618–30. February 2021. doi:10.2337/dc20-1893. PMID 33472962. https://diabetesjournals.org/care/article/44/2/618/35482/Effects-of-Vitamin-C-Supplementation-on-Glycemic. Retrieved December 21, 2023.
- ↑ "The effects of vitamin C supplementation on glycemic control in patients with type 2 diabetes: A systematic review and meta-analysis". Diabetes and Metabolic Syndrome 17 (8): 102824. August 2023. doi:10.1016/j.dsx.2023.102824. PMID 37523928.
- ↑ "Iron Deficiency Anemia". Med Clin North Am 101 (2): 319–332. March 2017. doi:10.1016/j.mcna.2016.09.004. PMID 28189173.
- ↑ "The Roles of Vitamin C in Skin Health". Nutrients 9 (8): 866. August 2017. doi:10.3390/nu9080866. PMID 28805671.
- ↑ "Topical Vitamin C and the Skin: Mechanisms of Action and Clinical Applications". J Clin Aesthet Dermatol 10 (7): 14–17. July 2017. PMID 29104718.
- ↑ "Clinical Efficacy of Topical Vitamin C on the Appearance of Wrinkles: A Systematic Literature Review". J Drugs Dermatol 22 (9): 898–904. September 2023. doi:10.36849/JDD.7332. PMID 37683066.
- ↑ "Efficacy of topical vitamin C in melasma and photoaging: A systematic review". J Cosmet Dermatol 22 (7): 1938–45. July 2023. doi:10.1111/jocd.15748. PMID 37128827.
- ↑ "Vitamin C status and cognitive function: A systematic review". Nutrients 9 (9): E960. August 30, 2017. doi:10.3390/nu9090960. PMID 28867798.
- ↑ "Plasma nutrient status of patients with Alzheimer's disease: Systematic review and meta-analysis". Alzheimer's & Dementia 10 (4): 485–502. 2014. doi:10.1016/j.jalz.2013.05.1771. PMID 24144963.
- ↑ "Dietary intakes of vitamin E, vitamin C, and β-carotene and risk of Alzheimer's disease: a meta-analysis". Journal of Alzheimer's Disease 31 (2): 253–8. 2012. doi:10.3233/JAD-2012-120349. PMID 22543848.
- ↑ "A critical review of vitamin C for the prevention of age-related cognitive decline and Alzheimer's disease". Journal of Alzheimer's Disease 29 (4): 711–26. 2012. doi:10.3233/JAD-2012-111853. PMID 22366772.
- ↑ "Antioxidants and antiinflammatory dietary supplements for osteoarthritis and rheumatoid arthritis". Alternative Therapies in Health and Medicine 16 (2): 32–40. 2010. PMID 20232616.
- ↑ "Antioxidant vitamin supplementation for preventing and slowing the progression of age-related cataract". The Cochrane Database of Systematic Reviews 6 (6): CD004567. June 2012. doi:10.1002/14651858.CD004567.pub2. PMID 22696344.
- ↑ "Photoacoustic imaging as a tool to probe the tumor microenvironment". Disease Models & Mechanisms 12 (7): dmm039636. July 2019. doi:10.1242/dmm.039636. PMID 31337635.
- ↑ "Battling quackery: attitudes about micronutrient supplements in American academic medicine". Archives of Internal Medicine 158 (20): 2187–91. November 1998. doi:10.1001/archinte.158.20.2187. PMID 9818798.
- ↑ "Vitamin C in human health and disease is still a mystery? An overview". Nutrition Journal 2 (7): 7. August 2003. doi:10.1186/1475-2891-2-7. PMID 14498993. PMC 201008. http://www.nutritionj.com/content/pdf/1475-2891-2-7.pdf.
- ↑ "Ascorbic acid supplements and kidney stone incidence among men: a prospective study". JAMA Internal Medicine 173 (5): 386–8. March 2013. doi:10.1001/jamainternmed.2013.2296. PMID 23381591.
- ↑ "Vitamin C in Critically Ill Patients: An Updated Systematic Review and Meta-Analysis". Nutrients 13 (10): 3564. October 2021. doi:10.3390/nu13103564. PMID 34684565.
- ↑ "Vitamin C Intervention for Critical COVID-19: A Pragmatic Review of the Current Level of Evidence". Life 11 (11): 1166. November 2021. doi:10.3390/life11111166. PMID 34833042. Bibcode: 2021Life...11.1166H.
- ↑ "Overview of the possible role of vitamin C in management of COVID-19". Pharmacol Rep 72 (6): 1517–28. December 2020. doi:10.1007/s43440-020-00176-1. PMID 33113146.
- ↑ 84.0 84.1 "Dietary Guidelines for Indians". National Institute of Nutrition, India. 2011. http://ninindia.org/DietaryGuidelinesforNINwebsite.pdf.
- ↑ "Chapter 7: Vitamin C". Vitamin and Mineral Requirements in Human Nutrition (2nd ed.). Geneva: World Health Organization. 2005. ISBN 978-92-4-154612-6.
- ↑ "Commission Directive 2008/100/EC of 28 October 2008 amending Council Directive 90/496/EEC on nutrition labeling for foodstuffs as regards recommended daily allowances, energy conversion factors and definitions". The Commission of the European Communities. October 29, 2008. http://eur-lex.europa.eu/legal-content/en/TXT/?uri=CELEX%3A32008L0100.
- ↑ "Vitamin C". Natural Health Product Monograph. Health Canada. http://www.hc-sc.gc.ca/dhp-mps/prodnatur/applications/licen-prod/monograph/mono_vitamin_c-eng.php.
- ↑ 88.0 88.1 "Overview of Dietary Reference Intakes for Japanese". 2015. https://www.mhlw.go.jp/file/06-Seisakujouhou-10900000-Kenkoukyoku/Overview.pdf.
- ↑ 89.0 89.1 "Overview on Dietary Reference Values for the EU population as derived by the EFSA Panel on Dietetic Products, Nutrition and Allergies". 2017. https://www.efsa.europa.eu/sites/default/files/assets/DRV_Summary_tables_jan_17.pdf.
- ↑ "Association between vitamin C intake and lung cancer: a dose-response meta-analysis". Scientific Reports 4: 6161. 2014. doi:10.1038/srep06161. PMID 25145261. Bibcode: 2014NatSR...4E6161L.
- ↑ "TABLE 1: Nutrient Intakes from Food and Beverages". National Health and Nutrition Examination Survey: What We Eat in America, DHHS-USDA Dietary Survey Integration. Centers for Disease Control and Prevention, U.S. Department of Health & Human Services. https://www.ars.usda.gov/ARSUserFiles/80400530/pdf/1314/Table_1_NIN_GEN_13.pdf.
- ↑ "TABLE 37: Nutrient Intakes from Dietary Supplements". National Health and Nutrition Examination Survey: What We Eat in America, DHHS-USDA Dietary Survey Integration. Centers for Disease Control and Prevention, U.S. Department of Health & Human Services. https://www.ars.usda.gov/ARSUserFiles/80400530/pdf/1314/Table_37_SUP_GEN_13.pdf.
- ↑ "Tolerable Upper Intake Levels For Vitamins And Minerals". European Food Safety Authority. 2006. http://www.efsa.europa.eu/sites/default/files/efsa_rep/blobserver_assets/ndatolerableuil.pdf.
- ↑ "Federal Register May 27, 2016 Food Labeling: Revision of the Nutrition and Supplement Facts Labels. FR page 33982.". https://www.gpo.gov/fdsys/pkg/FR-2016-05-27/pdf/2016-11867.pdf.
- ↑ "Daily Value Reference of the Dietary Supplement Label Database (DSLD)". https://www.dsld.nlm.nih.gov/dsld/dailyvalue.jsp.
- ↑ REGULATION (EU) No 1169/2011 OF THE EUROPEAN PARLIAMENT AND OF THE COUNCIL Official Journal of the European Union. page 304/61. (2009).
- ↑ 97.0 97.1 "Vitamin C Content of Citrus from Conventional versus Organic Farming Systems". Acta Horticulturae 868 (868): 389–94. 2010. doi:10.17660/ActaHortic.2010.868.52. https://www.researchgate.net/publication/216361377. Retrieved May 5, 2018.
- ↑ "NDL/FNIC Food Composition Database Home Page". USDA Nutrient Data Laboratory, the Food and Nutrition Information Center and Information Systems Division of the National Agricultural Library.. https://fdc.nal.usda.gov/.
- ↑ 99.0 99.1 "Natural food-Fruit Vitamin C Content". The Natural Food Hub. http://www.naturalhub.com/natural_food_guide_fruit_vitamin_c.htm.
- ↑ 100.0 100.1 100.2 "USDA National Nutrient Database for Standard Reference Legacy: Vitamin C". 2018. https://www.nal.usda.gov/sites/www.nal.usda.gov/files/vitamin_c.pdf.
- ↑ "The nutritional composition of Australian aboriginal bushfoods. I". Food Technology in Australia 35 (6): 293–296. 1987.
- ↑ "Nutritional composition and vitamin C stability in stored camu-camu (Myrciaria dubia) pulp". Archivos Latinoamericanos de Nutricion 50 (4): 405–8. December 2000. PMID 11464674.
- ↑ "Chemical composition of acerola fruit (Malpighia punicifolia L.) at three stages of maturity". Food Chemistry 71 (2): 195–198. 2000. doi:10.1016/S0308-8146(00)00152-7.
- ↑ (in en) A Textbook of Foods, Nutrition & Dietetics. Sterling Publishers Pvt. Ltd. 2008. p. 72. ISBN 978-81-207-3714-3. https://books.google.com/books?id=tMNnaw3lN7oC&pg=PP82.
- ↑ (in en) Handbook of Fruits and Fruit Processing. John Wiley & Sons. 2012. ISBN 978-1-118-35263-2. https://books.google.com/books?id=1qwuBXeczzgC&pg=PT1734.
- ↑ "Vitamin C Content in Sea Buckthorn Berries (Hippophaë rhamnoides L. ssp . rhamnoides) and Related Products: A Kinetic Study on Storage Stability and the Determination of Processing Effects". Journal of Food Science 73 (9): C615–C620. 2008. doi:10.1111/j.1750-3841.2008.00957.x. PMID 19021790.
- ↑ "Comparing Milk: Human, Cow, Goat & Commercial Infant Formula". Washington State University. January 8, 2007. http://www.saanendoah.com/compare.html.
- ↑ "A model study on rate of degradation of L-ascorbic acid during processing using home-produced juice concentrates". International Journal of Food Sciences and Nutrition 46 (2): 107–15. May 1995. doi:10.3109/09637489509012538. PMID 7621082.
- ↑ "The losses of ascorbic acid during the large-scale cooking of green vegetables by different methods". The British Journal of Nutrition 4 (2–3): 95–100. 1950. doi:10.1079/BJN19500024. PMID 14801407.
- ↑ 110.0 110.1 "Safety (MSDS) data for ascorbic acid". Oxford University. October 9, 2005. http://physchem.ox.ac.uk/MSDS/AS/ascorbic_acid.html.
- ↑ 111.0 111.1 111.2 "Introduction". Vitamin C Fortification of Food Aid Commodities: Final Report.. National Academies Press (US). 1997. https://www.ncbi.nlm.nih.gov/books/NBK230149/. Retrieved January 3, 2024.
- ↑ "Liposomal-encapsulated Ascorbic Acid: Influence on Vitamin C Bioavailability and Capacity to Protect Against Ischemia-Reperfusion Injury". Nutrition and Metabolic Insights 9: 25–30. 2016. doi:10.4137/NMI.S39764. PMID 27375360.
- ↑ "Why fortify?". December 2023. https://www.ffinetwork.org/savelives.
- ↑ 114.0 114.1 "Map: Count of Nutrients In Fortification Standards". https://fortificationdata.org/map-number-of-nutrients/.
- ↑ "USAID's Bureau for Humanitarian Assistance website". November 21, 2023. https://www.usaid.gov/who-we-are/organization/bureaus/bureau-humanitarian-assistance.
- ↑ "Pretreatments to prevent darkening of fruits prior to canning or dehydrating". Utah State University. 2017. https://digitalcommons.usu.edu/cgi/viewcontent.cgi?article=2781&context=extension_curall.
- ↑ "Ingredients". The Federation of Bakers. https://www.fob.uk.com/about-the-bread-industry/how-bread-is-made/ingredients/.
- ↑ "Frequently Asked Questions | Why Food Additives". http://www.faia.org.uk/faq2_4.php.
- ↑ 119.0 119.1 119.2 119.3 119.4 UK Food Standards Agency: "Current EU approved additives and their E Numbers". http://www.food.gov.uk/safereating/chemsafe/additivesbranch/enumberlist.
- ↑ 120.0 120.1 120.2 US Food and Drug Administration:"Listing of Food Additives Status Part I". https://www.fda.gov/Food/FoodIngredientsPackaging/FoodAdditives/FoodAdditiveListings/ucm091048.htm.
- ↑ 121.0 121.1 121.2 121.3 Health Canada "List of Permitted Preservatives (Lists of Permitted Food Additives) - Government of Canada". November 27, 2006. https://www.canada.ca/en/health-canada/services/food-nutrition/food-safety/food-additives/lists-permitted/11-preservatives.html.
- ↑ 122.0 122.1 122.2 122.3 122.4 Australia New Zealand Food Standards Code"Standard 1.2.4 – Labeling of ingredients". September 8, 2011. http://www.comlaw.gov.au/Details/F2011C00827.
- ↑ "Listing of Food Additives Status Part II". https://www.fda.gov/Food/FoodIngredientsPackaging/FoodAdditives/ucm191033.htm#ftnT.
- ↑ 124.0 124.1 "SVCT1 and SVCT2: key proteins for vitamin C uptake". Amino Acids 34 (3): 347–55. April 2008. doi:10.1007/s00726-007-0555-7. PMID 17541511.
- ↑ "Glucose transporter isoforms GLUT1 and GLUT3 transport dehydroascorbic acid". The Journal of Biological Chemistry 272 (30): 18982–89. July 1997. doi:10.1074/jbc.272.30.18982. PMID 9228080.
- ↑ "Recycling of vitamin C from its oxidized forms by human endothelial cells". Biochimica et Biophysica Acta (BBA) - Molecular Cell Research 1640 (2–3): 153–61. May 2003. doi:10.1016/S0167-4889(03)00043-0. PMID 12729925.
- ↑ "Maturational loss of the vitamin C transporter in erythrocytes". Biochemical and Biophysical Research Communications 360 (1): 295–98. August 2007. doi:10.1016/j.bbrc.2007.06.072. PMID 17586466.
- ↑ 128.0 128.1 "Vitamin C: the known and the unknown and Goldilocks". Oral Diseases 22 (6): 463–93. September 2016. doi:10.1111/odi.12446. PMID 26808119.
- ↑ "Renal excretion of ascorbic acid: effect of age and sex". Journal of the American College of Nutrition 12 (5): 537–42. October 1993. doi:10.1080/07315724.1993.10718349. PMID 8263270.
- ↑ 130.0 130.1 "Vitamin C. Biosynthesis, recycling and degradation in mammals". The FEBS Journal 274 (1): 1–22. January 2007. doi:10.1111/j.1742-4658.2006.05607.x. PMID 17222174.
- ↑ "Testing Foods for Vitamin C (Ascorbic Acid)". British Nutrition Foundation. 2004. http://www.foodafactoflife.org.uk/attachments/ff2caf4a-75e5-4aa129132873.pdf.
- ↑ "Measuring the Vitamin C content of foods and fruit juices". Nuffield Foundation. November 24, 2011. http://www.nuffieldfoundation.org/practical-biology/measuring-vitamin-c-content-foods-and-fruit-juices.
- ↑ "Biosynthesis of vitamin C by yeast leads to increased stress resistance". PLOS ONE 2 (10): e1092. October 2007. doi:10.1371/journal.pone.0001092. PMID 17971855. Bibcode: 2007PLoSO...2.1092B.
- ↑ "The biosynthetic pathway of vitamin C in higher plants". Nature 393 (6683): 365–9. May 1998. doi:10.1038/30728. PMID 9620799. Bibcode: 1998Natur.393..365W.
- ↑ 135.0 135.1 135.2 "The Natural History of Ascorbic Acid in the Evolution of the Mammals and Primates and Its Significance for Present-Day Man Evolution of Mammals and Primates". Journal of Orthomolecular Psychiatry 1 (2): 82–89. 1972. http://orthomolecular.org/library/jom/1972/pdf/1972-v01n02%2603-p082.pdf. Retrieved December 31, 2023.
- ↑ "The hepatic glycogenoreticular system". Pathology & Oncology Research 7 (2): 107–10. 2001. doi:10.1007/BF03032575. PMID 11458272.
- ↑ 137.0 137.1 "Biosynthesis of L-Ascorbic Acid in Plants: New Pathways for an Old Antioxidant". Trends in Plant Science 9 (12): 573–577. 2004. doi:10.1016/j.tplants.2004.10.002. PMID 15564123. http://www.bmbq.uma.es/lbbv/index_archivos/pdf/Valpuesta%202004.pdf. Retrieved October 8, 2018.
- ↑ "Molecular basis for the deficiency in humans of gulonolactone oxidase, a key enzyme for ascorbic acid biosynthesis". The American Journal of Clinical Nutrition 54 (6 Suppl): 1203S–1208S. December 1991. doi:10.1093/ajcn/54.6.1203s. PMID 1962571.
- ↑ "Guinea pigs possess a highly mutated gene for L-gulono-gamma-lactone oxidase, the key enzyme for L-ascorbic acid biosynthesis missing in this species". The Journal of Biological Chemistry 267 (30): 21967–72. October 1992. doi:10.1016/S0021-9258(19)36707-9. PMID 1400507.
- ↑ "Random nucleotide substitutions in primate nonfunctional gene for L-gulono-gamma-lactone oxidase, the missing enzyme in L-ascorbic acid biosynthesis". Biochimica et Biophysica Acta (BBA) - General Subjects 1472 (1–2): 408–11. October 1999. doi:10.1016/S0304-4165(99)00123-3. PMID 10572964.
- ↑ "Plasma ascorbic acid concentrations in healthy dogs". Res. Vet. Sci. 71 (1): 33–5. August 2001. doi:10.1053/rvsc.2001.0481. PMID 11666145.
- ↑ "l-Ascorbic acid (vitamin C) supplementation to optimize health and reproduction in cattle". Vet Q 32 (3–4): 145–50. 2012. doi:10.1080/01652176.2012.734640. PMID 23078207.
- ↑ 143.0 143.1 143.2 Medicinal Natural Products: A Biosynthetic Approach (3rd ed.). John Wiley and Sons. 2009. p. 493. ISBN 978-0470741672.
- ↑ Fowler's Zoo and Wild Animal Medicine, Volume 8. Elsevier Health Sciences. 2014. p. 389. ISBN 9781455773992. https://books.google.com/books?id=llBcBAAAQBAJ&q=Caviidae+%22vitamin+C%22&pg=PA389. Retrieved June 2, 2016.
- ↑ "Variation of l-gulonolactone oxidase activity in placental mammals". Comparative Biochemistry and Physiology B 67 (2): 195–204. 1980. doi:10.1016/0305-0491(80)90131-5.
- ↑ "Progressive pseudogenization: vitamin C synthesis and its loss in bats". Molecular Biology and Evolution 28 (2): 1025–31. February 2011. doi:10.1093/molbev/msq286. PMID 21037206.
- ↑ "Recent loss of vitamin C biosynthesis ability in bats". PLOS ONE 6 (11): e27114. Nov 2011. doi:10.1371/journal.pone.0027114. PMID 22069493. Bibcode: 2011PLoSO...627114C.
- ↑ "Can passerines synthesize vitamin C?". The Auk 114 (3): 513–516. July 1997. doi:10.2307/4089257.
- ↑ 149.0 149.1 149.2 "The genetics of vitamin C loss in vertebrates". Current Genomics 12 (5): 371–378. August 2011. doi:10.2174/138920211796429736. PMID 22294879.
- ↑ Freshwater Fish Distribution. University of Chicago Press. 2008. p. 55. ISBN 978-0-226-04443-9. https://books.google.com/books?id=K-1Ygw6XwFQC&pg=PA55.
- ↑ "Nutritional characteristics of wild primate foods: do the diets of our closest living relatives have lessons for us?". Nutrition 15 (6): 488–98. June 1999. doi:10.1016/S0899-9007(99)00078-7. PMID 10378206. http://www.direct-ms.org/pdf/EvolutionPaleolithic/primaten.pdf.
- ↑ 152.0 152.1 "Evolution and the need for ascorbic acid". Proceedings of the National Academy of Sciences of the United States of America 67 (4): 1643–8. December 1970. doi:10.1073/pnas.67.4.1643. PMID 5275366. Bibcode: 1970PNAS...67.1643P.
- ↑ Parrott, Terri (October 2022). "Nutritional Diseases of Nonhuman Primates". https://www.msdvetmanual.com/exotic-and-laboratory-animals/nonhuman-primates/nutritional-diseases-of-nonhuman-primates.
- ↑ "Inactivation dates of the human and guinea pig vitamin C genes". Genetica 139 (2): 199–207. February 2011. doi:10.1007/s10709-010-9537-x. PMID 21140195.
- ↑ "Conserved or lost: molecular evolution of the key gene GULO in vertebrate vitamin C biosynthesis". Biochemical Genetics 51 (5–6): 413–25. June 2013. doi:10.1007/s10528-013-9574-0. PMID 23404229.
- ↑ "Identification and analysis of unitary pseudogenes: historic and contemporary gene losses in humans and other primates". Genome Biology 11 (3): R26. 2010. doi:10.1186/gb-2010-11-3-r26. PMID 20210993.
- ↑ "Isolation and sequence analysis of a complementary DNA encoding rat liver L-gulono-gamma-lactone oxidase, a key enzyme for L-ascorbic acid biosynthesis". The Journal of Biological Chemistry 263 (4): 1619–21. February 1988. doi:10.1016/S0021-9258(19)77923-X. PMID 3338984.
- ↑ 158.0 158.1 "Vitamin C biosynthesis in prosimians: evidence for the anthropoid affinity of Tarsius". American Journal of Physical Anthropology 73 (1): 65–70. 1987. doi:10.1002/ajpa.1330730106. PMID 3113259.
- ↑ "Primate phylogeny, evolutionary rate variations, and divergence times: a contribution from the nuclear gene IRBP". American Journal of Physical Anthropology 124 (1): 01–16. 2004. doi:10.1002/ajpa.10322. PMID 15085543.
- ↑ "Toward a phylogenetic classification of Primates based on DNA evidence complemented by fossil evidence". Molecular Phylogenetics and Evolution 9 (3): 585–98. June 1998. doi:10.1006/mpev.1998.0495. PMID 9668008.
- ↑ "Phylogeny and Evolution of Selected Primates as Determined by Sequences of the ε-Globin Locus and 5′ Flanking Regions". International Journal of Primatology 18 (2): 261–295. April 1997. doi:10.1023/A:1026328804319.
- ↑ "Similar functions of uric acid and ascorbate in man?". Nature 228 (5274): 868. 1970. doi:10.1038/228868a0. PMID 5477017. Bibcode: 1970Natur.228..868P.
- ↑ "l-Galactono-gamma-lactone dehydrogenase from Arabidopsis thaliana, a flavoprotein involved in vitamin C biosynthesis". The FEBS Journal 275 (4): 713–26. February 2008. doi:10.1111/j.1742-4658.2007.06233.x. PMID 18190525.
- ↑ "Feedback inhibition of spinach L-galactose dehydrogenase by L-ascorbate". Plant & Cell Physiology 45 (9): 1271–9. September 2004. doi:10.1093/pcp/pch152. PMID 15509850.
- ↑ "Ascorbate efflux as a new strategy for iron reduction and transport in plants". The Journal of Biological Chemistry 289 (5): 2515–25. January 2014. doi:10.1074/jbc.M113.514828. PMID 24347170.
- ↑ 166.0 166.1 166.2 "L-ascorbic Acid: a multifunctional molecule supporting plant growth and development". Scientifica 2013: 1–24. 2013. doi:10.1155/2013/795964. PMID 24278786.
- ↑ 167.0 167.1 "Genetic Control of Ascorbic Acid Biosynthesis and Recycling in Horticultural Crops". Frontiers in Chemistry 5: 50. 2017. doi:10.3389/fchem.2017.00050. PMID 28744455. Bibcode: 2017FrCh....5...50M.
- ↑ "The regulation of ascorbate biosynthesis". Current Opinion in Plant Biology 33: 15–22. October 2016. doi:10.1016/j.pbi.2016.04.010. PMID 27179323.
- ↑ "One hundred years of vitamins-a success story of the natural sciences". Angewandte Chemie 51 (52): 12960–12990. December 2012. doi:10.1002/anie.201205886. PMID 23208776.
- ↑ "The production of vitamin C". Competition Commission. 2001. http://www.competition-commission.org.uk/rep_pub/reports/2001/fulltext/456a4.2.pdf.
- ↑ "One-Step Biosynthesis of Vitamin C in Saccharomyces cerevisiae". Front Microbiol 12: 643472. 2021. doi:10.3389/fmicb.2021.643472. PMID 33717042.
- ↑ "Metabolic engineering of Escherichia coli for direct production of vitamin C from D-glucose". Biotechnol Biofuels Bioprod 15 (1): 86. August 2022. doi:10.1186/s13068-022-02184-0. PMID 35996146.
- ↑ "Vantage Market Research: Global Vitamin C Market Size & Share to Surpass $1.8 Bn by 2028". Globe Newswire (Press release). November 8, 2022. Archived from the original on December 21, 2023. Retrieved December 21, 2023.
- ↑ "Vitamin C price trend and forecast". September 2023. https://www.chemanalyst.com/Pricing-data/vitamin-c-1258.
- ↑ "Scott and Scurvy". March 7, 2010. http://idlewords.com/2010/03/scott_and_scurvy.htm.
- ↑ "Infantile scurvy: a historical perspective". Pediatrics 108 (4): E76. October 2001. doi:10.1542/peds.108.4.e76. PMID 11581484. http://pediatrics.aappublications.org/content/108/4/e76.full. "As they sailed farther up the east coast of Africa, they met local traders, who traded them fresh oranges. Within six days of eating the oranges, da Gama's crew recovered fully".
- ↑ "Santa Helena, A Forgotten Portuguese Discovery". Estudos Em Homenagem a Luis Antonio de Oliveira Ramos: 623–631. 2004. http://ler.letras.up.pt/uploads/ficheiros/4999.pdf. "On returning, Lopes' ship had left him on St Helena, where with admirable sagacity and industry he planted vegetables and nurseries with which passing ships were marvelously sustained. [...] There were 'wild groves' of oranges, lemons and other fruits that ripened all the year round, large pomegranates and figs.".
- ↑ The Surgions Mate. London, England: Edward Griffin. 1617. p. 89. https://archive.org/stream/surgionsmateortr00wood#page/89/mode/1up. "Succus Limonum, or juice of Lemons ... [is] the most precious help that ever was discovered against the Scurvy[;] to be drunk at all times; ..."
- ↑ "Observation on Naval Hygiene and Scurvy, more particularly as the later appeared during the Polar Voyage". British and Foreign Medico-chirurgical Review: Or, Quarterly Journal of Practical Medicine and Surgery 22: 295–305. 1858. https://books.google.com/books?id=7VJYAAAAMAAJ&pg=PA295.
- ↑ (in Latin) Observationes circa scorbutum. Leiden (Lugdunum Batavorum), Netherlands: Conrad Wishof. 1734. p. 16. https://books.google.com/books?id=bj8_AAAAcAAJ&pg=PA16. "... sed ex nostra causa optime explicatur, que est absentia, carentia & abstinentia a vegetabilibus recentibus, ... ( ... but [this misfortune] is explained very well by our [supposed] cause, which is the absence of, lack of, and abstinence from fresh vegetables, ..."
- ↑ "Captain Cook and the Scourge of Scurvy". British History in depth. BBC. February 17, 2011. https://www.bbc.co.uk/history/british/empire_seapower/captaincook_scurvy_01.shtml.
- ↑ Preserving the self in the south seas, 1680–1840. University of Chicago Press. 2001. p. 117. ISBN 978-0-226-46849-5. https://books.google.com/books?id=hSoj1DR4ZSMC.
- ↑ Trick or Treatment: The Undeniable Facts about Alternative Medicine. WW Norton & Company. 2008. pp. 15–18. ISBN 978-0-393-06661-6.
- ↑ The journals of Captain Cook. Harmondsworth [Eng.]: Penguin. 1999. ISBN 978-0-14-043647-1. https://archive.org/details/journalsofcaptai00jame.
- ↑ "Copley Medal, Past winners". https://royalsociety.org/grants-schemes-awards/awards/copley-medal/.
- ↑ "Cook's Voyages 1768–1780". Navy and the Nation: The Influence of the Navy on Modern Australia. Allen & Unwin Academic. 2006. p. 74. ISBN 978-1-74114-200-6. https://books.google.com/books?id=BGs6__kbqKIC&pg=PA74.
- ↑ "Arctic indigenous peoples experience the nutrition transition with changing dietary patterns and obesity". The Journal of Nutrition 134 (6): 1447–53. June 2004. doi:10.1093/jn/134.6.1447. PMID 15173410.
- ↑ "Lost in the Twentieth Century". Annual Review of Biochemistry 32 (1): 1–14. June 1963. doi:10.1146/annurev.bi.32.070163.000245. PMID 14140702.
- ↑ "Edmund Langley Hirst". Advances in Carbohydrate Chemistry and Biochemistry Volume 35. 35. 1978. pp. 1–29. doi:10.1016/S0065-2318(08)60217-6. ISBN 9780120072354.
- ↑ "Redoxon trademark information by Hoffman-la Roche, Inc. (1934)". http://www.trademarkia.com/redoxon-71350953.html.
- ↑ "Industrial fermentation of Vitamin C". Industrial Biotechnology of Vitamins, Biopigments, and Antioxidants. Wiley-VCH Verlag GmbH & Co. KGaA.. 2016. p. 161. ISBN 9783527337347. https://books.google.com/books?id=WgamCgAAQBAJ&pg=PA161.
- ↑ "[Axel Holst and Theodor Frolich--pioneers in the combat of scurvy]" (in no). Tidsskrift for den Norske Laegeforening 122 (17): 1686–7. June 2002. PMID 12555613.
- ↑ "Vitamine--vitamin. The early years of discovery". Clinical Chemistry 43 (4): 680–5. April 1997. doi:10.1093/clinchem/43.4.680. PMID 9105273.
- ↑ 194.0 194.1 "The chemical nature of vitamin C". The Biochemical Journal 26 (3): 865–70. 1932. doi:10.1126/science.75.1944.357-a. PMID 16744896. Bibcode: 1932Sci....75..357K.
- ↑ "[Albert Szent-Györgyi--biography of a free genius]" (in hu). Orvosi Hetilap 143 (12): 611–4. March 2002. PMID 11963399.
- ↑ "[Eventful life of a scientist. 80th birthday of Nobel prize winner Albert Szent-Györgyi]" (in de). Munchener Medizinische Wochenschrift 115 (51): 2324–6. December 1973. PMID 4589872.
- ↑ "[2 interesting early articles by Albert Szent-Györgyi]" (in hu). Orvosi Hetilap 115 (52): 3118–9. December 1974. PMID 4612454.
- ↑ 198.0 198.1 "The Albert Szent-Gyorgyi Papers: Szeged, 1931-1947: Vitamin C, Muscles, and WWII". Profiles in Science. United States National Library of Medicine. http://profiles.nlm.nih.gov/WG/Views/Exhibit/narrative/szeged.html.
- ↑ "Scurvy". Online Entymology Dictionary. https://www.etymonline.com/word/scurvy.
- ↑ "The synthesis of L-ascorbic acid in the rat from D-glucuronolactone and L-gulonolactone" (PDF). The Journal of Biological Chemistry 223 (2): 897–905. December 1956. doi:10.1016/S0021-9258(18)65088-4. PMID 13385237. https://www.jbc.org/article/S0021-9258(18)65088-4/pdf. Retrieved December 3, 2022.
- ↑ "Missing step in guinea pigs required for the biosynthesis of L-ascorbic acid". Science 124 (3232): 1148–9. December 1956. doi:10.1126/science.124.3232.1148-a. PMID 13380431. Bibcode: 1956Sci...124.1148B.
- ↑ "Ascorbic acid: biologic functions and relation to cancer". Journal of the National Cancer Institute 83 (8): 547–50. April 1991. doi:10.1093/jnci/83.8.547. PMID 1672383. https://zenodo.org/record/1234351. Retrieved March 18, 2020.
- ↑ 203.0 203.1 Saul, Andrew. "Orthomolecular Medicine Hall of fame - Irwin Stone, Ph.D.". http://www.orthomolecular.org/history/index.shtml.
- ↑ "Erythrocyte Glut1 triggers dehydroascorbic acid uptake in mammals unable to synthesize vitamin C". Cell 132 (6): 1039–48. March 2008. doi:10.1016/j.cell.2008.01.042. PMID 18358815.
- ↑ "Vitamin C: update on physiology and pharmacology". British Journal of Pharmacology 157 (7): 1097–110. August 2009. doi:10.1111/j.1476-5381.2009.00282.x. PMID 19508394.
- ↑ "Supplemental ascorbate in the supportive treatment of cancer: Prolongation of survival times in terminal human cancer". Proceedings of the National Academy of Sciences of the United States of America 73 (10): 3685–9. October 1976. doi:10.1073/pnas.73.10.3685. PMID 1068480. Bibcode: 1976PNAS...73.3685C.
- ↑ Boseley, Sarah (September 12, 2008). "Fall of the vitamin doctor: Matthias Rath drops libel action". https://www.theguardian.com/world/2008/sep/12/matthiasrath.aids2.
- ↑ Colquhoun, David (August 15, 2007). "The age of endarkenment | Science | guardian.co.uk". Guardian. https://www.theguardian.com/science/2007/aug/15/endarkenment.
- ↑ "The Dark Side of Linus Pauling's Legacy". September 14, 2014. https://www.quackwatch.org/01QuackeryRelatedTopics/pauling.html.
- ↑ "Review of high-dose intravenous vitamin C as an anticancer agent". Asia-Pacific Journal of Clinical Oncology 10 (1): 22–37. March 2014. doi:10.1111/ajco.12173. PMID 24571058.
- ↑ "Let the chemical games begin!". Swiss Info. Swiss Broadcasting Corporation. February 17, 2011. http://www.swissinfo.ch/eng/Specials/International_year_of_chemistry/Background/Let_the_chemical_games_begin!.html?cid=29513206.
- ↑ British Pharmacopoeia Commission Secretariat (2009). "Index, BP 2009". http://www.pharmacopoeia.co.uk/pdf/2009_index.pdf.
- ↑ "Japanese Pharmacopoeia, Fifteenth Edition". 2006. http://jpdb.nihs.go.jp/jp15e/JP15.pdf.
External links
- "Vitamin C Fact Sheet". https://ods.od.nih.gov/factsheets/Vitaminc-HealthProfessional/.
- "Scott and Scurvy". March 6, 2010. https://idlewords.com/2010/03/scott_and_scurvy.htm.
![]() | Original source: https://en.wikipedia.org/wiki/Vitamin C.
Read more |